ABSTRACT
Aluminosilicate are often produced from kaolin with a kaolin bond in the formulation intended to increase the thermal strength of the product. Because kaolin formulations do not sinter well, investigation of methods for increasing the density of kaolin is highly relevant. The density can be increased by increasing the degree of dispersion. An additive which improves sinterability would be especially effective. Bentonite with its high plasticity and reactive surface meets these requirements to a certain extent. Four formulations of the porcelain tableware were prepared in different percentage from 0 to 15 wt.% of calcic bentonite. The mass loss and shrinkage of different formulations were measured by using the following thermal analysis such as: Differential thermal analysis (DTA), thermal gravimetric analysis (TGA) and dilatometric analysis. Its density, water absorption, total porosity and the flexural strength were also measured at different temperatures between 1250 and 1400°C. It was found that the bentonite added to the formulation increases the strength of the product and reduces the total porosity to 3.35% by increasing the degree of dispersion during the grinding of the components, thereby improving the sintering process of the formulation. On one hand, the weight loss, shrinkage and sintering time were decreased. On the other hand, the thermal resistance and flexural strength were increased at 1380°C for 10 wt.% of bentonite content.
Key words: Firing, suspensions, total porosity, thermal properties, flexural strength, bentonite.
Formulations of traditional porcelain usually involve 25 wt.% of plastic component, 25 wt.% silica and 50 wt.% feldspar (generally sodium feldspar) for soft porcelain and 50 wt.% of clay, 25 wt.% silica and 25 wt.% feldspar (generally potassium feldspar) for hard porcelain (Jouene, 2001; Alessandro, 2007). Bentonite is a natural mixture of minerals, it is characterized by a high capacity adsorption, ion exchange and swelling. There are two types of bentonite: sodic and calcic. In order to realize the sodium bentonites, the calcic bentonites are treated by sodium hydroxide because they have the highest capacity of swelling. Bentonite is used extensively in various branches of industry such as paper, food, pharmaceutical and petrochemical industries, etc. (Savic et al., 2014a). Also, bentonite has been applied in environmental preservation when removing heavy metal ions from wastewater (Savic et al., 2012; Savic et al., 2014b).
The obtained results by Rodriguez and Torrecillas (2002); García and Aguilar-Elguézaba (2009); Andreola and Siligardi (2009) of the use of the bentonite in the different formulations of ceramic pastes for wall tiles, bricks, ceramic tiles and porcelain stoneware are:
(1) Processes during firing are different from those of the classical formulation of kaolin.
(2)Better control of the dimensional characteristics of pieces during firing has been achieved.
(3)Technological parameters pieces formulated with bentonite (shrinkage, modulus of rupture, apparent density, water absorption, expansion by humidity) satisfy the industrial requirements for the production of wall tiles and they have an important function: to confer plasticity in the green state and, during firing, undergo some structural modifications which furnish the main oxides to form some important phases such as mullite (3Al2O3·2SiO2).
Substitution of clay by the bentonite treated thermally present economic advantages, increases the strength of the product and reduces the total porosity. The obtained porcelain tiles mixtures represent the plastic components that affect the rheological behavior, that is, it increases the viscosity and thixotropy. The elasticity and resistance to rupture were increased by using the bentonite in the ceramic formulations with 10 wt.%.
The held exchangeable cations on the layers’ surface were linked together. The intensity of these bonds depends on the valence of the cations (Wakim, 2005). The more compensating cations are small and weakly charged, the more the clay swelling is important. Therefore, in this study, the bentonite swelling is low because the exchangeable cations are Ca+2. As a result, the specific surface is low. The reactive surface of bentonites takes the form of lateral faulted bonds and external basal-plane boundaries with a very low negative charge. For minerals of the kaolinite group the reactive surface is represented only by the edge regions of the crystals at the rupture sites on the bonds Si-O-Si and OH-AlIV-OH when the fringe atoms of oxygen or hydroxyl are incompletely sutured with silicon and aluminum respectively.
The aim of this paper provides the performance of the nature of interactions between raw materials and calcic bentonite for producing the material porcelain tableware. According to the previous studies, the resulted materials using the calcic bentonite are not enameled. The obtained formulations are characterized in terms of: physical, mechanical and thermal analysis.
Location of raw materials
The different formulations of porcelain tableware (0, 5, 10 and 15 wt.% of bentonite) were composed of kaolin from Djebel Debbagh, Guelma (Algeria) used in formulations tableware. The quartz comes from the Boussaâda area, the Feldspar from the Bouira area all in Algeria; and the bentonite was calcined in a continuous roller kiln (heating to 973°K with a heating rate of 5°Ks-1 and maintained at this temperature for 3m). To this is added a sodium tripoliphosphate (Na2P3O10, 6H2O) as deflocculant (0.3 wt.%) to improve the dispersion of the slurries.
Raw materials characterization
Mineralogical analyses were carried out with a diffractometer (Philips PW 1710, France) in step scan mode using Ni-filtered CuKa radiation (1.5406 Å); wide-angle X-ray spectra were recorded with a D 500. Elementary chemical analyses were performed by ICP (Induced Coupled Plasma) references Iris, marketed by Thermo Jorrell (Cheshire, England).
Samples preparation
The standard composition of triaxial porcelain, defined as P0 (0 wt.% of bentonite), was the formulation reference. Three formulations, with increasing amounts of bentonite (with respect to P0), designated from P1 to P3 (the number refers to the wt.% of bentonite in the batch), were produced in laboratory conditions via pouring and sintering. Table 1 summarizes the batch formulations and the chemical compositions of the porcelains (in wt.%). Bentonite was used in the three batches replacing feldspar that is normally used in traditional compositions. The main observations show the role of the bentonite as a fluxing agent.
Various formulations were dry-milled in a ball mill for 4 h and subsequently wet-milled in a ball mill for 40 m. In general the size of quartz and feldspar reached a value of (45 and 53 μm) respectively, to increase the strength and verification of porcelain material (Bragança and Bergmann, 2004). The resulting slurries were passed through a 63 μm sieve to calculate the residue on sieve. Values were between 5 and 7% depending on production requirements (Rodríguez and Torrecillas, 2002). The mixtures sunk in plaster rectangular molds were dried at room temperature for 48 h and at 105°C for 24 h. The samples were fritted at the temperatures of: 1250, 1300, 1380 and 1400°C (heating and cooling rates 5°/min and 1 h soaking at the highest temperature). The starting temperatures were chosen based on the respective liquidus temperatures of the SiO2–Al2O3–Na2O and SiO2–Al2O3–K2O systems.
Characterization of samples
Differential thermal analysis (DTA, Netzsch, STA 409) and thermal gravimetric analysis (TGA, Netzsch, STA 409) were carried out using standard procedure with alumina as reference, the analysis results of dilatometric analysis were measured in the apparatus (NETZSCH, 402C heating rate 5°C/min). The flexural strength was performed using equipment of a mark (Zwick / Roell) (Reference Z2.5KN), 0.5 mm/min displacement.
To characterize the fired specimens, water absorption was measured according to the ISO-standard 10545-3, 1995; weight gain of dried bulk samples after immersion into boiling water for 2 h, cooling for 3 h and sweeping of their surfaces with a wet towel.
True density (DT) was determined by the densimeter Candlot- Le Chatelier and apparent density (DA) by Archimedes method. The total porosity (P %) was then calculated using Equation (1). 80 parallelepiped bars (3 × 4 × 40 mm) were used for flexural tests (three point bending)
Raw materials and porcelain formulations
XRD analyses were carried out to determine the mineralogical compositions. The results show in the kaolin the presence of kaolinite (Al2 Si2O5 (OH)4) as the main phase and halloysite (Si2 Al2O5 (OH)4 nH2O) as a secondary phase. Feldspar is composed of feldspar potassic (KAlSi3O3) and anorthite (Si2O8CaAl2). Quartz is composed of quartz (SiO3) and traces of Ca, Mg and Al. The bentonite spectra indicate the presence of quartz, feldspar, cristobalite and carbonate (calcite) and it has a high proportion of montmorillonite.
Table 2 shows the chemical analyses of the raw materials. These results deduced from the elementary chemical measures analysis by ICP, showed that the kaolin contains small amounts of impurities such as Fe2O3, K2O, CaO and MnO; this is consistent with the white clay kaolin. The proportion of SiO2 in the feldspar more than 50 wt.% can contribute the formation of the liquid phase. The percentage of SiO2 in the quartz from Boussaâda exceeds 80 wt.% according to García and Aguilar-Elguézaba (2009) and the results obtained of raw materials used are alumino-silicate materials. Besides, it is important to note the iron oxide content of the samples for their use into porcelain tableware: the bentonites having a Fe2O3 > 5% will not be used to avoid the black core and changes on colorimetric properties of the ceramic porcelain after firing. The bentonite contains Fe2O3<5% and more MgO and slightly less Al2O3 compared to other raw materials.
Vitrification behavior
TGA and DTA of the four porcelain samples are shown in Figures 1 to 4. Major endothermic peaks at 122, 111.4, 122.1 and 113.1°C for 0, 5, 10 and 15 wt.% bentonite, respectively, were attributed to the elimination of water of clay minerals. Mass loss associated with these peaks was about 13.88, 13.36, 12.22 and 13.78% for 0, 5, 10 and 15 wt.% bentonite, respectively. Endothermic peaks at 526.1, 519.3, 538.1 and 515.1°C for 0, 5, 10 and 15 wt.% bentonite, respectively, were assigned to the dehydroxylation of the hydroxyl groups in the kaolin to form metakaolin (A12O3, 2SiO2) (Schneider et al., 1994) and dehydroxylation of montmorillonite (Celik, 2010). Mass loss associated with these peaks was 3.78, 3.67, 3.70 and 3.58% for 0, 5, 10 and 15 wt.% bentonite, respectively. Knowing that the bentonite stores some amount of water between its layers (Alessandro, 2007; García and Aguilar-Elguézaba, 2009), high quantity of water evaporated after a heat treatment of porcelains mixtures P1, P2 and P3.
Exothermic peaks appeared at 1004.70, 1003.70, 996.70 and 1008.61°C for 0, 5, 10 and 15 wt.% bentonite, respectively. Mass losses associated with these peaks were about 1.10, 0.87, 0.77 and 0.81% for 0, 5, 10 and15 wt.% bentonite, respectively. The bentonite added to the formulation increases the strength of the product by increasing the degree of dispersion during the grinding of the components, improving the sintering process of the formulation (the firing temperature of the formulations 0, 5, 10 wt.% of bentonite was: 1250, 1300, 1380°C. For the formulation 15 wt.% of bentonite was undetermined).
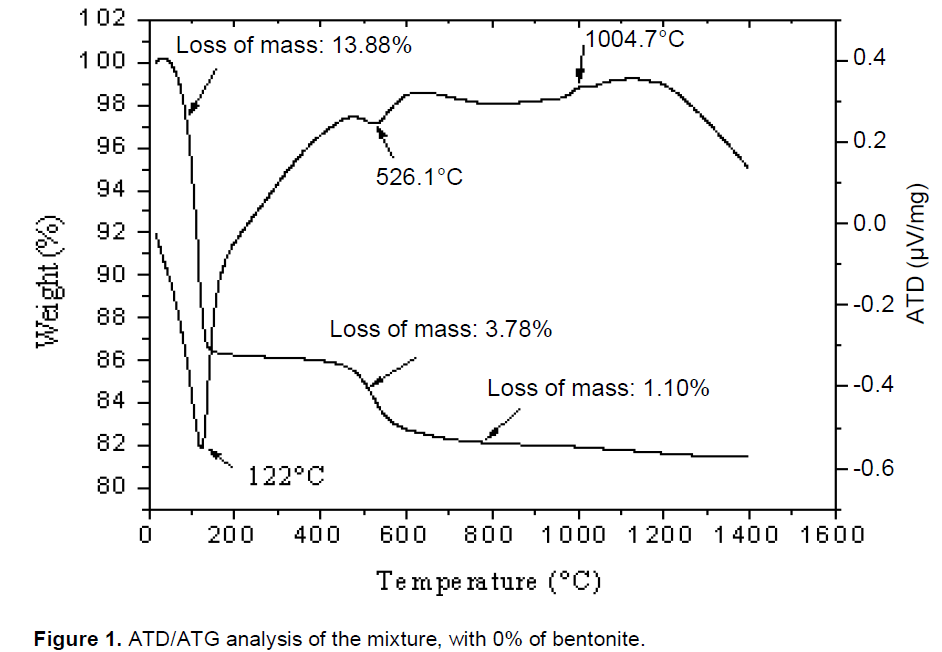
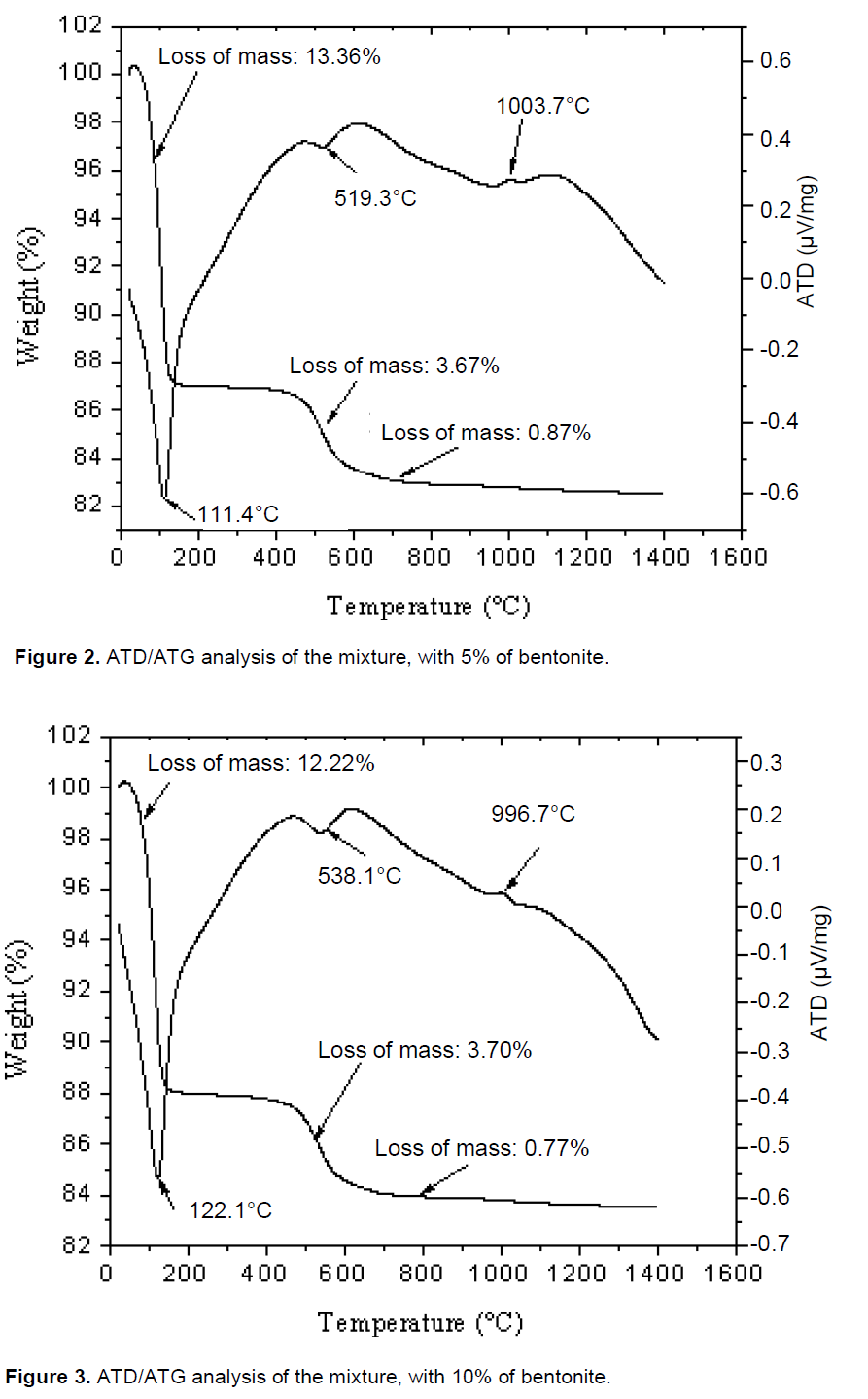
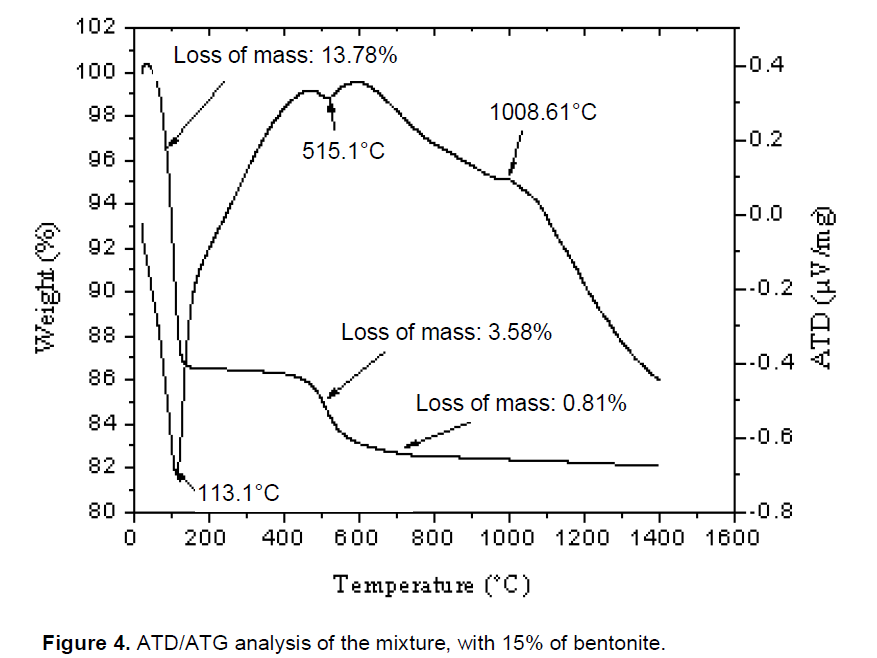
The TDA analysis showed a considerable liquid phase was formed, that has contributed to the fusion of bentonite (García and Aguilar-Elguézaba, 2009). It is considered that these changes are consistent with a mechanism of liquid phase sintering. This mechanism concerns the dissolution of the solids in the liquid phase and growth in the crystalline phases demonstrated by (Kingery et al., 1976; Celik, 2010). The closed pores are generated by the gas trapped and micro pores are generated by the process of crystallization (Karamanovand Ferrari, 2006). The ATG experimental analysis showed that the total mass loss mixtures of 0, 5, 10 and 15 wt.% of bentonite were: 18.76, 17.90, 16.69 and 18.17% respectively, and the lower value correspond to the mixture of 10 wt.% of bentonite.
After the high temperature treatment of bentonite, these polycationic species are transformed after dehydration and dehydroxylation into rigid pillars, linked permanently to the silicate layers. This process "pillar" leads to the formation of two-dimensional micro and/or mesoporous materials, known as pillared clays which are characterized by a good thermal stability. The thermal analysis results showed that the choice of raw materials percentages and development processes in thermaltreatments should be imposed to a weight of 10 wt.% in bentonite.
Values of maximum density, water absorption, total porosity and flexural strength of formulations for 0, 5, 10 and 15 wt.% of bentonite are: (2.48, 2.55, 2.59 and 2.68 g/cm3), (0.16, 0.09, 0.03 and 0.56%), (5.42, 3.72, 3.35 and 6.23%) and (90.71, 120.88, 135.07, and 36.43 N/mm2 and represented in Figure 5. The density for the porcelain of 10 wt.% bentonite was 2.59 g/cm3 at 1380°C, at this temperature, porcelain had water absorption value of 0.03% and total porosity of 3.35%.
Water absorption and porosity reduces with increasing firing temperature. This is due to the increasing formation of glass phase. The liquid phase aids in sintering, thereby increasing consolidation at higher temperatures. The firing temperature that gave maximum strength was approximately the lowest value of water absorption. It may be noted that maximum strength and maximum density were obtained from the same firing temperature. The highest flexural strength (that corresponds to the highest optimal density) was 135.07 N/mm2 for 10 wt.% of bentonite (which corresponds to the firing temperature 1380°C). For percentages (0, 5 and 15 wt.% of bentonite) the flexural strength was: 90.71 (1250°C), 120.88 (1300°C) and 36.43 N/mm2 (1400°C), respectively. Density is a property that has a strong influence on the mechanical strength. In general, the higher the density, more strength increases. The increased amount of liquid phase at higher temperature certainly affects negatively the mechanical strength. Generally, in ceramic compositions containing clays and feldspars, increased temperatures result in higher mullite formation, thereby improving mechanical properties.
Many studies have described the influence of the sum Fe2O3 + TiO2 from which the chemical composition is rich in the bentonite and favors the process of sintering of the ceramic matrix and the formation of a mullite composition of 10 wt.% bentonite (Kausik and Kumar, 2004; Kamseu et al., 2007; Andreola and Siligardi, 2009). Ti4+ and Fe3+ play important role by either substituting Al3+ or by their integration into the structural interstices of the matrix.
Increasing the amount of bentonite increases the formation of more of the liquid phase, density, the flexural strength; and decreases the porosity and water absorption. The dimensional changes observed after firing of the mixtures of porcelain P0, P1, P2 and P3 are given in Figure 6. Slight shrinkage surroundings between 33.62 and 112°C for percentages 0, 5, 10 and 15 wt.% of bentonite is due to the elimination of water of clay minerals. The maximum expansion rate between 112 and 600°C was 593.75°C; its percentage corresponds to 15 wt.% of bentonite. There was a small expansion of percentage mixtures 0, 5, 10 and 15 wt.% of bentonite between 500 and 600°C due to the α→β-quartz transformation (Celik, 2010). An initial shrinkage started at around 542.42, 555.75, 542.42 and 593.73°C of percentages 0, 5, 10 and 15 wt.% of bentonite respectively, due to the formation of metakaolinite. A second shrinkage from 808.30, 814.08, 865.97 and 804.84°C was attributed to sintering and formation of vitreous phases (Celik, 2010).
The maximum shrinkage rate between 990.22 and 1400°C was obtained at 998.59°C for the composition 15 wt.% bentonite. When the temperature reached 1034.20°C, the rate of shrinkage was approximately low -1.24% that corresponds to the composition of 10 wt.% of bentonite between the high temperature and room temperature. In particular the last step between 990 and 1400°C is an advantage in the use of bentonite. In the industrial process, the dimensional change should be minimal (García and Aguilar-Elguézaba, 2009; Dondi et al., 2014). The smallest shrinkage was due to the percentage 10 wt.% of bentonite.
Based on the results of the present study, the following conclusions can be drawn: the incorporation of small quantities of bentonite in raw materials represents a great potential in industrial porcelain. The bentonite occupies an important role in the mechanism of various steps of fire. The influence of bentonite increases the thermal resistance, densification and improves thermal firing cycle of the porcelain tableware. Densification was perfect and the firing cycle was short compared to the porcelain without bentonite. The appropriate percentage of bentonite is 10 wt.%.
The authors have not declared any conflict of interest.
REFERENCES
Andreola F, Siligardi C (2009). Rheological behavior and mechanical proprieties of porcelain stoneware bodies containing Italian clay added with bentonite. Ceramics Int. 35(3):1159-1164.
Crossref |
|
Alessandro F (2007). Thermal behavior of the raw materials forming porcelain stoneware mixtures by combined optical and in situ X-ray dilatometry. Am. Ceramic Soc. 90(4):1222-1231. |
|
Bragança SR, Bergmann CP (2004). Traditional and glass powder porcelain: technical and microstructure analysis. J. Eur. Ceramic Soc. 24(8):2383-2388.
Crossref |
|
Celik H (2010). Technological characterization and industrial application of two Turkish clays for the ceramic industry. Appl. Clay Sci. 50(2):245-254. |
|
Rodríguez DL, Torrecillas R (2002). Ceramic clays: a review of the different types, meanings and applications. Bull. Spanish Soc. Ceramics Glass 41(5):459-470.
Crossref |
|
Jouene CA (2001) Treaty of Ceramic Materials and Minerals. Editions Septima, Paris, pp. 657.
Crossref |
|
García A, Aguilar-Elguézaba A (2009). Use of thermally treated bentonitic clay in the formulation of ceramic tiles. Appl. Clay Sci. 46(3):271-276.
Crossref |
|
Karamanov A, Ferrari A (2006). The effect of fired scrap addition on the sintering behavior of hard porcelain. Ceramics Int. 32(7):727-732.
Crossref |
|
Kingery WD, Bowen HK, Uhlmann DR (1976). Introduction to Ceramics 2nd Edition, Wiley-Interscience, New York, P. 1008.
Crossref |
|
Kausik D, Kumar D (2004) Effect of substitution of fly ash for quartz in triaxial kaolin-quartz-feldspar system. J. Eur. Ceramic Soc. 24(10-11):3169-3175.
Crossref |
|
Dondi M, Raimondo M, Zanelli C (2014). Clays and bodies for ceramic tiles: Reappraisal and technological classification. Appl. Clay Sci. 96:91-109.
Crossref |
|
Kamseu E, Leonelli C, Boccaccini DN, Veronesi P, Miselli P, Giancarlo Pellacani, Chinje Melo U (2007). Characterisation of porcelain compositions using two china clays from Cameroon. Ceramics Int. 33(5):851-857.
Crossref |
|
Schneider H, Okada K, Pask J (1994). In Mullite and Mullite Ceramics. Wiley, New York, P. 106.
Crossref |
|
Savic IM, Stojiljkovic ST, Savic IM, Dragoljub GG (2014a). Industrial application of clays and clay minerals, In: Clays and Clay Minerals: Geological Origin, Mechanical Properties and Industrial Applications, Editors: Liam R. Wesley, Nova Science Publishers, Inc., USA. pp. 379-402. ISBN: 978-1-63117-779-8.
Crossref |
|
Savic IM, Savic IM, Stojiljkovic ST, Dragoljub GG (2014b). Modeling and optimization of energy-efficient procedures for removing lead(II) and zinc(II) ions from aqueous solutions using the central composite design. Energy 77(c):66-72.
Crossref |
|
Savic Ivana M, Stojiljkovic Stanisa T, Savic Ivan M, Sreten Stojanovic B, Karl Moder (2012). Modeling and optimization of iron(III) adsorption from water using bentonite clay: comparation of central composite design and artificial neural network, Chemical Engineering & Technology, 35(11):2007-2014.
Crossref |
|
Wakim J (2005). Influence of aqueous solutions on the mechanical behavior of argillaceous rocks. Thesis doctoral of higher national School of Mines, Paris.
Crossref |
|