ABSTRACT
Breeding for drought tolerance based on direct selection for high grain yield under drought has been hindered by the complex nature of drought tolerance mechanisms and the approaches used. Molecular marker-based approaches are a promising alternative. In this study, 30 rice (Oryza sativa L.) accessions cultivated in Nigeria were screened in a greenhouse for drought tolerance based on morpho-physiological traits and assessed for DNA polymorphisms using SSR markers for possible marker-trait associations. Our results showed that five Nigerian rice landraces (IJS-02, IJS-09, IK-PS, IK-FS and Lad-f) and three improved varieties (FARO-44, IR-119 and IWA-8) were highly drought tolerant. Sixteen of 20 markers tested yielded amplified products and generated 221 alleles (4 to 5 alleles per marker) with PIC values ranging from 0.24 to 0.95 per marker. Although, none of the markers were present in all the accessions that were found to be highly drought tolerant with respect to any particular morph-physiological trait, some of the markers (RM252, RM331, RM432, RM36, RM525, RM260 and RM318) amplified alleles unique to nearly all the tolerant Nigerian landraces (IJS-02, IJS-09, IK-PS, IK-FS) and FARO-11, a drought tolerant control. These markers may be usefully exploited for molecular breeding of rice for drought tolerance.
Key words: Nigeria, climate change, rice, drought stress, drought tolerance, SSR markers, molecular breeding.
Rice is recognized as one of the most important staple food crop, accounting for more than half of human caloric intake globally. It is generally valued for its high nutritional benefits apart from being rich in calories, it is high in fibre, vitamins and minerals and low in cholesterol and sodium, suggesting it is a healthy source of energy. Asia is the largest producer and consumer of rice (Khush, 2005; Sellamuthu et al., 2011). In 2009, Nigeria was ranked 12th in the world’s list of rice-consuming countries, while it is ranked 17th globally, third in Africa and first in West Africa, as producers of rice (FAO, 2011). However, Nigerian rice production does not meet current demandor have the capacity to cope with an expanding population. Production is also suggested to be declining due to effects of climate change particularly through drought, heat, flooding and pests and diseases (Rosenzweig et al., 2000).
Drought is recognized as a major abiotic stress that limits rice productivity and adversely affects grain quality in rain-fed and upland ecosystems (Bimpong et al., 2011; Yang, 2008). Rice is most sensitive to drought stress during reproductive development at which time moderate water shortages can result in a significant reduction in grain yield (O’Toole, 1982; Venuprasad et al., 2008). The extent to which drought affects yield varies depending on the intensity and the time of occurrence of the stress within the crop growth cycle (Srividhya et al., 2011). Yield losses ranging from 15 to 50% have been reported (Pandey and Bhandari, 2009; Srividhya et al., 2011). The situation becomes more serious with increasing global climate change. Hence, the development of high-yielding and drought- tolerant varieties for rain-fed regions is a major goal of rice breeding.
Plant responses to drought are well known and believed to be complex involving numerous changes at the physiological, biochemical and molecular levels (
Atkinson and
Urwin, 2012, Bargaz et al., 2015). Tolerance to drought stress is therefore the result of expression of a number of traits over the stress time period. Thus, no single trait is likely to improve crop productivity, in response to water- deficits (Farooq et al., 2009; Kamoshita et al., 2008). Various traits associated with rice performance under drought stress, including root morphology, root penetrability and distribution, leaf rolling, reduced leaf area, early flowering and early seed maturity, osmotic adjustment (accumulation of compatible solutes such as proline and soluble sugars), and increased production of ABA and stomatal closure, have been reported (Bimpong et al., 2011; Price et al., 2000). Selection and use of these traits in breeding programmes could lead to sustainable production in drought prone regions (Nguyen et al., 1997). The wild species of rice, though phenotypically inferior in agronomic traits, are important reservoirs of many useful genes, especially genes for tolerance to major biotic and abiotic stresses, and can be used to improve the cultivated species for these desired traits through breeding (Ali et al., 2010; Sanchez et al., 2014). Genes from
O. glaberrima were used to develop NERICA lines with improved yield, earliness, weed competitive ability and tolerance to abiotic stresses, by interspecific hybridization with
O. sativa (Sanchez et al., 2014).
Complex responses to drought coupled with often unreliable and labour-intensive conventional phenotyping have made it difficult to breed rice varieties with improved drought tolerance (Ingram et al., 1994). To overcome this problem, molecular markers have been utilized to identify genotypes having traits directly related to drought tolerance and the strategy is already well developed and known to be more efficient than conventional variety improvement. Development of molecular markers and their use for the genetic dissection of agronomically-important traits has become a powerful approach for studying the inheritance of complex plant traits such as drought tolerance (Suji et al., 2011). The use of molecular markers for the selection of complex breeding traits offers greater selection accuracy with less labour and time inputs, and enables assemblage of different target traits into a single cultivar. Hence, use of molecular markers to detect QTLs controlling drought tolerance related traits has the potential to accelerate breeding for drought tolerance and will ultimately contribute to reducing the problem of food security aggravated by changing climatic conditions.
Substantial efforts have been made towards the identification of QTLs underlying traits associated with drought tolerance in rice chromosomes using molecular markers. Zheng et al. (2000) identified two QTLs for root penetration ability and root thickness that co-localizes with rice SSR markers RM252 on rice chromosome 4 and RM60 on chromosome 3. Rice QTLs for root growth rate and root penetration ability have also been mapped using RFLP and AFLP markers (Price et al., 2000; Price and Tomas, 1997). The co-location of QTLs for root traits with those of yield under drought, has allowed combined selection of both traits (Salunkhe et al., 2011). Vasant (2012) found 12 SSR markers that were strongly associated with root traits under drought and 14 SSR markers that were significantly associated with yield and its components under drought. Several other studies of molecular markers associated with drought related traits have also been reported in the literature, indicating that these markers could be usefully utilized in the molecular breeding of rice for improved drought tolerance.
The objectives of this study were to evaluate some of the SSR markers reportedly linked to drought tolerance traits in rice varieties and landraces cultivated in Nigeria as part of the development of a protocol for marker-assisted breeding for drought tolerance for sustainable rice production in the face of increasing climate change.
Plant
Thirty O. sativa accessions from different regions of Nigeria including a drought tolerant control and a susceptible control as well as some improved varieties were used (Table 1). Seeds of the local rice accessions selected based on popularity were obtained from farmers in the Nigeria States of Ebonyi, Enugu, Ekiti and Osun, while the improved varieties were obtained from the AfricaRice and the Alliance for Green Revolution in Africa (AGRA) through the Biotechnology Research and Development Centre, Ebonyi State University, Abakaliki, Nigeria.
Drought screening
Drought screening was conducted in a greenhouse of the Department of Botany, Obafemi Awolowo University, Ile-Ife, Nigeria where temperature ranged between 23 and 39°C during April and October, 2015. Rice seeds were germinated in plastic pots containing sandy-loam soil. At the 3-leaf stage corresponding to around 2-weeks after sowing, seedlings were transplanted (one plant per pot) into polyethylene bag growing pots measuring 30 × 20 cm with a volume of about 9,420 cm3. Drainage holes were provided at the bottom and the pots arranged in a completely randomized block design with ten replicates. The plants were irrigated for 45 days after sowing (DAS) by daily watering to slightly above soil saturation and thereafter five replicates each were assigned to one of two treatments – the control (well-watered) and those exposed to drought by withholding of water.
Adequate irrigation was maintained for the control treatment, while irrigation was withheld for 8 days in the drought stressed treatment during which the soil volumetric moisture content (SVMC measured using ASTM D-2216, 2014) declined from 19.5 ± 0.7 to 2.2 ± <0.1%.
The physical and chemical properties of the soil used are shown in Table 2. Compound fertilizer (NPK 15–15–15) was applied at the rate of 4.4 g pot-1 corresponding to 200 kg ha-1 in two applications (2 weeks after transplanting and at panicle initiation stage). The plants were kept weed-free throughout the period of the experiment by regular hand weeding. Irrigation was resumed on the drought-stressed group after the 8 days and continued till maturity at the same rate as that in the control treatment (Ndjiondjop et al., 2010).
Agro-botanical traits measurements
Data on plant height, leaf length and width, panicle length, number of primary branches, number of spikelet per panicle, spikelet fertility, panicle density, grain weight per plant and 1000 seed weight (adjusted to 14% moisture content) were collected using the procedures specified in Standard Evaluation System for Rice (SES) (IRRI, 1996) and those of Vasant (2012). After grain harvest, plants were harvested and the soil was washed off and the shoots and roots separated and wrapped in aluminium foil for oven drying at 80°C to a constant weight. Shoot and root dry weights were recorded and used to calculate root/shoot ratios (Vasant, 2012). Grain length and breadth were measured using Vernier callipers. Days to 50% booting and heading or flowering and days to maturity were also recorded using the SES procedures (IRRI, 1996).
Measurement of leaf water potential (Ψ)
Leaf water potential (LWP) was measured on the youngest fully developed leaf on the main tiller using WP4C Dewpoint psychrometer (Decagon Devices, Inc., USA) following the procedures used by Xiong et al. (2015).
Genotyping using SSR markers
Genomic DNA was extracted from two week old leaves of the rice accessions grown in greenhouse using Zymo Research plant/seed DNA extraction kit (Dixit et al., 2005). Twenty SSR primers were tested to find polymorphisms among the rice accessions. The SSR markers were chosen based on previous reports of their association with drought tolerant traits in rice (Vasant, 2012; Temnykh et al., 2001; Zheng et al., 2000). The list of SSR primers used for the study is shown in Table 3.
The PCR mixture composed of 2.0 µl of DNA template (50 ng 25 µl-1), 1.0 µl each of the forward and reverse primers (5 µM), 1.5 µl of MgCl2 (50 mM), 2.0 µl of 10 X Taq buffer, 0.4 µl of 2.5 mM dNTP mix, 1.0 µl DMSO (dimethyl sulfoxide), 0.1 µl of 5 units Taq DNA polymerase and made up to 25.0 µl with nuclease-free water. The PCR profile was 94°C for 2 min followed by 30 cycles of 94°C for 30s, 55°C for 45 s and 72°C for 45 s with a final extension at 72°C for 5 min. The amplification products were resolved on 8% denaturing polyacrylamide gel and the DNA fragments were revealed by silver staining and captured using a gel imager. The presence or absence of specific amplification bands were scored and used to determine number of alleles per primer and polymorphism information content (PIC) value of each microsatellite locus and to generate a dendrogram of the 30 rice accessions.
Statistical analysis
Analysis of variance (ANOVA) was performed using SAS software version 9.0, on the morpho-physiological trait values to compare the performances of the accessions under the imposed drought; percentage changes in the mean values of the traits due to stress or drought tolerance index (DI) and stress index (Is) based on a combination of traits values were also computed using the formulae shown subsequently and were used to rank the performances of the accessions under the drought; principal components analysis was also performed on the drought tolerance index values to determine traits that contributed most to the observed differences in drought performance among the rice accessions. Population genetic structure was determined using a dendrogram of the SSR data by the UPGMA method using Numerical Taxonomy System (NTSYSpc) version 2.02, while association analysis was carried out by physically comparing the allelic pattern of individual SSR markers and the pattern of phenotypic traits depressions. Number of alleles per primer and polymorphism information content (PIC) of each SSR locus were also recorded.
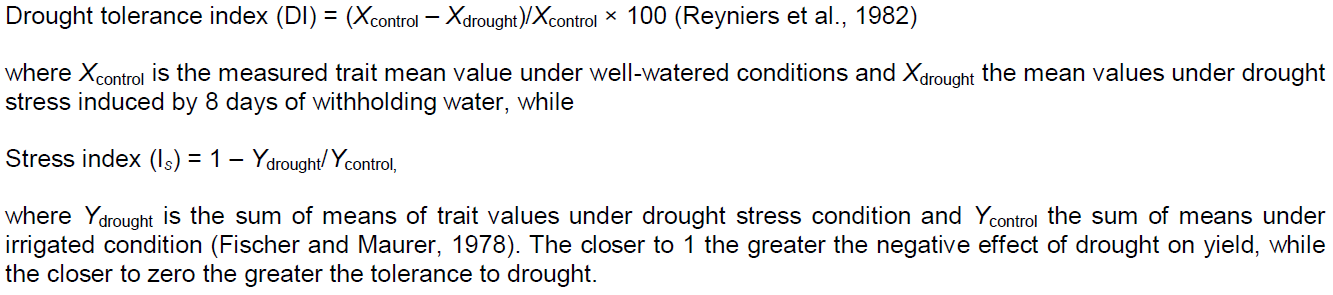
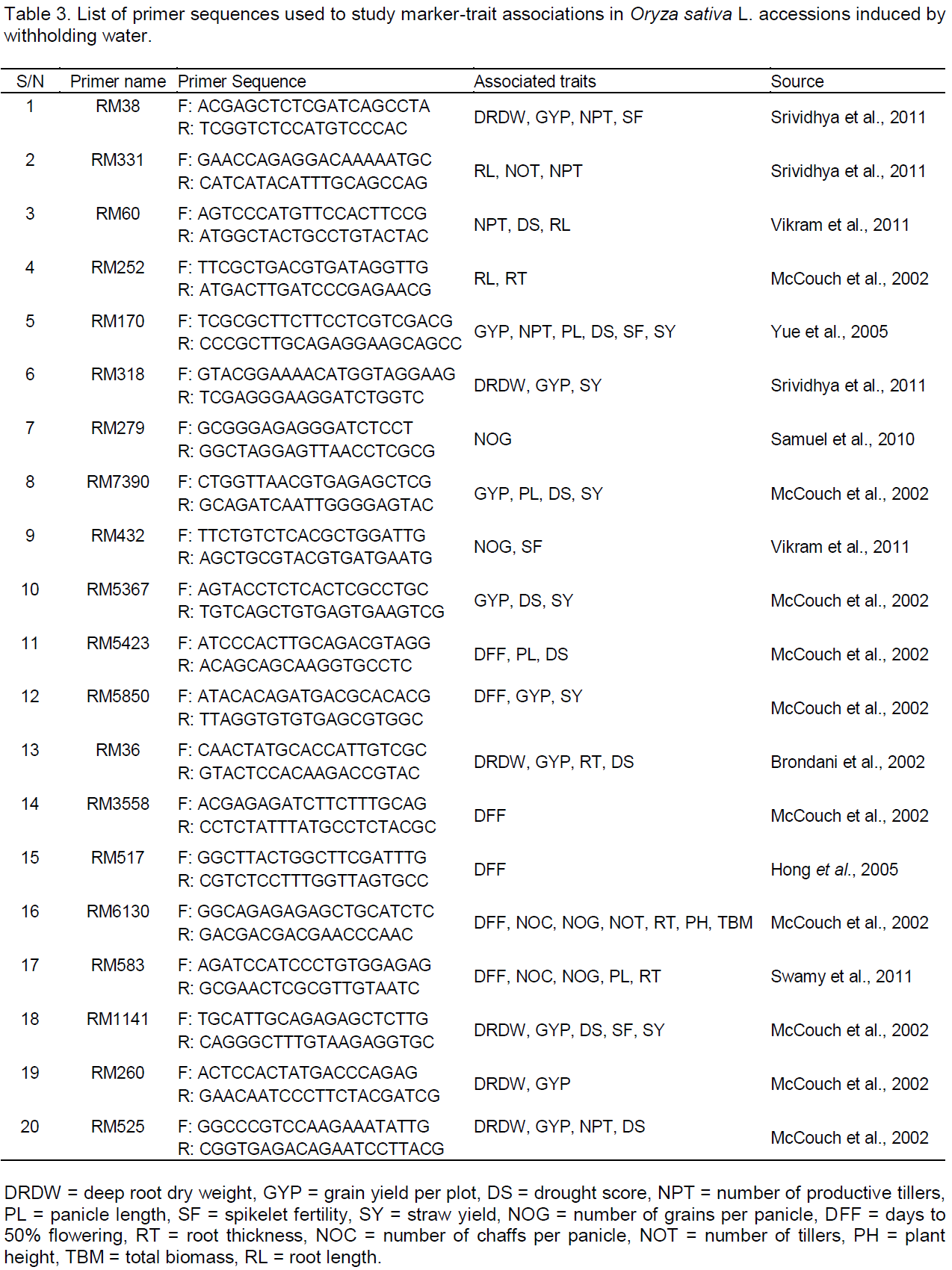
Effect of 8 days drought on the rice accessions
Water withholding for 8 days decreased SVMC from 19.5 to 2.2% (≈ 88.6% reduction) and Figure 1 is a photo showing the effect on the rice plants. Almost all the measured growth, yield and physiological parameters were significantly affected and the accessions responded differently to the drought treatment (Tables 4).
For growth and yield, only 2 of the rice accessions (AGW-PS and UPIA-1) did not show reductions in plant heights whereas around 65% showed significant (P<0.05) height reduction between 4.5 and 23.4%, with the largest reduction observed in AGW-55 and FARO-11. Around 44% of the accessions had significant reductions in leaf length (LL) on the main tillers between 6.6 and 23.1% with the largest reduction occurring in UPIA-1, IFW-13 and AGW-PS, while IWA-10, NERI-34, FARO-44, IR-119, FARO-19, UPIA-2, IK-PS, and IJS-02 were not affected. 61% of the accessions showed significant reductions in leaf width (LW) between 8 and 29% with, AGW-PS and IR-119 having the largest reductions but some accessions such as FARO-19, NERI-34 and FARO-11 were not affected. Panicle length (PL) was significantly reduced from 5 to 28% in 56% of the accessions, IHEK and FARO-19 showed the largest reductions. Sixty one percent of the accessions showed significant reductions of between 5.6 and 32% in the number of primary branches per panicle (NPBPP) on the main tillers, the largest effect was on AGW-PS, IFW-07 and FARO-11, while 26% of the accessions including FARO-19, IJS-09, Lad-f, NERI-34 and FARO-44 did not show any effect. The drought treatment significantly reduced the number of spikelet per panicle on the main tillers in 78% of the accessions, but had no effect on FARO-44. Reduction ranged from 1.9 to 40.1%. IJS-02 and IR-119 exhibited the lowest reductions while IHEK, IWA-10, IFW-07 and IFW-13 had the largest reductions. Reductions in spikelet fertility (SF) between 3.7 and 20.4% were observed in 70% of the accessions. The largest reduction occurred in IR-119 and NERI-34 whereas in IWA-10, AGW-PS and FARO-19 there were no effects. Panicle density (PD) was depressed in all the accessions, ranging from 5.8 to 37.9% except in FARO-44, FARO-19, NERI-34 and IK-FS. Reductions were significant (P<0.05) in 70% of the accessions from 9.5 to 37.9% in PD. PD was most depressed in IWA-10, FARO-57, IFW-13 and IFW-07. With the exception of IJS-02, seed weight per plant (SWPP) decreased in all the accessions with the reduction ranging from 0.4% in IJS-09 to 43.7% in IHEK, reductions (9.4 to 44%) were significant in 78% of the accessions. The reduction in 1000 seed weight (1000SW) varied from 0.8% in IFW-13 to 14.7% in IHEK, while there was no reduction for FARO-19. Shoot dry weight (SDW) decreased significantly in 78% of the accessions, with reductions ranging from 10.5% in IR-119 to 45.7% in UPIA-2, but no reduction was observed with Lad-f and IWA-8. Root dry weight (RDW) depression occurred in almost all accessions varying from 2.3% in IWA-10 to 43.6% in AGWU-116, reductions were significant in 56.5% of the accessions (7 to 43.6%), the exceptions were IJS-09, FARO-44, AGW-PS, Lad-f and IWA-8. Root shoot dry weight ratio was significantly depressed (from 1.5 to 26.8%) in about 39% of the accessions. Significant increases in RSDWR of around 6.8 to 76% were apparent in many accessions (47%), but there were no effects in 13% of the accessions. The greatest reduction was seen with Lad-f, FARO-57 and FARO-19 while the largest increase was with AGW-PS, AGW-55, FARO-44, IJS-09, IWA-10 and UPIA-1. The effect of drought on grain lengths and widths showed that only about 30% of the accessions had significant reductions (p<0.05) in grain lengths between 3 and 9.4%, while significant reductions in grain width between 5.9 and 11.3% occurred in 22% of the accessions. The most reduced growth and yield traits were shoot dry weight, seed weight per plant and root dry weight.
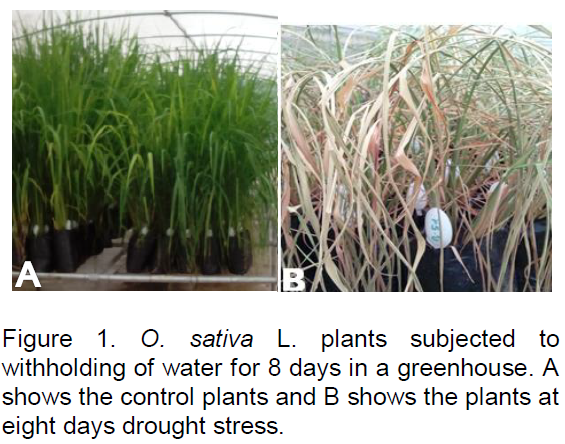
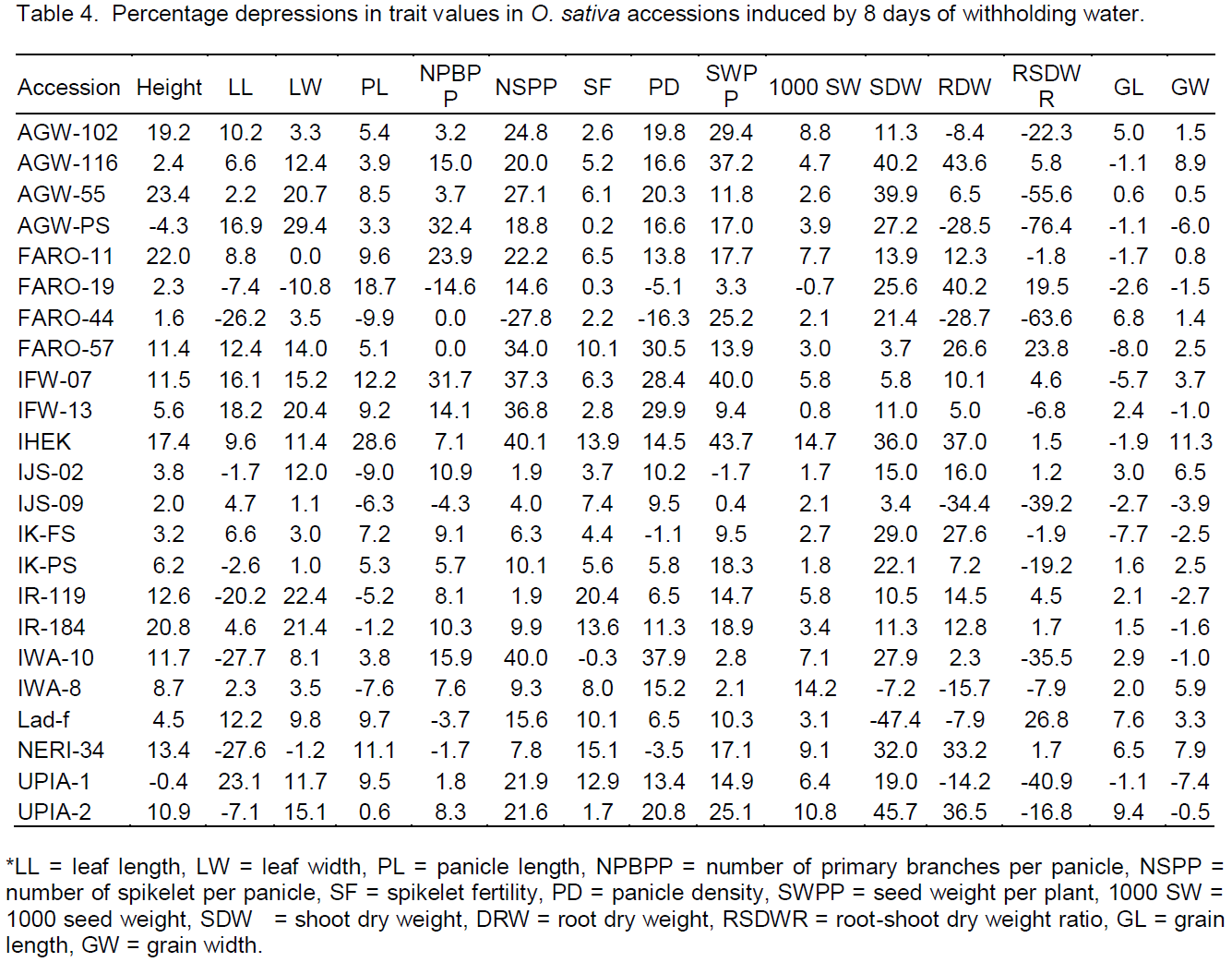
The effects of withholding water, on all of the traits, were combined in Stress Index (Is) for each accession and the values were used to rank the accessions in order of drought tolerance. Based on this, FARO-44, the Nigerian landraces (Lad-f, IJS-02, IJS-09, IK-FS and IK-PS) and IR-119 showed lower depressions in growth and yield traits due to withholding water, while IHEK and IFW-07 exhibited the largest growth depressions (Figure 2).
For delays, booting, flowering and maturity dates were significantly delayed in almost all accessions. Delays ranged from 0 to 21 days for days to 50% booting, 1 to 21 days for days to 50% flowering and 1 to 22 days for days to maturity were observed (Table 5). Accessions IK-FS, FARO-44, IK-PS and IHEK delayed the least, while FARO-11 followed by IFW-13 and FARO-19 had the longest delays to booting and flowering dates. Stress index based on earliness traits indicated that IK-PS, FARO-44 and IK-FS were more drought tolerant while FARO-11 followed by IFW-13 and FARO-19 exhibited higher sensitivity to drought (Figure 3).
For leaf water status, leaf water potential (LWP) was the most significant drought affected metric (Table 6). Reduction ranged from 145 to >4,000%. The Nigerian landraces (IJS-09, IJS-02, IK-FS and IK-PS) and the landraces IJS-09, IJS-02, IK-FS followed by IR-119, IK-PS and FARO-44 to have lower reductions in LWP, while accessions AGW-102, AGW-PS and AGW-116 were reduced the most (Figure 4).
Principal components analysis of trait depression values
The percentage depressions in trait values were subjected to principal components analysis to determine those most responsible for the observed differences in the accessions responses to withholding water. Eight (8) components were used but only 4 were significant (Table7) and explained about 62% of the total variations. The result of the PCA indicates that reductions in NSPP, PD and PL, as well as, the delay in maturation (MD) provided the greatest contributions to the observed differences in the performance of the accessions under drought. The second set of agro-morphological traits whose reductions contributed noticeably to the observed drought response differences among the accessions include grain weight, root dry weight, root-shoot dry weight ratio and spikelet fertility; the third set of traits were 50% BD, 50%HD, number of primary branches per panicle, leaf width and seed weight per plant; whereas the last set of traits were grain length, shoot dry weight, leaf length and leaf water potential.
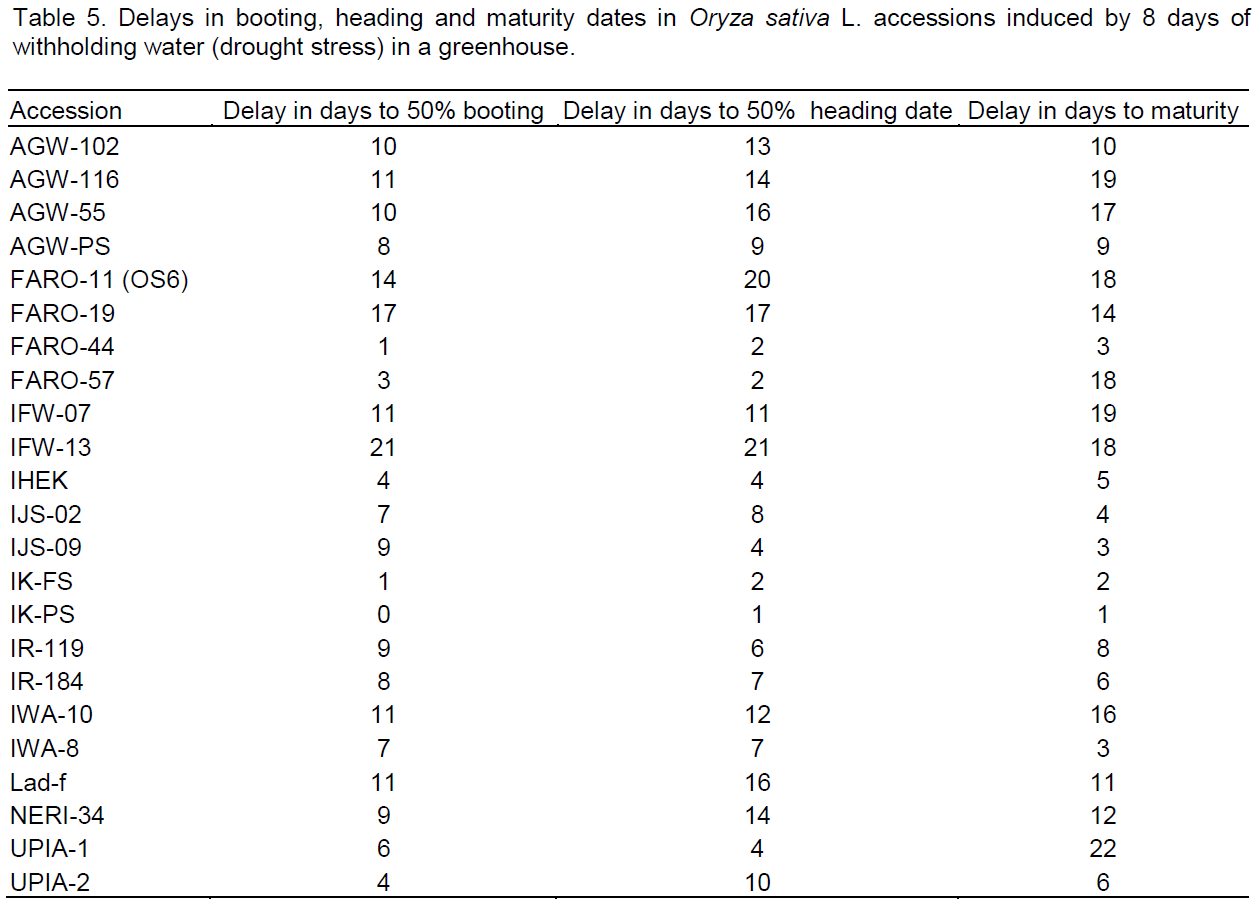
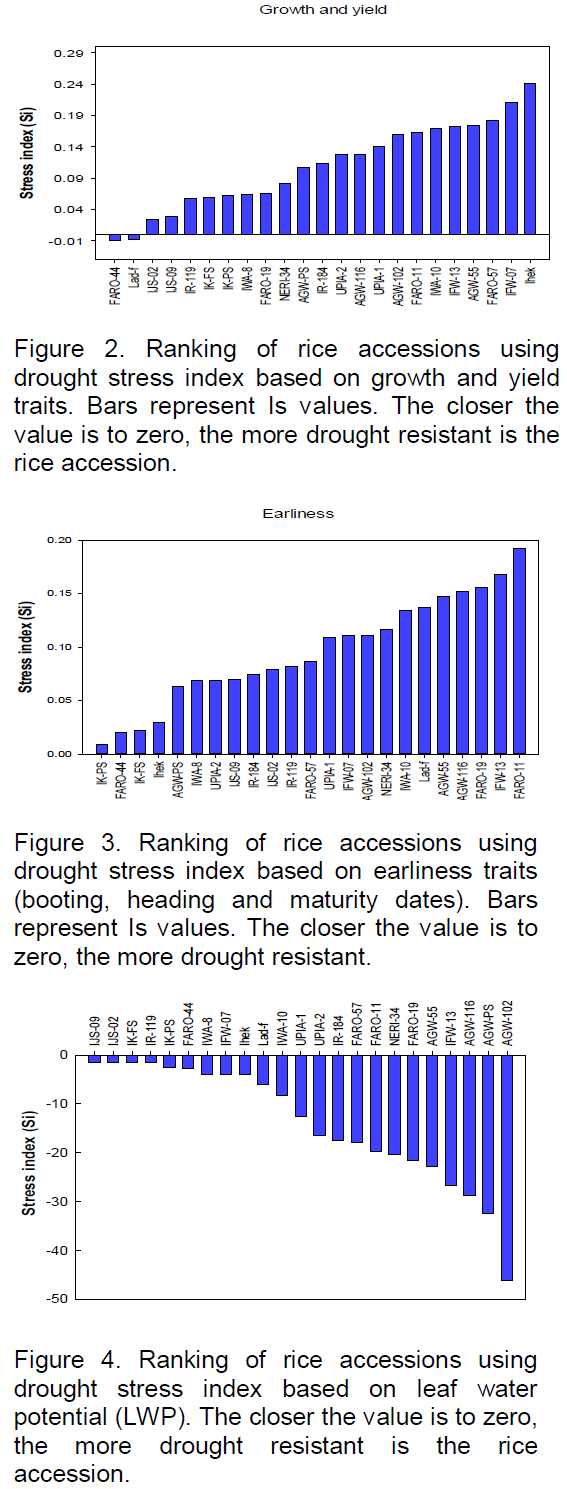
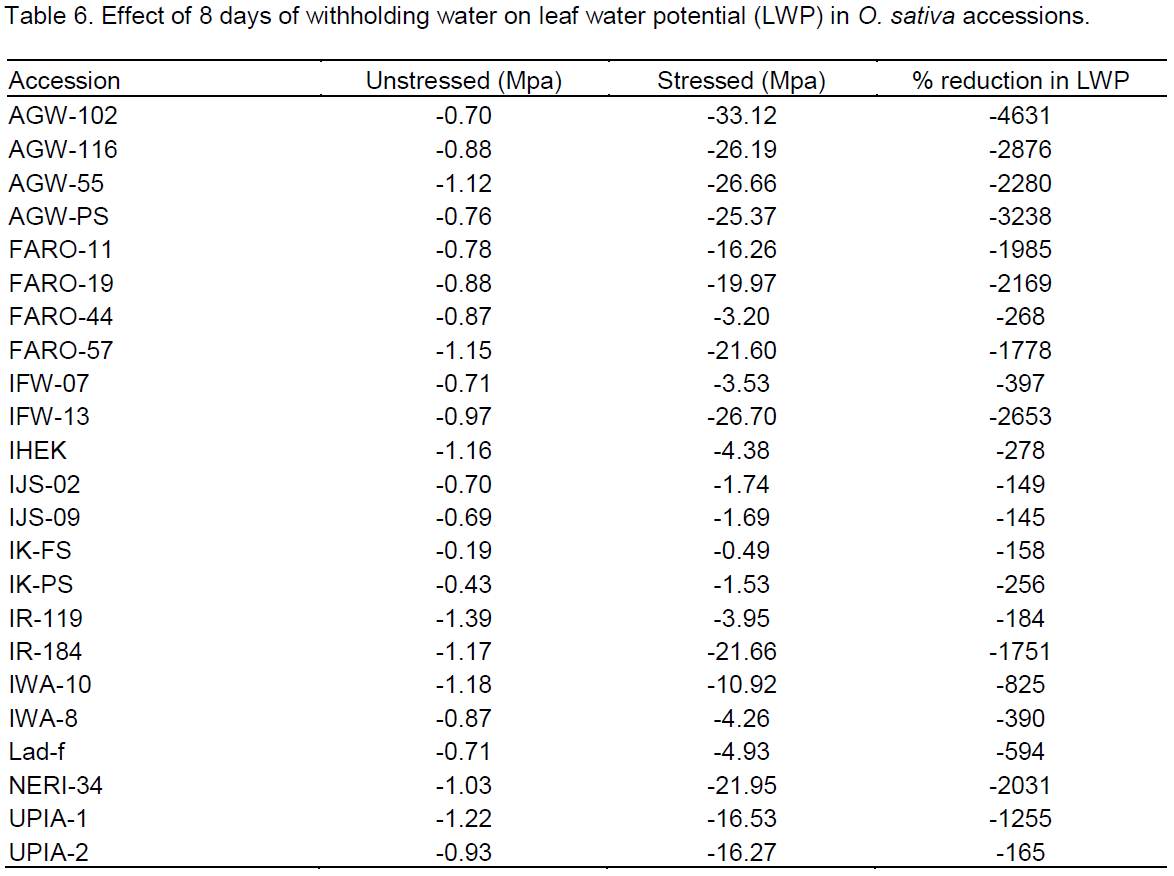
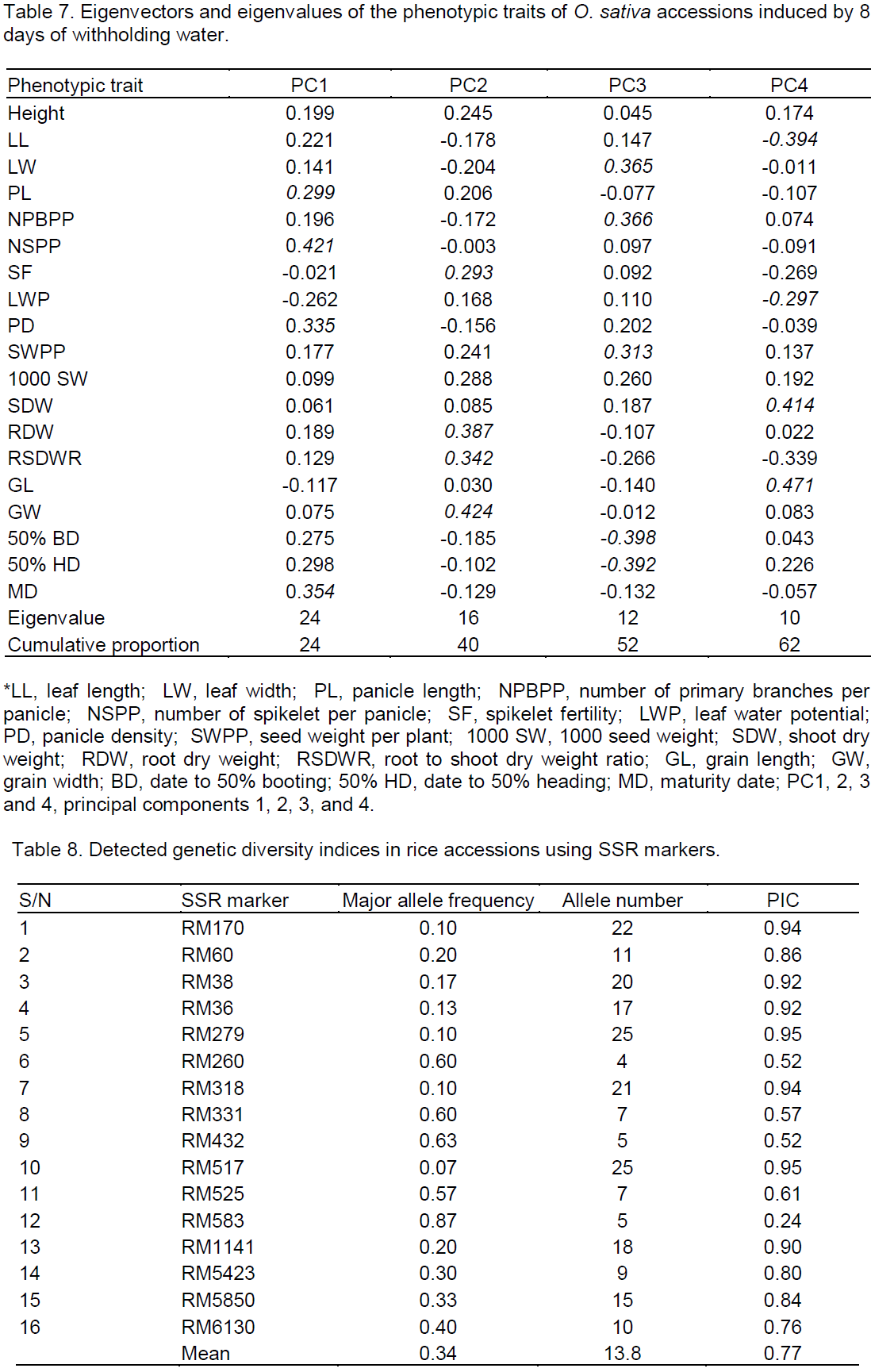
SSR polymorphism and population structure of the accessions
To access the level of genetic diversity in the population studied, a total of 20 SSR primers were used to study DNA polymorphism among the rice accessions. Table 8 shows the major allele frequency, number of alleles and PIC of each of the microsatellite loci in the studied accessions. Of the 20 primers, 16 produced scorable amplification bands used in the analysis, while 4 primers failed to amplify any of the rice DNA. The 16 SSR primers amplified a total of 221 alleles. Number of alleles per primer ranged from 4 to 25 with a mean of 13.8 while the PIC values spanned from 0.24 to 0.95 with an average value of 0.77.
A dendrogram of the 30 rice accessions using UPGMA procedure clustered the accessions into 6 major groups almost in accordance with their source locations (Figure 5). Nwad, a landrace from Ebonyi State, formed a distinct group (Group 1) suggesting that it is distantly related from the rest of the accessions. Group 2 included all accessions from Ebonyi State with only 2 accessions (Nwad and Lad-f) falling outside this group. Group 3 was a large cluster with distinct sub-groups. Accessions from Enugu State (AGW-PS, AGW-116 and AGW-102) are clustered together with only 1 (AGW-55) outside the sub-group but still showing a significant relationship. Accessions from Ife in Osun State (IFW-55, IFW-07 and IFW-13) are clustered together; the FARO lines (FARO-19, FARO-44 and FARO-57) except FARO-11 are grouped together while the improved varieties (UPIA-2, NERI-34, IR-119 and IR-184) are clustered together. Group 4 contained the single accession (Lad-f) also from Ebonyi State. Group 5 was comprised of accessions from Ekiti State (IJS-02, IJS-09, IK-PS and IK-FS) and FARO-11, whereas group 6 was another cluster of improved varieties including IWA-8, IWA-10 and UPIA-1).
Analysis of marker-trait association under drought-stress
The pattern in which each of the SSR markers clustered the accessions was compared with the pattern of individual trait depression due to the drought. Our result show that none of the markers clearly grouped the accessions according to the pattern of trait depression but few of the markers amplified alleles common only to accessions IJS-02, IJS-09, IK-PS, IK-FS and FARO-11. With the exception of FARO-11, these accessions are among the first five accessions that exhibited lower depressions in LWP (Figure 4) and also among the first seven accessions that showed lower drought depressions in overall growth and yield traits (Figure 2). Furthermore, they are among the first ten accessions that exhibited the least delay in flowering and maturation. FARO-11(OS6) is a known drought tolerant cultivar and is used here as a drought tolerant control. RM252 amplified about 100 bp fragment, RM331 amplified about 80 bp, RM432 amplified about 90 bp, RM36 produced about 80 bp, RM525 produced about 70, 72 and 75 bp fragments in these accessions (IJS-02, IJS-09, IK-PS, IK-FS and FARO-11), whereas RM260 amplified as short 30 bp fragment in the accessions including ARUB. RM318 amplified about 70 bp fragment in the landraces IJS-02, IJS-09, IK-PS, IK-FS and ARUB but not in FARO-11 (Figure 6).
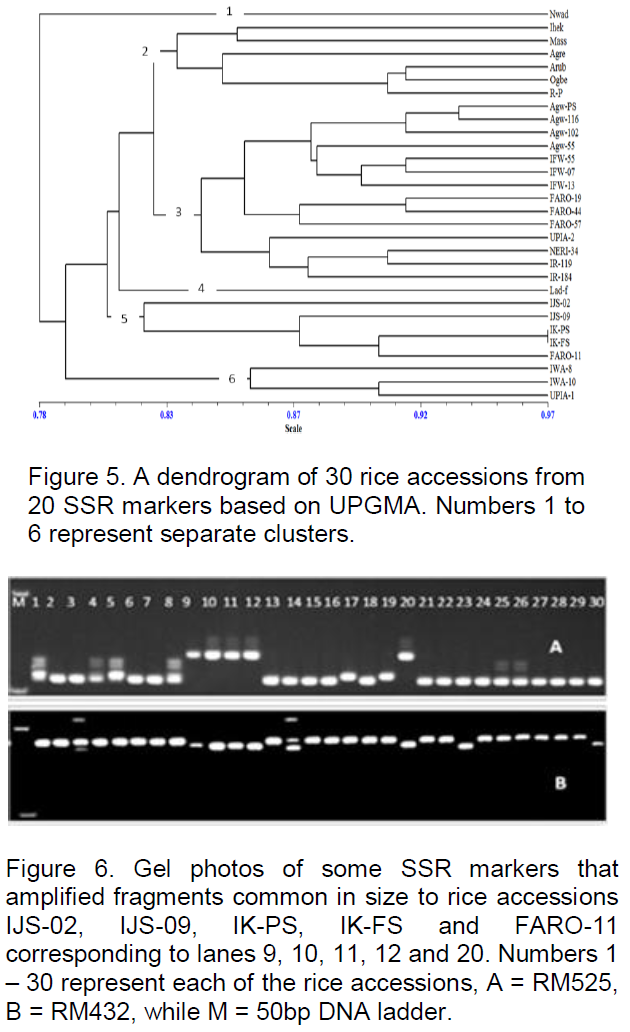
Screening of rice for growth and yield performance under drought stress
Drought tolerance generally denotes the ability of a crop plant to survive, grow and yield satisfactorily under water-limited conditions (Fleury et al., 2010; Turner, 1979). In this study, some rice varieties cultivated in Nigeria, including landraces and improved varieties, were screened in the greenhouse for their growth and yield performance under complete withholding of water for 8 days when at a late vegetative stage in their development (45 DAS). A number of vegetative and reproductive traits were used to characterize the accessions under drought. The rice accessions exhibited large differences in their responses to withholding water. Although the duration of withholding of water was short, the adverse effects (Figure 1) were actually severe probably due to the low water retention capacity of the soil used (74% sand; Table 2), with SVMC declining by 88% of field capacity and drought stress developing more rapidly due to the soil’s low water holding capacity. A SVMC of 2% measured in a sandy loam soil, means that all the available moisture would have been used with the soil at or close to the permanent wilting point (Kramer, 1969). However, it is suitable to assess tolerance to drought within a short period of the stress since genotypic differentiation of leaf elongation rates is greatest under moderate short stress (Cal et al., 2013). Leaf elongation rate is a useful indicator of drought tolerance and is closely correlated with a subsequent loss of yield due to drought. Under severe drought, leaf differences in elongation rate are very small between sensitive and tolerant cultivars. Serraj and Sinclair (2002) asserted that comparison of genotypic growth responses to drought is more appropriate under short and mild stress before the drought survival phase become apparent. The observation of physiological responses (reduction in LWP) implies that biochemical and molecular changes occurred in the rice accessions under the mild drought stress. It is also very relevant to the study environment where rice growing season in much of Nigeria is characterized by periods of short droughts rather than terminal drought.
It was observed that no single accession showed either the greatest or least depressions in all the traits measured. For instance, AGW-PS followed by UPIA-1, FARO-44 and IJS-09 were the most drought tolerant accessions based on reductions in plant height, whereas FARO-44, IJS-02, IWA-8 and IJS-09, respectively, were the most tolerant using panicle length depression. Similarly, FARO-44 followed by IJS-02, IR-119, IJS-09 and IK-FS were the most tolerant in terms of depression in spikelet number on the main panicle while IJS-02 followed by IJS-09, IWA-8 and IWA-10 was the most drought tolerant in terms of grain yield per plant (SWPP) (Table 4). This type of response can be linked to the complex nature of drought tolerance involving mechanistic interactions between an array of morphological, physiological, biochemical and genes and their expression (Li and Xu, 2007; Mitra, 2001; Price et al., 2002) and the differential responses of different rice accessions to drought (Vasant, 2012). It also suggests that tolerant plants have various pathways of exercising trade-offs to ensure survival.
To unambiguously rank the rice accessions based on their overall growth and yield performance under the imposed water withholding conditions and to select the most drought tolerant accessions, a stress index (Is) was used which relies on a combination of trait values under withholding water and well-watered (Fischer and Maurer 1978). Based on this procedure, FARO-44, Lad-f, IJS-02, IJS-09, IR-119, IK-FS and IK-PS, in decreasing order, were the most tolerant of the 23 accessions screened in relation to growth and yield, while IHEK followed by IFW-07, FARO-57, AGW-55, IFW-13 and IWA-10 were the most susceptible. It is worth noting that 5 of the 7 most tolerant accessions here are Nigerian landraces (Lad-f, IJS-02, IJS-09, IK-FS and IK-PS). These accessions, especially IJS-02, IJS-09, IK-FS and IK-PS, were found to be the earliest maturing of the 23 accessions (91 to 104 days; data not shown), which is an important late season drought avoidance strategy (Araus et al., 2002; Jongdee et al., 2002). These landraces also recorded the highest 1000 seed weight (39 to 42 g). However, they have a very low tiller number (3 to 5) which requires improvement to fully exploit their drought avoidance potential. Alternatively, increasing the sowing density of these accessions may adequately compensate for the lower tiller number, considering their high seed quality (1000 seed weight). Furthermore, planting them in this way may not lead to an unacceptable level of competition for photosynthetically-active radiation, but would amount to effective utilization of space and soil resources, since the accessions are not of an ‘open plant’ type. These accessions can be promising breeding material for improvement of higher yielding genotypes for enhanced drought tolerance in Nigeria and other similar situations and locations. However these genotypes have been somewhat neglected, by farmers, owing to their low tillering and yields, but as landraces, they appear better adapted to the Nigerian environment and are potential reservoirs of adaptability genes including those for drought and other abiotic stress tolerance (Villa et al., 2005; Friis-Hansen and Sthapit, 2000). Furthermore, these landraces performed better than FARO-11 which was used here as drought tolerant control (Ubi et al., 2011), while sharing several similar phenotypic features such as tiller number, height, culm morphology, grain shape and size with FARO-11. Principal components analysis revealed depressions in panicle lengths, number of grains per panicle, panicle density and delays in maturity date as the most important traits determining variations in rice performance under drought.
Screening for ability to maintain leaf water status
Analysis based on LWP also indicated that same landraces (IJS-09, IJS-02, IK-FS and IK-PS) among the 5 most drought tolerant accessions by their ability to maintain higher LWP under water withholding conditions. This suggests that these accessions may be using drought avoidance mechanism to cope with the stress of a water shortage. Drought tolerance is frequently apparent as increased capacity to maintain a higher LWP relative to a reduction in SVMC (Fukai et al., 1999; Kato et al., 2001, 2006; Mitra, 2001). By so doing they are able to extract water from the soil as its water potential falls thereby minimizing the yield losses (Singh et al., 2012). Of the 23 accessions screened, these accessions (IJS-09, IJS-02, IK-FS and IK-PS) also maintained the highest root to shoot ratio (0.23 to 0.28; data not shown) which can enhance root soil exploitation to extract more of the available soil moisture to maintain root and leaf tissue turgor and therefore growth under drought (Blum et al., 1989; Samson et al., 2002; Wang et al., 2006).
Screening for SSR polymorphism and their association with phenotypic drought traits
Genetic improvement of rice for drought tolerance through conventional breeding is slow due to the spatial and seasonal variations in drought timing and severity, the complex nature of drought tolerance itself and the difficulty in selecting for combinations of traits which best suit combating drought induced yield reductions (Courtois et al., 2003; Khush, 2001). Among the factors accounting for the slow progress in developing drought tolerant rice is the low heritability, multiple gene control, epistatic gene interaction, high incidence of genotype x environment interactions, etc. which could seriously influence ‘actual’ yields (Atlin and Lafitte, 2002; Cattivelli et al., 2008). The use of molecular markers to select accessions possessing genes and genomic regions that control target traits can fast-track the progress in breeding for drought tolerant rice, because molecular markers are transmitted faithfully from generation to generation and are not subject to environmental influences (Crouch and Ortiz, 2004; Gupta et al., 1999; Korzun et al., 2001; Senior et al., 1998). SSRs are a DNA marker system of choice for genetic analysis in rice because of their abundance in the rice genome, high level of polymorphism and high but simple reproducible assays involved (Powell et al., 1996; Singh et al., 2010). The 16 SSR primers used here generated 4 to 25 alleles per primer with PIC values ranging from 0.24 to 0.95. As high as 11 out of the 16 markers (≈69%) produced PIC values between 0.76 and 0.95 reflecting the high discriminating powers of the markers used.
The patterns of accession clustering of individual SSR markers when compared with that of the individual trait depressions caused by drought, was used to determine marker-trait associations for drought tolerance. Although none of the markers typically clustered the accessions absolutely in accordance with the pattern of trait depressions, some of the markers (RM252, RM331, RM432, RM36, RM525, RM260 and RM318) each amplified alleles unique to accessions IJS-02, IJS-09, IK-PS, IK-FS and FARO-11. Four of these accessions (IJS-02, IJS-09, IK-PS and IK-FS) are landraces from Ekiti State and are among the most drought tolerant accessions found here based on their capacity to maintain LWP, and grow and yield satisfactorily under the imposed drought.
Dendrogram analysis provided supporting evidence of which plant characteristics facilitate drought tolerance and how these characteristics are linked to apparent geographical origin, but equally important, it has capacity to illuminate linkages between trait groupings and functional aspects of tolerance. Here rice accessions in group 5 of the dendrogram contains all the drought tolerance accession with respect to little fall in leaf water potential, and most of these show little change in leaf length reductions. One of the first and well described responses to drought is a reduction in leaf growth (Cutler et al., 1980). Reductions in leaf area, particularly during canopy establishment can reduce yield (Cal et al., 2013). Here we see the greatest reduction in leaf length with UPIA-1, IFW-13 and AGW-PS. Conversely, there was little effect of drought on leaf length for IWA-10, NERI-34, FARO-44, FARO-19, UPIA-2, IR-119, IK-PS and IJS-02. The latter two accessions are in Group 5, where leaf water potentials showed the least reduction in response to drought of all the accessions. The accession AGW-PS also showed the largest reduction in leaf width and is in Group 3, where accessions lie which show the greatest decline in leaf water potential on exposure to drought. AGW-PS however does show the least change in height growth and root growth. The most likely explanation for this apparent paradox is that by maintaining root growth, maximal access and uptake of water can be achieved. This accession is able to utilise much of the available water to grow in the absence of conservative drought responses, such as stomatal closure which restricts water use and maintains leaf water potential (as with isohydric species). Reports suggest that rice under severe drought shows anisohydric behaviour (Boonjung and Fukai, 1996; Jongdee et al., 2002; Sibounheuang et al., 2006) keeping their stomata open and photosynthetic rates high for longer periods, even in the presence of decreasing leaf water potential. Root growth was also maintained in IJS-09 and FARO-44, Groups 5 and 3 accessions respectively, where limited drought induced little reductions in leaf water potential, while the opposite was true for AGWU-116. Comparative experiments, else-where, using deeper rooting rice Dro1-NIL, suggest that drought avoidance is achieved through root access to water deeper in the soil profile (Arai-Sanoh et al., 2014). Root growth potential has been the focus of trait exploration for rice drought avoidance (Price et al., 2002; Uphoff et al., 2015). Parent et al. (2010) observed lower sensitivity of drought in upland rice than lowland genotypes and suggested that drought sensitivity in rice maybe due to poor root system growth. FARO-44 and IJS-02, in groups 3 and 5 respectively, show little response to drought, results here strongly support the idea that root growth and water uptake provide a means of drought avoidance, at least for intermittent drought stress.
The genetic dendrogram was highly effective in reflecting the source locations of the accessions. The accessions from Ebonyi State (IHEK, Mass, AGRE, Arub, Ogbe and R-P) were grouped almost entirely together with only 2 accessions (Nwad and Lad-f) outside the cluster. This analysis also separately grouped accessions from Enugu State (AGW-PS, AGW-116,AGW-102 and AGW-55) and accessions from Osun State (IFW-55, IFW-07 and IFW-13) and showed that these 2 groups were closely related, which was supported by their poor performances under water withholding. The dendrogram also revealed a close linkage of the Enugu (AGW-) and Osun (IFW-) accessions with the FARO lines except FARO-11. It was noted that all accessions from Ekiti State (IJS-02, IJS-09, IK-PS and IK-FS) were grouped with FARO-11 and that all members of this group exhibited highly similar phenotypic features, but the landraces were much more drought tolerant than FARO-11 (a drought tolerant control). The improved varieties other than the FARO lines (UPIA-2, NERI-34, IR-119, IR-184, IWA-8, IWA-10 and UPIA-1) were clustered into two separate groups. It can be deduced from this study that the markers were able to partition the accessions in line with their source locations reflecting the robustness of SSR markers to dissect the population genetic structure and demographic history of domestication (Akkaya et al., 1992; Cho et al., 2000; Garris et al., 2005).
It is important to note that though FARO-11(OS6) is a known drought tolerant cultivar (Ubi et al., 2011) and used as a drought tolerance validation, it did not really perform in this study as expected.
The authors have not declared any conflict of interest.
This research is supported by funding from the Department for International Development (DfID) under the Climate Impact Research Capacity and Leadership Enhancement (CIRCLE) programme.
REFERENCES
Akkaya MS, Bhagwat AA, Cregan PB (1992). Length polymorphisms of simple sequence repeat DNA in soybean. Genet. 132:1131-1139.
|
|
Ali ML, Sanchez PL, Yu S, Lorieux M, Eizenga GC (2010). Chromosome segment substitution lines: a powerful tool for the introgression of valuable genes from Oryza wild species into cultivated rice (O. sativa L.). Rice 3:218-234.
Crossref
|
|
|
Arai-Sanoh Y, Takai T, Yoshinaga S, Nakano H, Kojima M, Sakakibara H, Kondo M, Uga Y (2014). Deep rooting conferred by DEEPER ROOTING 1 enhances rice yield in paddy fields. Scientific Reports 4. Article Number: 5553.
Crossref
|
|
|
Araus JL, Slafer GA, Reynolds MP, Royo C (2002). Plant breeding and drought in C3 cereals: what should we breed for? Ann. Bot. 89:925-940.
Crossref
|
|
|
Atkinson NJ, Urwin PE (2012). The interaction of plant biotic and abiotic stresses: from genes to the field. J. Exp. Bot. 63:3523-3543.
Crossref
|
|
|
Atlin GN, Lafitte HR (2002). Marker-assisted breeding versus direct selection for drought tolerance in rice. In: Saxena N, P O'Toole JC (eds.). Field Screening for Drought Tolerance in Crop Plants with Emphasis on Rice. Proceedings of an International Workshop on Field Screening for Drought Tolerance in Rice, 11 – 14 December 2000, International Crop Research Institute for the Semi-Arid Tropics, 2002.
|
|
|
Bargaz A, Zaman-Allah M, Farissi M, Lazali M, Drevon J-J, Maougal RT, Georg C (2015). Physiological and molecular aspects of tolerance to environmental constraints in grain and forage legumes. Int. J. Mol. Sci. 16:18976-19008.
Crossref
|
|
|
Bimpong IK, Serraj R, Chin JH, Ramos J, Mendoza E, Hernandez J, Mendioro MS, Brar DS (2011). Identification of QTLs for drought-related traits in alien introgression lines derived from crosses of rice (Oryza sativa cv. IR64) × O. glaberrima under lowland moisture stress. J. Plant Biol. 54:237-250.
Crossref
|
|
|
Blum A, Shpiler L, Golan G, Mayer J (1989). Yield stability and canopy temperature of wheat genotypes under drought- stress. Field Crops Res. 22:289-296.
Crossref
|
|
|
Boonjung H, Fukai S (1996). Effects of soil water deficit at different growth stages on rice growth and yield under upland conditions. 1. Growth during drought. Field Crops Res. 48:37-45.
Crossref
|
|
|
Brondani C, Rangel N, Brondani V, Ferreira E (2002). QTL mapping and introgression of yield-related traits from Oryza glumaepatula to cultivated rice (Oryza sativa) using microsatellite markers. Theor. Appl. Genet. 104:1192-1203.
Crossref
|
|
|
Cal AJ, Liu D, Mauleon R, Hsing Y-lC, Serraj R (2013). Transcriptome profiling of leaf elongation zone under drought in contrasting rice cultivars. PLOS One: 8(1):54537.
Crossref
|
|
|
Cattivelli L, Rizza F, Badeck FW, Mazzucotelli E, Mastrangello AM, Francia E, Mare C, Tondelli A, Stanca M (2008). Drought tolerance improvement in crop plants: An integrated view from breeding to genomics. Field Crops Res. 105:1-14.
Crossref
|
|
|
Cho YG, Ishii T, Temnykh S, Chen X, Lipovich L, McCouch SR, Park WD, Ayres N, Cartinhour S (2000). Diversity of microsatellites derived from genomic libraries and GenBank sequences in rice (Oryza sativa L.). Theor. Appl. Genet. 100:713-722.
Crossref
|
|
|
Courtois B, Shen L, Petalcorin W, Carandang S, Mauleon R, Li Z (2003). Locating QTLs controlling constitutive root traits in the rice population IAC 165xCo39. Euphytica 134:335-345.
Crossref
|
|
|
Crouch JH, Ortiz R (2004). Applied genomics in the improvement of crops grown in Africa. Afr. J. Biotechnol. 3:489-496.
Crossref
|
|
|
Cutler JM, Shahan KW, Steponkus PL (1980). Influence of water deficits and osmotic adjustment on leaf elongation in rice. Crop Sci. 20:314-318.
Crossref
|
|
|
Dixit A, Jin M, Chung J, Yu J, Chung H, Ma K, Park Y, Cho E (2005). Development of polymorphic microsatellites markers in sesame (Sesamum indicum L.). Mol. Ecol. Notes 5:736-739.
Crossref
|
|
|
FAO (2011). Http://faostat.fao.org/.
|
|
|
Farooq M, Kobayashi N, Wahid A, Ito O, Basra SMA (2009). Strategies for producing more rice with less water. Adv. Agron. 101:351-388.
Crossref
|
|
|
Fischer RA, Maurer R (1978). Drought resistance in spring wheat cultivar: I- Grain yield response. Aust. J. Agric. Res. 29:897-912.
Crossref
|
|
|
Fleury D, Jefferies S, Kuchel H, Langridge P (2010). Genetic and genomic tools to improve drought tolerance in wheat. J. Exp. Bot. 61:3211-3222.
Crossref
|
|
|
Friis-Hansen E, Sthapit B (2000). Participatory approaches to the conservation of plant genetic resources. Int. Plant Genet. Resour. Institute, Italy, Rome, P 199.
|
|
|
Fukai S, Pantuwan G, Jongdee B, Cooper M (1999). Screening for drought resistance in rainfed lowland rice. Field Crops Res. 64:61-74.
Crossref
|
|
|
Garris A, Tai TH, Coburn J, Kresovich S, McCouch S (2005). Genetic structure and diversity in Oryza sativa L. Genet. 169:1631-1638.
Crossref
|
|
|
Gupta PK, Varshney RK, Sharm PC, Ramesh B (1999). Molecular markers and their applications in wheat breeding. Plant Breed. 118:369-390.
Crossref
|
|
|
Hong L, McCouch SR, Rutger JN, Coburn JR, Tai TH, Redus MA (2005). Population structure and breeding patterns of 145 US rice cultivars based on SSR marker analysis. Crop Sci. 45:66-76.
Crossref
|
|
|
Ingram KT, Bueno FD, Namuco OS, Yambao EB, Beyrouty CA (1994). Rice root traits for drought resistance and their genetic variation. In: Kirk GJD (ed) Rice Roots: Nutrient and Water Use. Selected papers from the International Rice Research Conference. International Rice Research Institute, Manila, Philippines, pp. 67-77.
|
|
|
IRRI (1996). Standard Evaluation System for Rice. 4th ed., International Network for Genetic Evaluation of Rice, Genetic Resource Center, Los Ba-os. The Philippines.
|
|
|
Jongdee B, Fukai S, Cooper M (2002). Leaf water potential and osmotic adjustment as physiological traits to improve drought tolerance in rice. Field Crops Res. 76:153-163.
Crossref
|
|
|
Kamoshita A, Babu RC, Boopathi NM, Fukai S (2008). Phenotypic and genotypic analysis of drought-resistance traits for development of rice cultivars adapted to rainfed environments. Field Crops Res. 109:1-23.
Crossref
|
|
|
Kato Y, Abe J, Kamoshita A, Yamagishi J (2006). Genotypic variation in root growth angle in rice (Oryza sativa L.) and its association with deep root development in upland fields with different water regimes. Plant Soil 287:117-129.
Crossref
|
|
|
Khush GS (2001). Green revolution: the way forward. Nat. Rev. Genet. 2:815-822.
Crossref
|
|
|
Khush GS (2005). What it will take to feed 5.0 billion rice consumers in 2030. Plant Mol. Biol. 59:1-6.
Crossref
|
|
|
Korzun V, Malyshev S, Voylokov AV, Borner A (2001). A genetic map of rye (Scale cereale L.) combining RFLP, isozyme, protein, microsatellite and gene loci. Theor. Appl. Genet. 102:709-717.
|
|
|
Kramer PJ (1969). Plant and Soil Water Relationships – A modern synthesis. McGraw-Hill Publication Company Ltd.
|
|
|
Li ZK, Xu JL (2007). Breeding for drought and salt tolerant rice (Oryza sativa L.): progress and perspectives. In: Jenks et al. (eds.). Advances in Molecular Breeding toward Drought and Salt Tolerant Crops, Springer pp. 531-564.
Crossref
|
|
|
McCouch SR, Teytelman L, Xu Y, Lobos KB, Clare K, Walton M, Fu B, Maghirang R, Li Z, Xing Y, Zhang Q, Kono I, Yano M, Fjellstrom R, DeClerck G, Schneider D, Cartinhour S, Ware D, Stein L (2002). Development and mapping of 2240 new SSR markers for rice (Oryza sativa L.). DNA Res. 9:199-207.
Crossref
|
|
|
Mitra J (2001). Genetics and genetic improvement of drought resistance in crop plants. Curr. Sci. Bangalore 80:758-763.
|
|
|
Ndjiondjop M, Cisse F, Futakuchi K, Lorieux M, Manneh B, Bocco R, Fatondji B (2010). Effect of drought on rice (Oryza spp.) genotypes according to their drought tolerance level. Second Africa Rice Congress, Bamako, Mali, 22–26 March 2010: Innovation and Partnerships to Realize Africa's Rice Potential, pp. 151-158.
|
|
|
Nguyen HT, Babu RC, Blum A (1997). Breeding for drought tolerance in rice: physiology and molecular genetics considerations. Crop Sci. 37:1426-1434.
Crossref
|
|
|
O'Toole JC (1982). Adaptation of rice to drought-prone environments. In: Drought Resistance in Crops with Emphasis on Rice. Int. Rice Res. Institute, Manila, Philippines, pp. 195-213.
|
|
|
Pandey S, Bhandari H (2009). Drought, Coping Mechanism and Poverty: Insights from rainfed rice farming in Asia. Occasional Paper, 7. Int. Fund Agric. Dev. (IFAD).
|
|
|
Parent B, Suard B, Serraj R, Tardieu F (2010). Rice leaf growth and water potential are resilient to evaporative demand and soil water deficit once the effects of root system are neutralized. Plant Cell Environ. 33:1256-1267.
Crossref
|
|
|
Powell W, Machray GC, Provan J (1996). Polymorphism revealed by simple sequence repeats. Trends Plant Sci. 1:215-222.
Crossref
|
|
|
Price AH, Cairns JE, Horton P, Jones HG, Griffiths H (2002). Linking drought-resistance mechanisms to drought avoidance in upland rice using a QTL approach: progress and new opportunities to integrate stomatal and mesophyll responses. J. Exp. Bot. 53:989-1004.
Crossref
|
|
|
Price AH, Steele KA, Moore BJ, Barraclough PB, Clark LJ (2000). A combined RFLP and AFLP map of upland rice (Oryza sativa L.) used to identify QTL for root-penetration ability. Theor. Appl. Genet. 100:49-56.
Crossref
|
|
|
Price AH, Tomos AD (1997). Genetic dissection of root growth in rice (Oryza sativa L.) II: mapping quantitative trait loci using molecular markers. Theor. Appl. Genet. 95:143-152.
Crossref
|
|
|
Reyniers FN, Truong B, Jacquinot L, Nicou R (1982). Breeding for drought resistance in dryland rice, In: Drought Resistance in Crops with Emphasis on Rice. IRRI, Los Ba-os, Philippines pp. 273-292.
|
|
|
Rosenzweig C, Iglesias A, Yang XB, Epstein PR, Chivian E (2000). Climate Change and U.S. Agriculture: The Impacts of Warming and Extreme Weather Events on Productivity, Plant Diseases, and Pests. Center for Health and The Global Environment Harvard Medical School.
|
|
|
Salunkhe AS, Poornima R, Prince KS, Kanagaraj P, Sheeba JA, Amudha K, Suji KK, Senthil A, Babu RC (2011). Fine mapping QTL for drought resistance traits in rice (Oryza sativa L.) using bulk segregant analysis. Mol. Biotechnol. 49:90-95.
Crossref
|
|
|
Samson B, Hasan M, Wade L (2002). Penetration of hardpans by rice lines in the rainfed lowlands. Field Crops Res. 76:175-188.
Crossref
|
|
Samuel O, Silva J, Oard JH (2010). Association mapping of grain quality and flowering time in elite japonica rice germplasm. J. Cereal Sci. 51:337-343.
Crossref
|
|
Sanchez PL, Wing RA, Brar DS (2014). The Wild Relative of Rice: Genomes and Genomics. In: Zhang Q., Wing A (eds.). Genetics and Genomics of Rice, Plant Genetics and Genomics. Springer New York, pp. 9-25.
|
|
|
Sellamuthu R, Liu GF, Ranganathan CB, Serraj R (2011). Genetic analysis and validation of quantitative trait loci associated with reproductive-growth traits and grain yield under drought stress in a doubled haploid line population of rice (Oryza sativa L.). Field Crops Res. 124:46-58.
Crossref
|
|
|
Senior ML, Murphy JP, Goodman MM, Stuber CW (1998). Utility of SSRs for determining genetic similarities and relationships in maize using an agarose gel system. Crop Sci. 38:1088-1098.
Crossref
|
|
|
Serraj R, Sinclair TR (2002). Osmolyte accumulation: can it really help increase crop yields under drought conditions? Plant Cell Environ. 25:333-341.
Crossref
|
|
|
Sibounheuang V, Basnayake J, Fukai S (2006). Genotypic consistency in the expression of leaf water potential in rice (Oryza sativa L.). Field Crops Res. 97:142-154.
Crossref
|
|
|
Singh N, Dang TT, Vergara GV, Pandey DM, Sanchez D, Neeraja CN, Septiningsih EM, Mendioro M, Tecson-Mendoza EM, Ismail AM, Mackill DJ, Heuer S (2010). Molecular marker survey and expression analyses of the rice submergence-tolerance gene SUB1A. Theor. Appl. Genet. 121:1441-1453.
Crossref
|
|
|
Singh S, Pradhan S, Singh A, Singh O (2012). Marker validation in recombinant inbred lines and random varieties of rice for drought tolerance. Aust. J. Crop Sci. 6:606-612.
|
|
|
Srividhya A, Vemireddy LR, Sridhar S, Jayaprada M, Ramanarao PV, Hariprasad AS, Reddy HK, Anuradha G, Siddiq E (2011). Molecular mapping of QTLs for yield and its components under two water supply conditions in rice (Oryza sativa L.). J. Crop Sci. Biotechnol. 14:45-56.
Crossref
|
|
|
Suji KK, Biji KR, Poornima R, Prince KS, Amudha K, Kavitha S, Mankar S, Babu RC (2011). Mapping QTLs for plant phenology and production traits using indica rice (Oryza sativa L.) lines adapted to rainfed environment. Mol. Biotechnol. 52:151-160.
Crossref
|
|
|
Swamy BP, Vikram P, Dixit S, Ahmed HU, Kumar A (2011). Meta-analysis of grain yield QTL identified during agricultural drought in grasses showed consensus. BMC Genom. 12:319.
Crossref
|
|
|
Temnykh S, DeClerck G, Lukashova A, Lipovich L, Cartinhour S, McCouch S (2001). Computational and experimental analysis of microsatellites in rice (Oryza sativa L.): frequency, length variation, transposon associations, and genetic marker potential. Genome Res. 11:1441-1152.
Crossref
|
|
|
Turner NC (1979). Drought resistance and adaptation to water deficits in crop plants. In: Mussell H, Staples RC (eds.). Stress physiology in crop plants. John Wiley and Sons, New York, pp. 343-372.
|
|
|
Ubi BE, Efisue AA, Oselebe OH (2011). Diversity of drought stress tolerance response in rice cultivars and breeding lines at the vegetative stage. J. Agric. Biotechnol. Ecol. 4:70-89.
|
|
|
Uphoff N, Fasoula F, Iswandi A, Kassam A, Thakur AK (2015). Improving the phenotypic expression of rice genotypes: Rethinking "intensification for production systems and selection practices for rice breeding. Crop J. 3:174-189.
Crossref
|
|
|
Vasant DV (2012). Genome wide association mapping of drought resistance traits in rice (Oryza sativa L.). M Sc. Thesis, Department of Plant Biotechnology, Centre for Plant Molecular Biology and Biotechnology, Tamil Nadu Agricultural University Coimbatore, India.
|
|
|
Venuprasad R, Cruz MT, Amante M, Magbanua R, Kumar A, Atlin GN (2008). Response to two cycles of divergent selection for grain yield under drought stress in four rice breeding populations. Field Crops Res. 107:232-244.
Crossref
|
|
|
Vikram P, Swamy BM, Dixit S, Ahmed HU, Cruz MTS, Singh AK, Kumar A (2011). qDTY 1.1, a major QTL for rice grain yield under reproductive-stage drought stress with a consistent effect in multiple elite genetic backgrounds. BMC genet. 12(1)1.
Crossref
|
|
|
Villa TC, Maxted N, Scholten M, Ford-Lloyd B (2005). Defining and identifying crop landraces. Plant Genet. Resour. Characterization Utilization 3:373-384.
Crossref
|
|
|
Wang H, Inukai Y, Yamauchi A (2006). Root development and nutrient uptake. Crit. Rev. Plant Sci. 25:279-301.
Crossref
|
|
|
Xiong D, Yu T, Zhang T, Li Y, Peng S, Huang J (2015). Leaf hydraulic conductance is coordinated with leaf morpho-anatomical traits and nitrogen status in the genus Oryza. J. Exp. Bot. 66:741-748.
Crossref
|
|
|
Yang JC, Liu K, Zhang SF, Wang XM, Zh Q, Wang XM, Liu LJ (2008). Hormones in rice spikelets in responses to water stress during meiosis. Acta Agronomica Sinica 34:111-118.
Crossref
|
|
|
Yue B, Xiong L, Xue W, Xing Y, Luo L, Xu C (2005). Genetic analysis for drought resistance of rice at reproductive stage in field with different types of soil. Theor. Appl. Genet. 111(6)1127-1136.
Crossref
|
|
|
Zheng H, Babu RC, Pathan MS, Ali L, Huang N, Courtois B, Nguyen HT (2000). Quantitative trait loci for root-penetration ability and root thickness in rice: comparison of genetic backgrounds. Genome 43:53-61.
Crossref
|
|