The shell model (SM) has long been shown to be the main key to understanding nuclear structure. It provides the theoretical framework for a microscopic description of nuclear properties essentially based on the use of effective interactions. In fact, as well known, within the shell-model approach only the particles outside a core made up of filled shells (valence particles) are considered to be active, and calculations are performed in a truncated Hilbert space, the so-called model space (Dean et al., 2004; Gargano et al., 2014).The SM has been successful in explaining the variation of neutron and proton energies and in predicting the observed properties of nuclei such as spins, parities and nuclear electromagnetic moments in different regions of the nuclide chart (Neyens, 2003). The essential assumption of this model is that neutrons and protons move independently in an average potential, interacting with each other through a residual interaction of a two-body character (Otsuka et al., 2002). The study of low-lying excited states of nuclei around double magic shells provides information about specific nuclear orbital nucleus because few nuclear orbits dominate the contribution to their wave functions. This is well proved by the attention focused on these nuclei in various recent papers (Al-Sammarraie et al., 2015). The calculation of transition probabilities in nuclear physics is a problem whose magnitude has developed substantially in the last decade .This is because we are continually challenged by new experimental results in several nuclei. Previous studies of low-lying states and transition probabilities for isotopes in the sd-shell region were studied (Kaneko et al., 2011; Mohammadi et al., 2017).
Brown has been the main person involved in expanding the shell-model code OXBASH. This code has been ported for different flavors of Unix operating systems. Several shell-model codes have been developed more recently such as m-scheme code ANTOINE and MSHELL and the J -scheme code NATHAN which are faster than ,However, OXBASH remains one of the most versatile of the existing codes. It has been extended to include up to about 250 m-states and 30 j-states. As far as we are aware the faster codes mentioned above have only been set up and work e going on to extend this OXBASH immediately allows one to do calculations in many different model spaces with several hundred existing interactions. OXBASH can calculate energies, one- and two-nucleon spectroscopic factors, one- and two-body transition densities and cluster overlaps. The package of programs called DENS can be used to generate spherical harmonic-oscillator, Woods-Saxon and HF radial wave functions, densities and HF binding energies. They can then be combined with the output of OXBASH to obtain the transition densities associated with beta decay, electromagnetic transitions and electron scattering. Together, OXBASH and DENS form a powerful set of tools for nuclear structure calculations .The goal of the code OXBASH is to reduce the dimensions of the matrix to be diagonalized by projecting angular momentum onto the m-scheme basis, and, therefore, focusing on states with definite angular momentum and isospin if desired and parity (Brown and Wildenthal,1988).
The allowable angular momentum states for two particles calculate from two theorems (Hasan and Hussain, 2013).
First theorem: if two identical particles in the same single particle orbit j (j half integer) can only couple their spins to even values of :-
The equations (4 and 5) to calculate the energies that consider only pure configuration states .However , the nucleons may be scattered from one state mixture of state must give the actual state. This mean that a term like should be added to equations (4 and 5), which can be written as:
The equations from (1 to 6) the oxbash program is ability to calculate them by executing certain commands after selecting the effective interaction and the space model to suit the selected isotopes in the study .So, we plan to continue to develop and refine this program.
We can describe the energy levels spectrum for (SDPN) model space with 18-20F isotopes at the configurations 0d5/2,1s1/2 and 0d3/2 above the 16O close core by using USDA and W Hamiltonians. In present work, we have used the OXBASH code in both m-scheme and jj-coupling. This program is a set of codes for carrying out shell-model calculations with dimensions up to about 50,000 in the J-T scheme and about 2,000,000 in the M-scheme. Oxbash comes with a library of model spaces and interactions (Hasan and Kareem, 2016). For light nuclei, there are various effective interactions such as USDA and W interactions. The universal Hamiltonian (USDA) was obtained from a least square fit of 380 energy data with experimental errors of 0.2 MeV or less from 66 nuclei (Brown and Wildenthal, 1988). The data were fitted from the lower and upper parts of the sd-shell by supposing that the simple mass dependence for the matrix elements was within (Wang et al., 2014):

Where A is mass number and P is equal to 0.3.
Brown and co-workers modified USD type Hamiltonian to USDA specified by 63 two body matrix element and three single particle energies for 0d5/2 0d3/2 and 1s1/2. The values are derived from the renormalized G matrix with modifications needed to reproduce the experimental binding energies and excitation energies for nuclei in the region A = 16 - 40 (Brown and Richter, 2006). The single-particle energies in unit MeV for protons and neutrons with 18F, 19F and 20F nuclei are 1.980, -3.944 and-3.061 as well as 2.112, -3.926 and -3.208 for USDA interaction and for 1.647, -3.948 and -3.164 for W interaction. The object of this present study is to calculate energy levels and reduced electric quadrupole transition probabilities B(E2;↓) by employing harmonic oscillator potential (HO, b), b<0 all isotopes and W and USDA interactions. The effects of core polarization have been taken into account in the calculations by effective charges of both protons and neutrons.
Energy spectrum
Tables 1 and 2 show the comparison between theoretical and available experimental data of18F nucleus (Tilley et al., 1995). The ground state of 18F nucleus is a close 16O core plus two nucleons distributed as one proton and one neutron in SDPN space at 0d5/2,1s1/2 and 0d3/2 configurations. Energy levels of 18F nucleus are correctly reproduced by using USDA and W results. Both USDA and W Hamiltonians agree reasonable well with empirical data. In the first sequences for energy spectra of USDA and W interactions, we predicted good corresponding for states 11+,31+,51+,01+ and 41+ with empirical values. Experimentally, the excited level 21+ was lower in energy than from theoretical value in both interactions. But in the second sequences of energy levels, we expected the states 32+,02+ and 42+ were rather close to the experimental data, but the ordering of the 22+ and 12+ states appear in experiment were reversed than the theoretical expected for the two interactions. While at the third sequence of energy levels, the order of 23+ and 13+ states was reversed in the experiment. New level 03+ was expected in both interactions which did not appear in the experiment. In other sequences, the state 26+ corresponds with the experimental value of both USDA and W interactions. New energy levels were predicted for the states 35+,27+ ,16+,36+,17+,and 28+; for USDA interaction at energies11.934, 12.587, 12.613, 15.377, 16.284 and 17.345 MeV respectively, but for W interaction at energies 11.749, 12.122, 12.535, 15.345, 15.405 and 16.887 MeV; 35+,27+,16+, 17+,36+ and 28+ states were not observed in the experimental data.
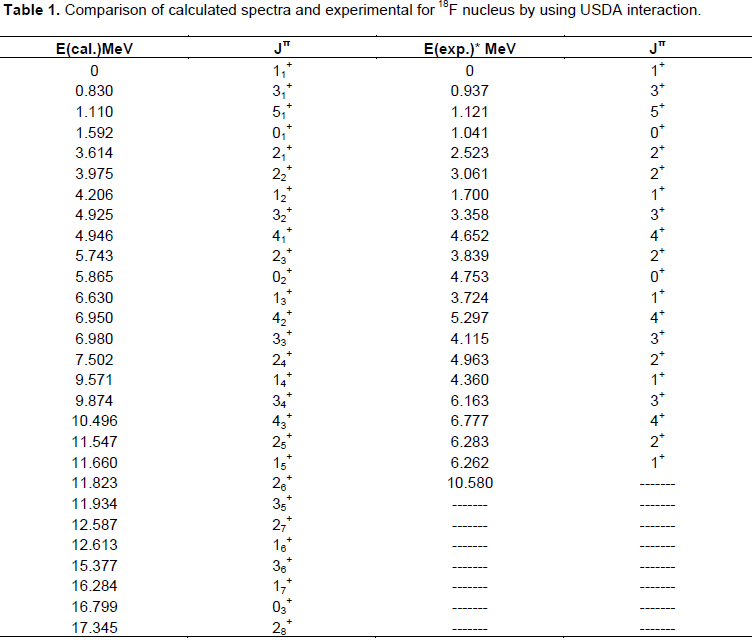
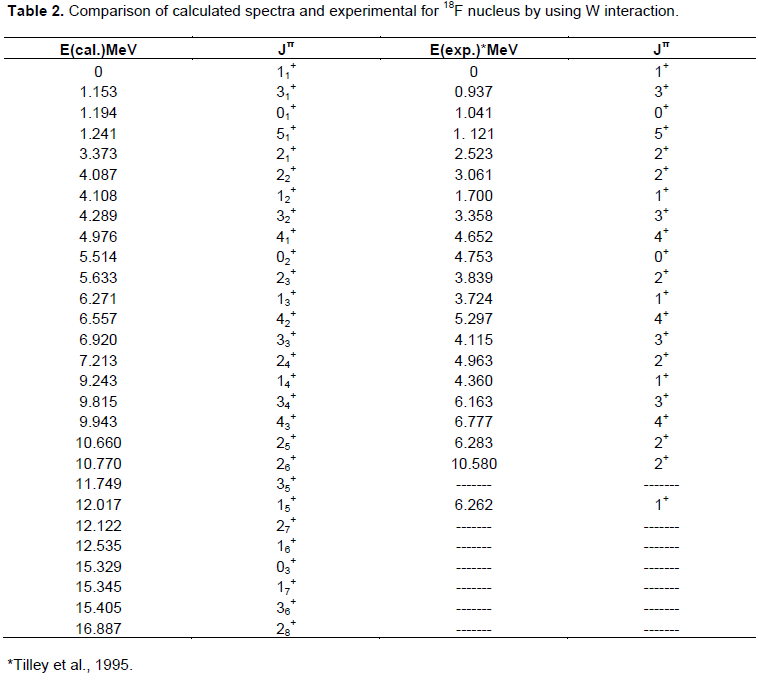
The calculated energy levels and experimental results of low-lying states in the 19F nucleus are presented in Tables 3 and 4. The 19F nucleus contains one proton and two neutrons over the 16o core in SDPN space at 0d5/2,1s1/2 and 0d3/2 configurations. The excited levels in USDA and W calculations of the first sequence corresponded well with some of the experimental values (Tilley et al., 1995), except the 5/21+ level which appears lower in energy than the experimental value. Good agreement was found in the USDA and W results for energy levels in the second sequence with the empirical values, except the 3/22+ state in the experimental data, which appears lower in energy than the theoretical expected in both two interactions. The excited states 5/22+,7/22+,1/22+,11/22+ and 13/22+ in both interactions agree with the experimental values. In the three sequence for energy levels, we predicted that they correspond with the available experimental data of the states, 7/23+ and 9/23+ at energies of 6.070 and7.929 MeV respectively for W interaction results, but the energy value, 9.834 MeV of 11/23+ state in both two interactions correspond with the empirical value. In another sequence, the state 11/24+ at four sequence of energy levels for the USDA and W results with energy values of 12.591 and 12.739 MeV respectively was not specified experimentally. We predicted that the 11/25+ state identified with the experimental value of 16.090 MeV in the five sequence for energy states. The USDA and W results in the six sequence identified the 11/26+ state with energy of 18.920 MeV, which was non- empirical data. The experimental energy states with values of 13.170, 14.240, 15.000 and 15.560 MeV identified with 9/27+, 9/28+,1/29+ and 1/210+ states which agrees with the theoretical prediction for the two interactions in this present study, but unidentified at spins.
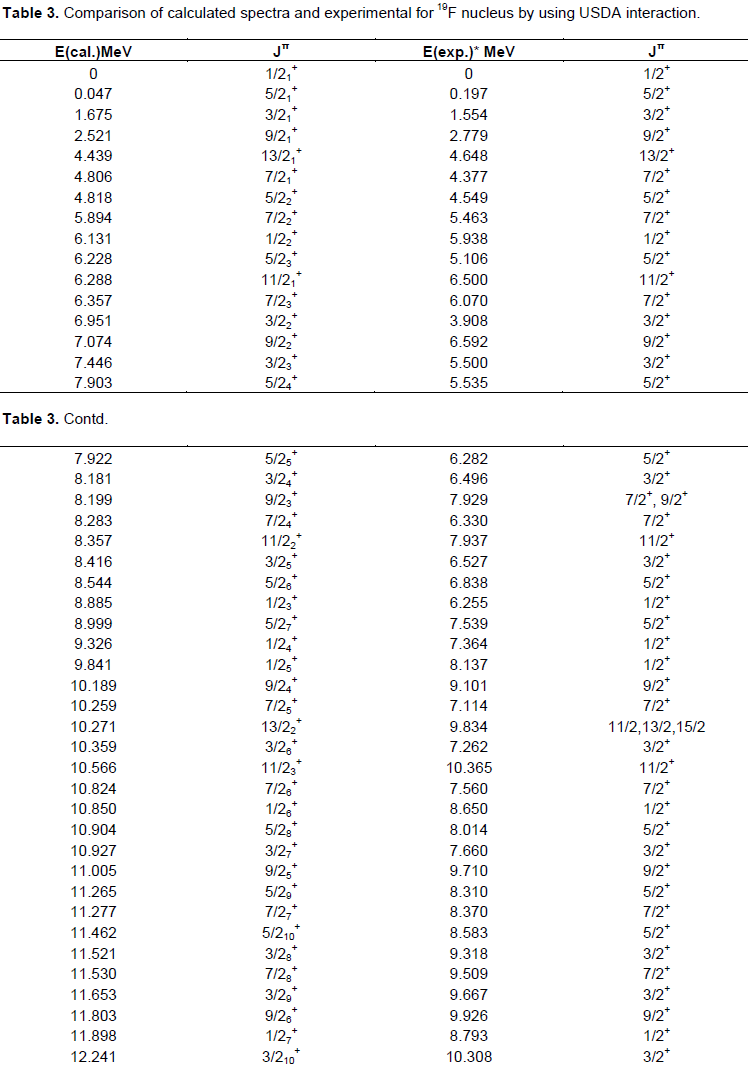
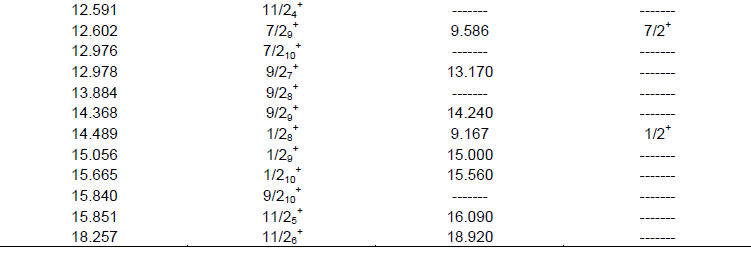
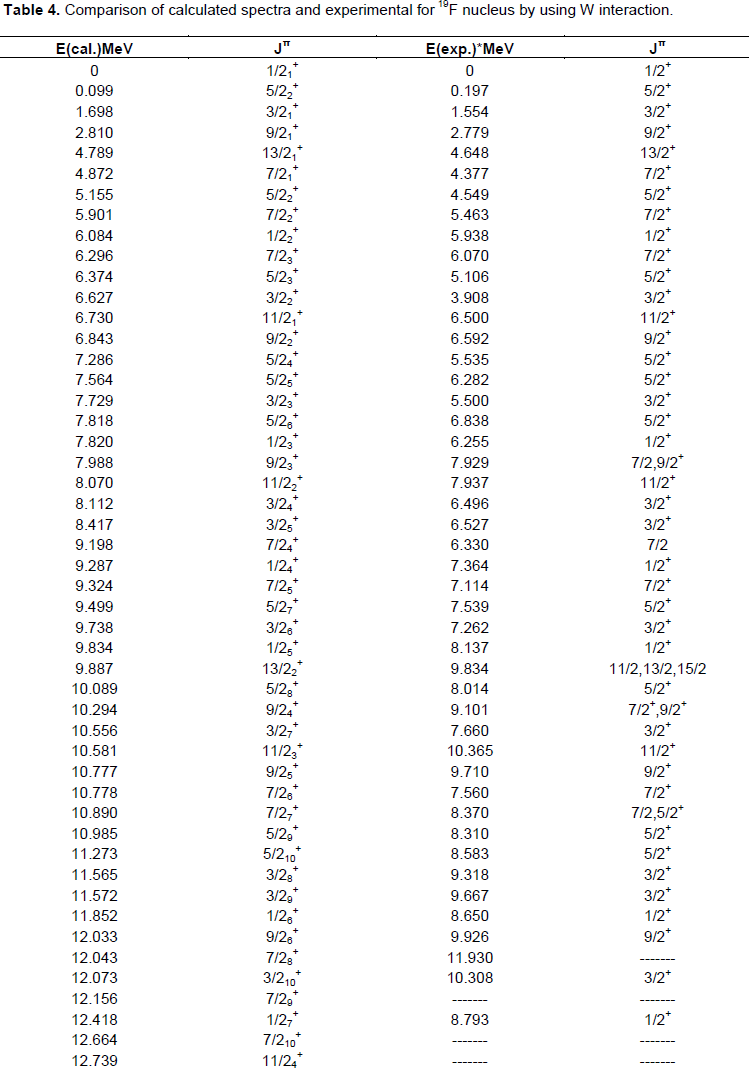
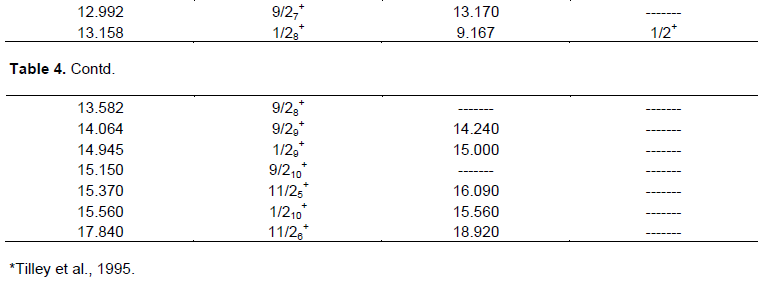
According to the shell model, the ground state of 20F nucleus, a close16o core with four nucleons has one proton and three neutrons distribution in SDPN space at 0d5/2, 1s1/2 and 0d3/2 configurations. For this nucleus, as illustrated in Tables 5 and 6, the order of first sequence for the excited states is well reproduced. In USDA interaction, the 41+ state corresponds with the experimental value. While in W interaction, 11+ and 01+ states agree reasonably with the experimental values (Tilley et al., 1998). The 71+,61+ and 81+ states identified for experimental values of 4.208,4.518 and 9.200 MeV respectively, which agrees with the theoretical prediction in both two interactions. In the second sequence, the order of energy states is well reproduced with the empirical states of W interaction .While in USDA interaction, the 32+ state is higher in energy than the experimental values. W interaction results predicted that the 22+and 02+ states correspond well with the experimental values and also confirm the experimental states 32+ and 52+ which agree with the experimental values from the theoretical results predicted. Experimentally, the energy 6.458 MeV was identified with the 62+ state from our calculations in both interactions in this study. New level at 72+ state is predicted in USDA and W interactions. For the third sequence of energy states, 33+ experimental state corresponds with the USDA and W predicted. There are many unconfirmed values of the experimental data after the 33+ state have been not affirmed in our theoretical results at the levels 3.586, 4.509 and 5.310 MeV. Excited experimental levels, 6.391, 8.113 and 8.349 MeV identified with the states 53+, 03+ and 63+, respectively theoretically, which appears to have a reasonable agreement at energies with USDA and W predicted. Shell model predicted for USDA and W results with empirical values for another sequences has good agreement. The experimental levels 3.761, 5.066 and 5.555 MeV affirmed with the 34+, 24+ and 14+ states, which agrees at energies with the USDA predicted than the W predicted. The experimental values at energies 6.111, 7.232, 8.500, 9.850 and 12.000 MeV were identified with the states 44+, 54+, 04+, 64+ and 74+, which agrees well with the USDA predicted. The agreement between the experimental values 5.319, 5.465 and 5.810 MeV with W results is good and also confirmed the 15+ state of the energy 5.810 MeV. USDA and W calculations, identified the 45+, 55+ and 05+ states for the experimental data 6.936, 8.062 and 11.490 MeV. In both interactions, there is a new level at the 75+ state, which is not found in the experimental data. The excited level 26+ was expected rather close to the experimental value by the USDA interaction predicted. There are many unconfirmed states of the experimental values 5.764 and 7.080 MeV after the 26+ state was confirmed in our results with the 36+ and 16+. The excited experimental energies 7.283, 8.940 and 12.200 MeV were identified with the 46+,56+ and 66+ states for two interactions. The 27+state at energy 6.856 MeV was rather close to the USDA results predicted than the W predicted. The levels after the 27+ level were unconfirmed at spins experimentally, which affirmed theoretically with the states 37+ and 17+. The experimental energy values 8.421, 9.650, 12.700 and 13.200 MeV agree with USDA expected and specified with the states 47+,57+,67+and 07+ from our theoretical results. Affirmed, the parity of the experimental 7.166 MeV energy value which slightly close to for W predicted. Identified, the parity of experimental energy 7.985 MeV which agrees with USDA result than from W result predicted.
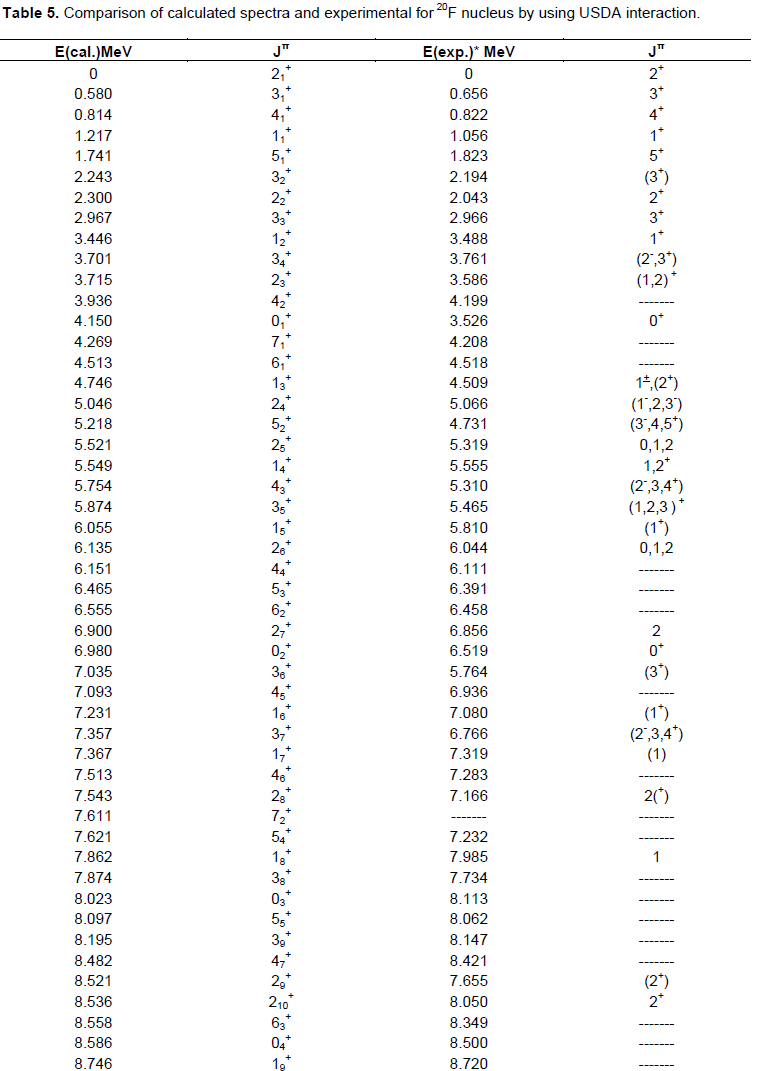
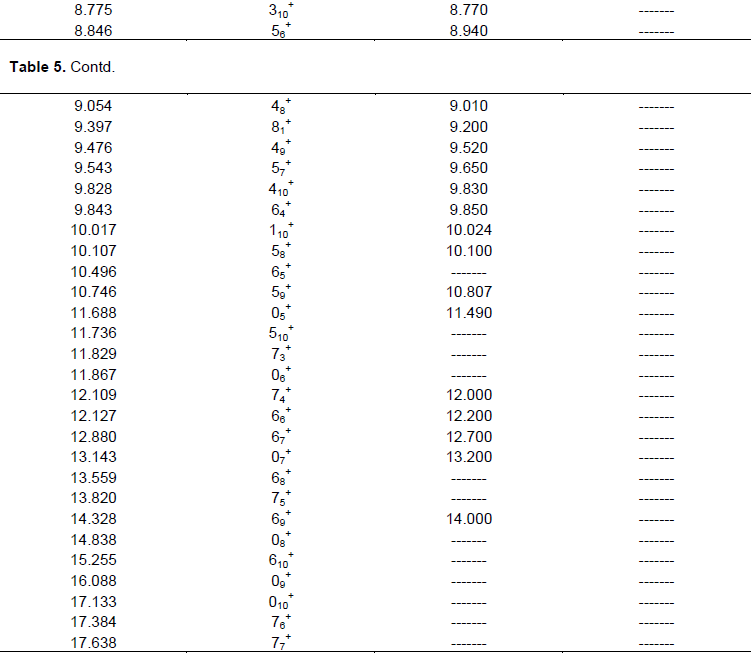
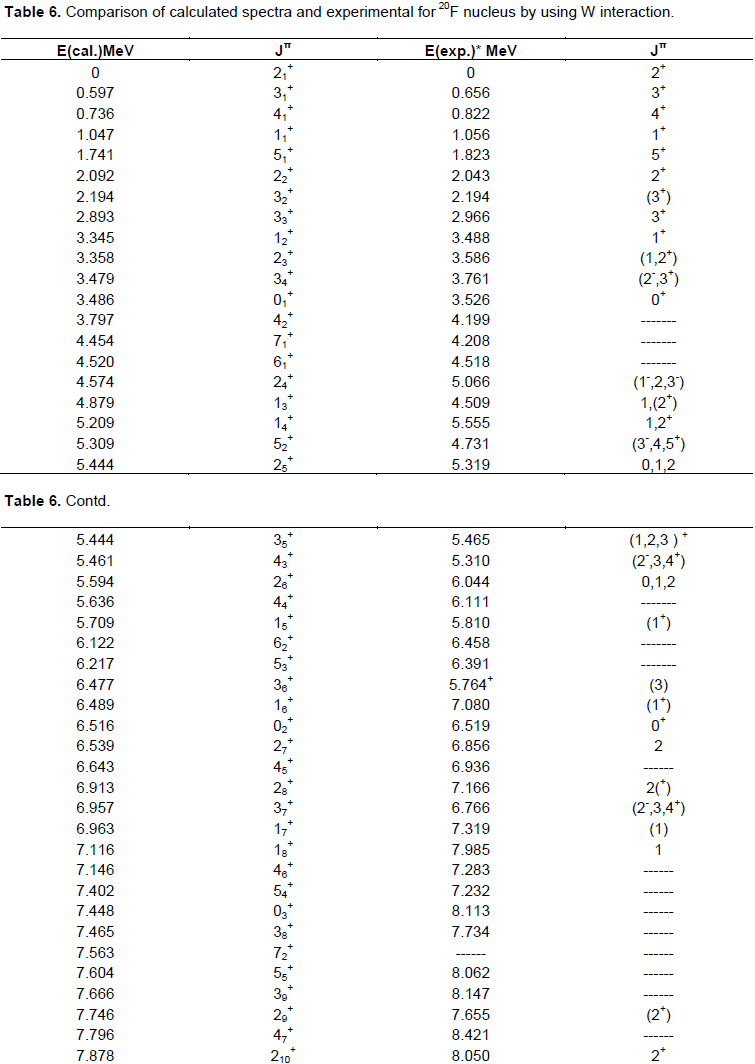
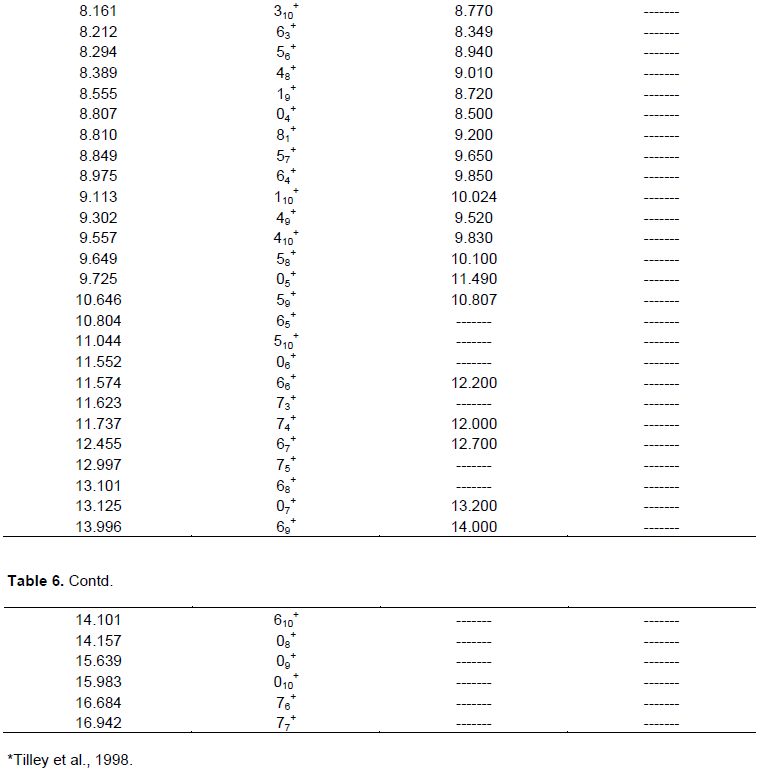
The experimental values at the energies 7.734, 9.010 and 10.100 MeV were specified with the 38+, 48+ and 58+ states from our theoretical result, which corresponds with the USDA predicted. New level at 08+ in both two interactions was not indicated in the experiment. The agreement between the experimental values 8.147, 8.720, 9.520, 10.807 and 14.000 MeV and USDA expected is good; we also specified these levels with 39+, 19+, 49+, 59+ and 69+ states from our calculations predicted. The experimental level 7.655 MeV at spin 2+ was confirmed which agrees with the W predicted. While the 09+ was a new level which no indication experimentally.
Experimentally, the level 8.050 MeV was rather close to the energy value for W result. While the energy values 8.770, 9.830 and 10.024 MeV agree well with the USDA predicted and identified with the 310+, 410+ and 110+ states in our theoretical calculations predicted.
Reduced electric quadrupole transition probability B (E2; ↓) values
The transition rates represent a sensitive test for the most modern effective interactions that have been developed
to describe sd-shell nuclei. The transition probability calculation in this present work was carried out using the harmonic oscillator potential (HO, b), where b < 0 for each in-band transition and application USDA and W interactions for 18F, 19F and 20F nuclei at SDPN model space. In general, the calculated results agree reasonably well for all the nuclei in this paper with available experimental data (Tilley et al., 1995, 1998) (Table 7).
In this paper, the agreement between theoretical and experimental levels is satisfactory for excitation energies and transition probabilities B(E2; ↓). There are many unconfirmed experimental energy levels confirmed by our calculations and new values for B(E2; ↓) which were not indicated in the experimental data .The choice of model space SDPN and (USDA and W) effective interactions are adequate in this mass region. The theoretical calculations for nuclear shell model by using OXBASH code for windows reasonably agree with the experimental data. This indicates that the shell model is very good to describe the nuclear structure for 18F, 19F and 20F nuclei.