ABSTRACT
Termitarian, the epigeal biogenic structure constructed by termites are widespread in the forest and derived savannah regions of Nigeria. Soil modification, with particular focus on the mineralogy, variation in geochemical composition and geotechnical properties of termite mound soil samples collected from nine location in the Ika area, Delta State of Nigeria are the objectives of this report. The major elements geochemical composition of the termite mound soil samples determined by XRF was found to be mainly SiO2 (40.25%), Fe2O3 (29.27%), Al2O3 (24.25%) and T1O2 (4.27%). The other major elements; CaO, MnO, MgO, Na2O and P2O5 were present at less than 1%. Trace element compositions of the termite mound soil samples were found to be Cr (8.20- 23.10 mg.kg-1), Pb (3.95- 13.85 mg.kg-1); Cu (2.75-12.00 mg.kg-1), Zn (3.60-9.60 mg.kg-1) and Cd (1.90-3.15 mg.kg-1). Minerological composition of the termite mound soil samples determined by XRD revealed significant hematatic composition with a range of 60.17-83.78%, antigorite (5.24 -24.17%) and quartz (7.14-14.67%). Anthophylite (19.92%) was found in only one termite mound soil samples. The geotechnical tests carried out on the termite mound soil samples included: Grain size, atterberg limit, compaction and triaxial tests. The range of values of the determined properties: Uniformity coefficient (3.75-7.50), coefficient of curvature (0.20-2.70), plasticity index (10.83-28.45%), plasticity limit (13.47- 19.57%), optimium moisture content (7.20- 15.40%), maximum dry density (1.72-2.10g.cm-3), angles of friction (2.83- 24.77°) and cohesion of up to about 50.11 KN.m2, suggest that the termite mound soil may have limited application in soil stabilization and concrete works.
Key words: Ika, geotechnical, geochemical, termite, mound.
The presence of living organisms is a major factor that distinguishes soils from disintegrated rock fragments from which they were derived. The wide variety and vast number of organisms in soil interact with environmental factor in complex cyclical and evolutionary process that transform fragmented rocks into mature soil. There is a vast literature on the role of organisms in soil processes and soil formation and in particular the interaction of microorganisms and invertebrates in decomposition and mineralization processes in becoming letter understood (Anderson, 1988). In general, soil provides a more stable environment for organisms than condition above the ground and therefore serves as a refuge for many epigeal invertebrates (Wood, 1988). In tropical regions, soil invertebrates macrofauna play a key role in organic matter, recycling (Levelle, 1994). The invertebrate fauna is dominated by termites in terms of density. Termites are terrestrial insects found approximately between 45°N and 45°S. Within these latitudinal limits they are restricted by a combination of extreme aridity and lack of vegetation and are rarely found at altitudes above 3000 m. Within this range, termites can be found in all soil types, except those which are semi- permanently water-logged and severally cracking vertisols (Wood, 1988). The impact of termites is usually due to their building ability where mounds (termitaria) represent spectacular biogenic structures. Termite mound density can be very high covering substantial land area (Levelle, 1994) and limiting available land for cultivation (Maduakor et al; 1995). The mode of mound construction differs among termite species: soil feeding species build their mounds with soil particles glued together with faeces (Wood et al., 1983); while funges – graving species mix soil particles with their saliva (Grasse, 1984). It has been suggested that mound building activity results in the upward transfer of clay, silt, sand and fine grain particles to the surface, a process which is opposite to leaching and this results in significant mobilization and dispersion of trace elements (Roquin et al; 1991; Chatupa and Dieng, 2000). The use of termite mound soil sampling in geochemical exploration has these become an active area of research (D’orey, 1975; Kedebe 2004; Petts et al; 2009). The ecological and geochemical significance of termite mound soils requires their systematic investigation. In this paper, the mineralogical and geochemical compositions and geotechnical properties of termite mound soil in the Ika area Delta State, Nigeria are reported.
Geological setting
The termite mound soil samples were collected from the Ika area, Delta State, Nigeria. The area is located in the Niger Delta Basin, Southern Nigeria within latitudes 5° and 6°30 N and longitude 5°40’ and 6°50’E. The Miocene-Recent Benin Formation (Reyment, 1965) typifies the Niger Delta basin and it is underlain successively in the north eastern (surface) Niger Delta by the paretic Ogwashi- Asaba formation, the Eocene Amiki Formation and the Paleocene Imo Formation. In the southwestern (subsurface) Nigeria, three diachronous units; (from top to bottom), Benin, Agbada and Akata Formations have been identified (Short and Stauble, 1967). The procleltaic marine Akata Formation composes mainly of shales and the poralic Agbada Formation consists mainly of sequences of sandstones shales, while the Benin Formation consists of sandstones and unconsolidated to partially consolidated sands and gravels with clay lenses (Nesbilt and Young, 1989) Figure 1 shows the geological setting of the study area.
Collection of termite mound soil samples
Termite mound soil samples were collected from nine locations (Abavo, Agbor Ekuku- Agbor, Idumuesah, Igbodo, Otolokpo, Owa, Umunede and Ute-Okpu) in the Ika area of Delta State (Figure 2). At each location, samples were taken from the top, middle and base of each of five mounds and composited. The samples were dried, ground in an agate motar and representative sample were taken for analysis.
Analyses of samples
The mineralogical composition of the termite mound soil samples was determined by x-ray diffraction (XRD) method using the Brauker D8 Advance x-ray diffractometer with a Lynx Eye detector. The scanning covered the 2Ï´ intervals of 4- 70° with a scanning angle step of 0.015° and a time step of 1 s. The mineral phases were quantified using a Riveted – based quantification routine with TOPAS® software.
Analysis for geochemical composition of the termite mound soil for samples was carried by x-ray fluorescence using the XRF – 1200 ARL, Advant’s thermoscientific. Trace elements determinations were carried out on acid digestates of the termite mound soil samples using a Shimadzu GFA – 7000A AAS. The geotechnical tests were conducted in accordance with the BSI 1377 methods (1998). The percentage of difference sizes of the termite mound soil particles determined by sieve analysis were plotted as distribution (gradation) curve which allowed the uniformity coefficient Cc and coefficient of curvature, Cu of the test mound soil samples to be determine. Consistency limits were determined by the Atterberg tests and compaction test was used to determine the maximum dry density and optimum moisture content of the termite mound soil samples. Shear strength measured as cohesion and friction angle was determined by triaxial test.
Minerological composition
The whole sample mineralogical composition of the termite mound samples are given in Table 1. All the termite mound soil samples revealed significant hematite content; with a range of 60.17 – 83.78% and an average composition of 72.51%. Hematite the mineral form of iron (ii) oxide (Fe2O3) is one of the several oxides widespread in rocks and / or highly weathered soils and along with other iron oxides or oxyhydroxides such as goethite is responsible for the red colour of many tropical soils. Antigorite, (MgFe)3 SiO5 (OH)4, a form of serpentine mineral was the next most abundant in the termite mound soil samples studied, with a range of 5.24-24.17% and an average value of 14.86%; which quartz occurred in the range 7.14- 14.67% with an average value of 10.41%. Quartz mineral, the second most abundant mineral in Earth’s continental crust after feldspar, composes of silicon and oxygen in a continuous framework of SiO4 silicon oxygen tetrahedron and occurs in all mineral environments and is an important constituent of many rocks. Anthophyllite, Mg2 Mg5 SiO8O22 (OH) magnesium iron inosilicate hydroxide, was found only in the termite mound soil sample from Abavo (19.92%). It is a rare stone that belongs to the Amphibole group found in schists and genises that are characterized by shales of brownigrey and off white colour, derived from magnesium rich metamorphic rocks found only in a few geographical locations (which may now include Abavo). The notable absence of clay minerals in the termite mound soil samples is significant and relevant in the potential geotechnical application of the termite mound soil.
Major element geochemistry
The concentration of the major elements analyses in the termite mound soil samples are given in Table 2. It can be seen that the termite mound soil samples contain mainly SiO2 (40.25 ±1.14%), Fe2O3 (29.27±1.31%), Al2O3 (24.25 ± 0.92%) and TiO2 (4.27± 0.46%). The amounts of MnO, MgO, CaO, Na2O and P2O5 were all less than 1%. The use of geochemical indices, SiO2/Al2O3, K2O/Al2O3, Al2O3/TiO2 etc. is important in relating chemical composition of sandstones to classification of terrigenous sands and shales and may be applied to the termite mound soil samples from the area of study. Cox et al. (1985) used the K2O/Al2O3 rates to uses the original detrital mineralogy of mudstone with values of the index greater than 0.5 indicating dominance of alkali K- feldspar in comparison with other minerals; and values less than 0.4 suggesting minimal feldspar in the original mudstone.
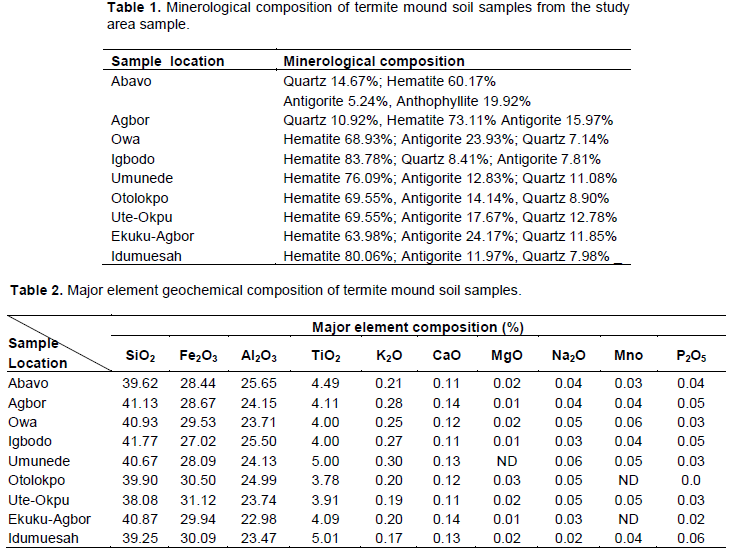
The values of the K2O/Al2O3 index for all the termite mound soil samples were all below 0.4, with a range of 0.007-0.012, and an average value of 0.009, thus indicating that the termite mound soils X have minimal K- feldspar. The SiO2/ Al2O3 index can be used as indicator of mineralogical maturity and allow for distinction between quartz-rich high ratio sandstones and clay-rich low-rate shales (Pettijohn et al., 1972). The values of this index for the termite mound soil samples are about the same order of magnitude varying somewhat within the range 1.55-1.78, suggesting that the soil samples are quartz rich with high-ratio sandstones deficient of clay minerals. This is in line with the results of mineralogical composition which gave an average value of quartz content of the termite mound soil of 10.41% and absence of clay minerals. The Fe2O3/K2O ratio may be thought of as an indicator of mineralogical stability. At the low temperature and pressure characteristic of sedimentary environment, the most stable rock-forming minerals are K-feldspar, muscovite, mican and quartz (Herron, 1988). In contrast, the less stable rock-forming minerals occurring commonly in lithic fragment tend to be rich in iron and magnesium. As a general rule, stable mineral assemblage have low Fe2O3/K2O values less stable assemblage located close to the sediment source and containing abundant lithic fragments have high Fe2O3/K2O values (Kedebe, 2004). A careful examination of the results in Table 2 indicate that the values of Fe2O3/K2O index are relatively high with a range 93.63- 230.94 and on average value of 144.58 + 30.08. These high values of the Fe2O3/K2O index may indicate Fe-rich shales or Fe-rich sands, the latter being more likely for the termite mound soils under study. The K2O/CaO ratio is a useful index for charactering source area weathering (Mesida, 1981), with values less than 1.0 indicative of unweathered source rock and values greater than 1.0 suggestive of weathered source rock. The values of K2O/CaO obtained for the termite mound soil (1.91-6.29) suggest a high degree of weathering, and is corroborated by the elevated values of TiO2/Na2O index (75.60-250.5). The Al2O3/TiO2 ratio is orderly used for the discrimination of source rock littology: low values 3 to 8 indicative of mafic igneous rocks, 8 to 21 for intermediate rocks and 21 to 70 for felsic igneous rocks (Hayasali et al., 1997; Ocheli et al., 2018).
The value of Al2O3/TiO2 obtained for the studied termite mound soils were in the range 4.68-6.61 supporting a line to passive continential margin (Ogala et al., 2015).
Weathering indices
Chemical index of alteration (CIA), chemical index of weathering (CIW). Plagioclase index of alteration (PIA) and mineralogical index of alteration (MIA) given as (Ocheli et al., 2018) are useful in the determination of the degree of weathering; a measure of the ratio of secondary aluminous minerals to feldspar (Eizien et al., 2004). Low values (of about 50) of weathering indices suggest unweathered or weak weathering, while values of 70-100 indicate intense weathering in which alkali and alkaline earth metals have been significantly removed relative to Al2O3 (Fello et al., 1995).
Intensity of weathering is indicated from values of MIA: 0-20% weak, 20 - 40% moderate, 40 - 60% intense and 60-100% extreme intensity of weathering. The range of values of weathering indices: CIA 98.13 - 99.08%; CIW 99.23 - 99.58%, PIA 100.13 - 101.66% and MIA 97.08- 98.84% indicate clearly that the termite mound soils, as expected, are extremely weathered.
Trace elements geochemistry
The concentrations of selected trace elements in the termite mound soils are given in Table 3 in comparison with adjacent soil samples collected at 10 m from the base of the mound soil. The most abundant trace element in the termite mound soil was Cr (average value 15.86 mg.kg-1), followed by Pb (7.44 mg. kg-1) while Ca (2.51 mg. kg-1) was the least abundant. The same pattern of variation was observed for the concentration of the trace elements in the surrounding soil samples: Cr (average value 10.33 mg. kg-1); Pb (5.79 mg. kg-1); Cu (3.98 mg. kg-1), Zn (3.67 mg. kg-1) and Cd (2.10 mg. kg-1). These results show marked increase in the concentration of Zn, Cr and Cu in the termite mound soils of 50.41, 53.53 and 60.05% respectively over the in the surrounding top soil samples. The relative increase of Pb (28.49%) and Cu (19.52%) in the termite mound soils in comparison with the surrounding top soil samples were less marked. The spatial variation of the total trace elements in the termite mound soils and surrounding soil samples given in Figure 3 are in the range 22.55-50.65 mg. kg-1 and 15.56 - 41.40 mg.kg-1 respectively and are apparently significant, and may indicate variations in the sub- surface geochemistry of the area.
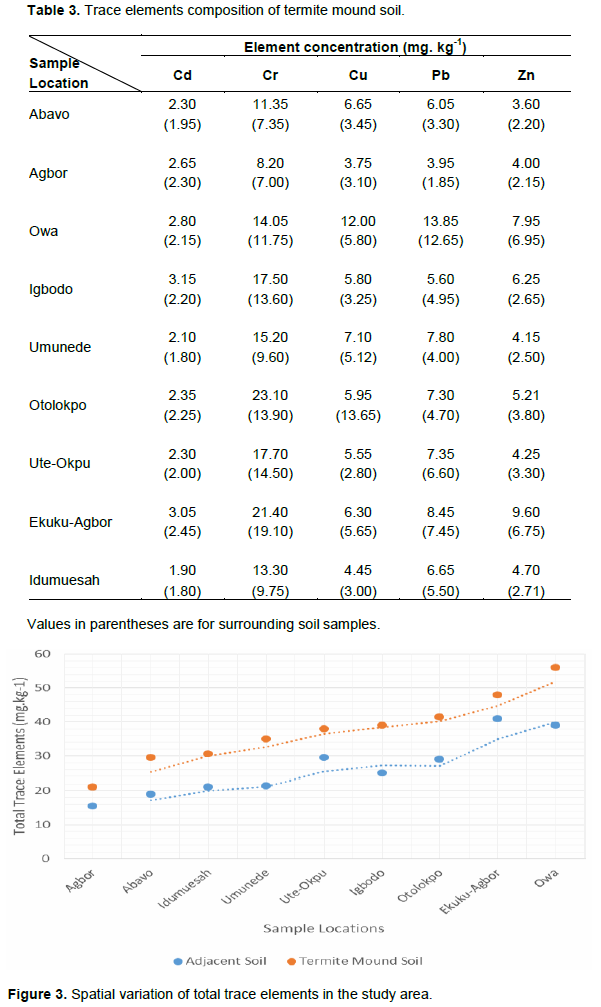
Geotechnical properties of termite mound soil
The results of the geotechnical tests carried out on the termite mound soil samples are given in Table 4. Typical particles size distribution curve for particles size analysis of the termite mound soil sample is given Figure 4. It revealed that the termite mound soils are largely constituted of fine to medium grain sands. The particle size indices uniformity coefficient, Cu and coefficient of curvature Cc obtained for the mound soils varied from 3.75 - 5.70 and 0.20- 2.70 respectively, suggesting that at the mound soils are coarse, poorly graded and poorly sorted (Hoitz and Kovacs, 1981; Das and Sobhan, 2013). The liquid- plastic - semi - solid - solid states of aqueous slurry, Atterberg limits of the termite mound soils are given in Table 4. The values of plasticity index, 0.82- 28.45% indicate that the mound soils have low plasticity (Clayton and Juckes, 1978) medium plasticity (Burmister, 1948) and are cohesion (Prakash and Jani, 2002).
The specific gravity of the mound soils varied within a narrow range; 2.34- 2.58 and within the organic soil range (1.0Ï´ - 2.60) (Bowles, 2012). These results suggest that the mound soils may not be suitable materials for civil construction. The moisture content at which soils are compacted in the field is controlled by its optimum moisture content, while the compaction energy applied is determined by its maximum dry density. The optimum moisture content (OMC) and maximum dry density (MDD) of the mounds soils can be seen in Table 4 to vary from 7.20- 17.10% and 1.74 and 2.10 g.cm-2 respectively. The mound soils can therefore be classified as sandy soil (O’Flanerty, 1988) or loose with little amount of clay (Ishaku et al., 2002). The shear strength parameters of soils defined as cohesion and friction angle depend on the effective stress, drainage conditions, density of the particles rate of strain and direction of stain (Roy and Bhalla, 2017). These shearing strength is affected by the consistency of the material, minerology, grain size distribution; shape of particles, initial void ratio and features such as layers, joints, fissures and cementation (Poulos, 1989). The values of shear strength indices; cohesion and angle of friction for the mound soil have wide spatial variation; 0.00- 50.11 KN.M-2 and 2.83-24.77° respectively. These relatively low values of shear strength indicator may be related to grallation and consistency of the mound soils; and suggests that the mound soils may have limited soil engineering applications.
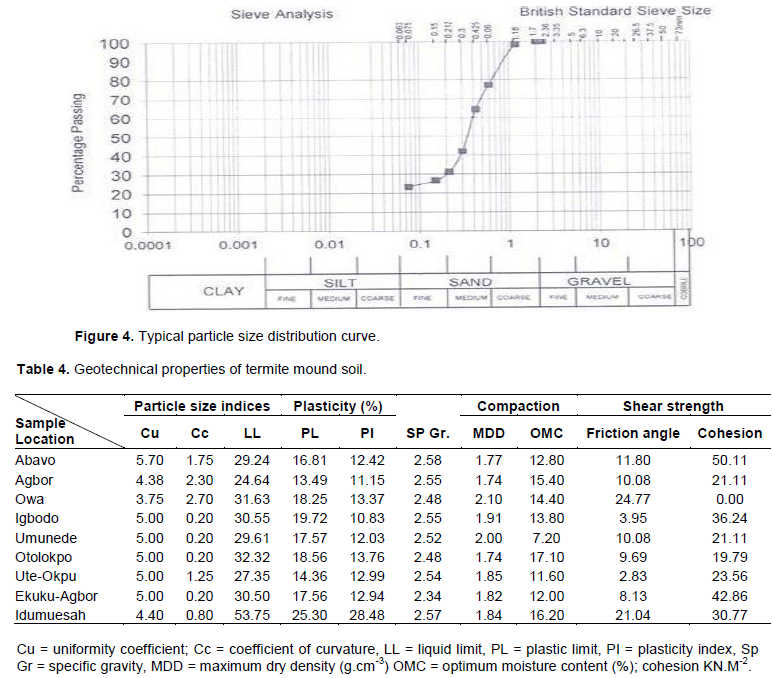
The authors have not declared any conflict of interests.
REFERENCES
Anderson JM (1988). Spatiotemporal effects of invertebrates on soil processes. Biology and Fertility of Soils 6:216-227.
Crossref
|
|
Bowles JE (2012). Engineering Properties of Soils and their Measurements, 4th edn, Mc Graw Hill Education (India).
|
|
|
British Standards Institution 'BS EN 1997-2-2007, Geotechnics Designs (Ground Investigation and Testing, section 5.) British Standards Institution, 'British Standards Methods of Test for Soil for Civil Engineering Purposes', BS1377. London, 1990.
|
|
|
Burmister DM (1948). Concept in Soil Mechanics, 2nd edn; Department of Civil Engineering, Columbia University.
|
|
|
Chatupa JC, Dieng BB (2000). Distribution of trace and major elements in the - 180 + 75 NM Fractions of the sandveld regolith in Northwest Ngami land, Botrwana. Journal of African Earth Sciences 30(3):515-553.
Crossref
|
|
|
Clayton CR, Jukes AW (1978). Standard Range of Plastic Limits of Soils. London Royal Charter.
|
|
|
Cox R, Lower DR, Cullers RL (1995). Influence of sediment recycling and basement evolution of mudrock chemistry in the Southeastern United States Geochimica et Cosmochimica Acta 59:2919-2940.
Crossref
|
|
|
Das BM, Sobhan K (2006). Principles of Geotechnical Engineering, edn. Cengage Learning, Boston, USA. 771p.
|
|
|
D'Orey FLC (1975) Contributions of termite mounds to locating Ridden copper deposits. Trans. Institu. Miner. Metallorgy 84:150-156.
|
|
|
Eizien SM, Farah AA, Alhaji AB, Mohamed BB, Al-Imam OA, Hussein BB, Khalid MK, Hamed BO (2004). Geochemistry of Merkhiyat sanstones. Omdurman Formation, Sudan: Implication of depositional environment, provenance and tectonic selting. International Journal Environmental and Agricultural Science 2(3):10-15.
|
|
|
Fello CM, Nesbitt H, Young GM (1995). Unraveling the effects of potassium metasomatism sedimentary rock and paleosois with implications for provenance and paleo weathering conditions. Geochim. Cosmochim. Acta 23:921-924.
Crossref
|
|
|
Grasse PP (1984). Termunologia Foundation Deesocieteet construction. Tome 11: Mason P 613.
|
|
|
Hayasali K, Fujisawa H, Holland HD (1997). Geochemistry of 1.9 Cra sedimentary rocks from Northeastern Labrador, Canada. Geochim: Cosmochum. Acta. 61:4115-4137.
Crossref
|
|
|
Herron MM (1988). Geochemical classification of terrigenous sands and shales from core or log data. Journal of Sedimentary Petrology 58(15):820-825.
Crossref
|
|
|
Hoitz R, Kovacs WD (1981). An Introduction to Geotechnical Engineering. Prentice Hall Inc.
|
|
|
Ishaku JM, Adekeye JID, Negro SS (2002). The hydrogeochemical and geotechnical characteristics of rock materials that aid gulley erosion in Lassa area of Borno State. Northeastern Nigeria. Water Resources Niger. Assoc. Hydrogeology 13(11):53.
|
|
|
Kedebe F (2004). Use of termite mounds in geochemical exploration of North Ethiopia. Journal of African Earth Sciences 40:101-103.
Crossref
|
|
|
Lavelle P (1994). Faunal activities and soil processes: adaptive strategies that determine ecosystem function. Advances in Ecological Research 27:93-132 Academic Press.
Crossref
|
|
|
Maduakor HO, Okere AN, Onyeanuforo CC (1995). Termite mounds in relation to surrounding soils in the forest and derived savannah zones of Southeastern Nigeria. Biology and Fertility of Soils 20:157-162.
Crossref
|
|
|
Mesida EA (1981). Latentu on the highway-understanding soil behavior. West African Technical Review, November 1981, pp.112-118.
|
|
|
Ocheli A, Okoro A, Ogbe OB, Aigbadon GO (2018). Parent rock assemblages, weathering intensity and tectonic settings of the late cretaceous sediments, western Anambra Basin; Nigeria. Journal of Applied Science and Technology 36:24-42.
|
|
|
O'Flaherty CA (1988). Highway Engineering. Vol 2. Edward Arnold Publishers, London. UK.
|
|
|
Ogala JE, Olabaniyi Cano-Irabor OO, Adaikpoh EO (2015). Petrographic and geochemical study of the maastricrtian Ajali sandstone, North Central Nigeria. Geological Survey Bulletin 59(1):79-90.
Crossref
|
|
|
Pettijohn FJ, Potter PE, Siever R (1972). Sands and Sandstones. Springer Verlag. Newyork. 618P.
Crossref
|
|
|
Petts AE, Hill SM, Worrall L (2009). Termite species variations and their importance for termitaria biogeochemistry: towards a robust media approach for mineral exploration. Geochemistry Exploration Environment Analysis 9:257-266.
Crossref
|
|
|
Poulos SJ (1989). Liquefaction Related Phenomena. In: Advanced Dam Engineering for Design, Constrcution and Rehabilitation. Jansen. R. B (Ed). Van Nostrand Reinhold. pp. 292-320.
|
|
|
Prakash S, Jani PK (2002). Engineering Soil Testing Nem Chand & Bros: Roorkee.
|
|
|
Reyment RF (1965). Aspects of Geology of Nigeria. University of Ibadan Press. 145P.
|
|
|
Roquin C, Freyssinet PH, Navikuff A, Tardy Y (1991). Geochemistry of termitaria and soils covering ferricrete: Application of gold exploration in West Africa. European Network on tropical laterite and global environment. Eurolat 'q1, Supergene are deposits and mineral formation; 5th international meeting, pp. 133-137.
|
|
|
Roy S, Bhalla SK (2017). Role of geotechnical properties of soil on civil engineering structures. Resources Environment 7(4):103-109.
|
|
|
Short KC, Stauble AJ (1967). Outline of geology of Niger Delta. American Association of Petroleum Geologists bulletin 51: 761-779.
Crossref
|
|
|
Wood TG (1988). Termites and the soil environment. Biology and Fertility of Soils 6:228-236.
Crossref
|
|
|
Wood TG, Johnson RA, Anderson JM (1983). Modification of the soil in Nigerian savannah by soil - feeding Cubitermes (Isoptera termitidea). Soil Biology and Biochemistry 15:575-579.
Crossref
|
|