ABSTRACT
Climate change has an impact on the common bean productivity and its negative effect has to be mitigated using alternative resilience strategies. Field experiments were conducted in 2012 and 2013 cropping seasons at Babile and Haramaya research stations, eastern Ethiopia, to assess the effects of climate change resilience strategies on yield and yield components of common bean. The results of the study revealed that row intercropping + compost application + furrow planting and row intercropping + compost application have increased yield and yield components of common bean and reduced climate change variables. There was a decreasing trend in yield and yield components from the most integrated climate change resilience strategies to the less integrated and sole planting of common bean. Row intercropping + compost application increased the mean number of pods per plant significantly by 40% during 2012 and by 25.9% during 2013 cropping season compared to sole planting at Babile. Compost application + furrow planting increased the average yield of common bean by 20% during 2012 compared to sole planting at Babile. The results obtained from this study indicated the climate change resilience strategies were responsible for increment of yield and yield components of common bean in eastern Ethiopia and in areas with similar agro-ecological conditions.
Key words: Compost application, furrow planting, row intercropping, soil temperature.
Common bean (Phaseolus vulgaris L.) is the most widely produced and consumed legume worldwide and occupies an important place in human nutrition in the east and Great Lakes Regions of Africa for improving the nutritional status of many subsistent farmers (Fininsa and Yuen, 2001; Fininsa, 2003; Shimelis et al., 2006; Lemessa et al., 2011). It is an important source of income nutrition. It provides a rich combination of carbohydrates (60 to 65%), proteins (21 to 25%) and fat (less than 2%), vitamins (Ensminger et al., 1994), good source of iron and zinc (Buruchara et al., 2011), have a low glycemic index and high fibers, contributing to the health conditions of human beings (Martin-Cabrejas, 1997; Bindera, 2009) and supplemental animal feed. It also used in intensifying crop production in space and species mixture (intercropping) and soil fertility management. This ecologically and economically important legume is extensively cultivated in low and mid altitude areas (1200 to 2000 masl) of eastern Ethiopia (Fininsa and Yuen, 2001; Tana et al., 2007; Katungi et al., 2009). Optimum temperature for common bean production is about 24°C. Common bean is grown on about 366,876.94 ha in Ethiopia from which about 463,008.5 tons are produced in the year 2012/2013, with an average national yield of 1.26 tons per hectare (CSA, 2013). Based on area and legume production, this crop ranked second next to faba bean at national level.
Adverse climatic effects can influence farming output at any stage of development from cultivation to harvest. International Panel for Climate Change (IPCC, 2007) predicted that the average global surface temperature would increase by 2.8°C ranging from 1.8 to 4.0°C during 2050, assuming no emission control policies. The agro-climate change includes increased drought affected areas, reduced precipitation at lower altitudes, decreased water availability in mid-latitude and semi-arid low altitudes. Dry spell causes poor seedling emergence, drying of seedlings after germination, stunted growth of crops and poor or no yield (Ghini et al., 2008). Thus, any change in rainfall pattern, soil moisture, soil temperature, soil fertility has direct impact on the productivity and production of crops in general and common bean in particular. In semi-arid agro-ecologies, where food security is a priority concern, climate variability and uncertainty tend to be higher (Garette et al., 2006; IPCC, 2007; Niggli et al., 2009; Valipour, 2014a, b, c.), which will dispose subsistent farmers in the sub-Saharan region to high risks.
The strategies that a particular agro-ecological region will cope with climate variability depend largely on the societies` ability to create and use available knowledge (both indigenous and technology). Enhancing resilience to the effects of climate variability is important, and functional diversity is one of the most effective targets for improved sustainability (Newton et al., 2011). Nowadays, the debate on climate change is not on its occurrence and effects, but rather on how to mitigate the ever-happening effects of climate change to ensure food security of the ever-increasing human population and the proper functioning of the natural ecosystem. This needs to set alternative resilience strategies that mitigate the existing and ever happening impacts of climate change. Climatic condition contains a wide range of weather parameters such as temperature, relative humidity, wind speed, solar radiation and the like (Valipour, 2014a), which could be similar or different in different agroecological zones.
The most important and feasible climate change adaptation strategies for crop production and productivity are increased diversification with the use of species and cultivar mixtures through intercropping (Fininsa, 2003; Tana et al., 2007). Soil water conservation with furrow planting (Wang et al., 2008; Zhao et al., 2012), increasing nutrient use efficiency, improving water-holding capacity of a soil, crop rotation and use of irrigation (Katungi et al., 2009; Niggli et al., 2009: Valipour, 2014c). Intercropping refers to the spatial and temporal arrangement of different crops to exploit natural resources efficiently and enhance productivity per unit area and time by increasing the effective utilization of land, soil moisture, nutrients and solar radiation (Rahimi et al., 2014:Valipour, 2015). It is known to make a more efficient use of growth factors as it captures and makes a better use of radiant energy (Matusso et al., 2012: Valipour, 2015), available water and nutrients (Sullivan, 2003), maintains and improves soil fertility (Sanginga and Woomer, 2009; Seran and Brintha, 2010).
Furrow planting (FP) collects rainwater from ridges and it is especially useful in arid and semi arid regions, where irrigation water is not available or is costly (Wang et al., 2008; Valipour, 2015). Furrow planting system can improve soil moisture storage, prolong the period of crop water availability, and enhance crop growth (Qin et al., 2014). Furrowing has been played vital role in the improvement of grain yield and water use efficiency per unit area of crops (Zhao et al., 2012: Rahimi et al., 2014; Valipour, 2014a,b). Furrowing has been shown to reduce topsoil temperature, especially at the seedling stage, decrease soil evaporation, increase topsoil moisture (Zhou et al., 2012), and enhance plant maturity and grain yield (Li et al., 2012; Zhou et al., 2012: Valipour, 2014b). Furrowing controls water evaporation from the surface soil and improves soil water retention (Grose, 2012; Rahimi et al., 2014; Valipour, 2015).
Composting is one of the few green house gases emission reduction methodologies related to agriculture. Compost is a valuable resource offering significant agricultural and environmental benefits to farmers and the community because of its unique characteristics, which can offer significant returns in the form of increased crop yields and improved quality of produce. Compost contains macro and trace elements essential for healthy plant growth (Stutz et al., 2003; Shehata et al., 2011), which reduces the need to use synthetic fertilizers by returning valuable nutrients to the soil. Compost improves soil structure resulting in increased water holding capacity and nutrient retention of the soil. It also reduces the potential ground water contamination from synthetic fertilizers that are toxic to plants and beneficial organisms. Therefore, it is important to apply nitrogen via composts at environmentally and agronomically responsible levels, which requires understanding of soil factors regulating the release of nitrogen compounds in soil systems, such as moisture content, pH, soil organic matter content and quality, soil texture, buffering capacity and nitrification rate (Sullivan, 2003; Bryan et al., 2009).
Integrating field based climate change resilience strategies for understanding the effects of climate change on crop production that leads to appropriate adaptation measures in action has to be investigated. Research in climate change related issues could result in improved understanding and management of crop production in the face of current and future climate extremes (Garette et al., 2006; Niggli et al., 2009). However, little is known about the effect of different climate change resilience strategies on productivity of crops especially common bean. Therefore, the influence of integrated field based climate change resilience strategies on crop productivity need to be assessed since such data of crop production under climate change resilience strategies would be useful in climate prediction and modelling (Valipour, 2004a). The objective of this study, therefore, was to assess the effects of integrated climate change resilience strategies on the productivity of common bean.
Experimental sites
Field experiments were conducted at Haramaya University experimental field stations at Babile in 2012 and 2013 and Haramaya in 2012, eastern Ethiopia, during main cropping seasons (June to November). Haramaya is located at 09° 26´ N and 42° 3´ E. The altitude of the area is 1980 m above sea level with average annual rainfall of 786.8 mm, with mean minimum temperature of 10.4°C and means maximum temperature of 23.4°C. The location has varied soil types (from luvisol to vertisol) with pH range of 5.0 to 8.0. Babile is situated at 09?13´ N and 42?19´ E, with altitude of 1655 m above sea level with annual total rainfall of 719.2 mm. The mean minimum and maximum temperatures are 15.4 and 28.3°C, respectively. The soil of Babile has sandy loam texture with pH of 7.6.
Both locations represent important common bean and sorghum growing areas in eastern Ethiopia. Babile is a representative of semi-arid agro ecology whereas Haramaya is a representative of midland agro ecology, and both locations are highly affected by climate change events such as increased temperature and variable rainfall pattern. The weather variables (mean maximum and minimum monthly temperature, mean monthly total rainfall) for 2012 and 2013 of both locations during growing seasons are presented in Figure 1.
Treatments and experimental design
Three field based climate change resilience strategies and a control were used as treatments. The strategies consisted of crop diversification (row intercropping), moisture conservation (furrow planting) and soil nutrient management (composting). The treatments were common bean-sorghum row intercropping, furrow planting, compost application, and sole planting. The treatments were applied separately and in integration (minimum of two) using two common bean varieties Gofta (G-2816) and Mexican 142 (G-11239). The varieties were obtained from Melkasa Agricultural Research Center, Ethiopia. Sorghum variety, Teshale (3442-2 OP) developed for the semi-arid environment as moisture stress and striga weed tolerant was used (Table 1). A sole planted sorghum was also included as control. Seventeen treatment combinations were arranged in randomized complete block design in a factorial arrangement and replicated three times per treatment.
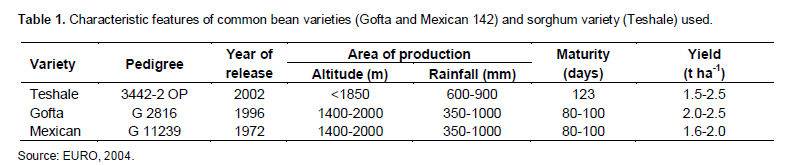
Sorghum was planted in 80 cm inter-row and 25 cm intra-row spacing. In row intercropping, a row of common bean was planted in the center of sorghum rows at 10 cm intra-row and 40 cm inter-row spacing. In the row intercropping, simultaneous planting was used. Similarly, in sole planting of common bean 40 cm inter-row and 10 cm inter-plant spacing with 9 rows per plot were used. Spacing between blocks was 1.2 m and between plots was 1 m. (on a plot size of 3 m x 4 m (12 m2) with the net harvested plot size of 9.6 m2 for intercropping and 8.4 m2 for sole common bean.
Experimental procedures
Compost was applied two weeks before sowing at a rate of 8 tons per hectare, about half the rate recommended for cereals (Stutz et al., 2003) for both plants. Close-ended furrows were prepared by digging 20 centimeters deep rows two weeks before sowing in
order to harvest the rainwater. Sorghum seeds were sown on 21 June 2012 and 28 June 2013 at Babile, 20 June 2012 at Haramaya by hand drilling seeds at the rate of 5 kg ha-1 and after emergence, the plants were thinned to one plant per hill of 25 cm intra-row spacing. Common bean seeds were sown on 11 July 2012 and 05 July 2013 at Babile, 07 July 2012 at Haramaya manually by planting two seeds per hill. After emergence and establishment of seedlings, the rows were thinned to one plant per hill. The plots were hand weeded three times at Haramaya and two times at Babile and cultivated once during the growth periods at both locations.
Data collection
Crop data
The plant heights (cm), number of pods per plant, number of seeds per pod were recorded from 10 randomly taken plants and the averages were calculated. Hundred seed weight (g) of common bean, 1000-kernel weight of sorghum and yield of both crops (kg) were recorded at 12.5% seed moisture content for sorghum and 10% for common bean. The grain yields (kg) of both crops were converted to ton per hectare (t ha-1). Yield data of common bean was recorded for each plot from the middle seven rows of sole and four rows of intercropped plots excluding two border rows on both sides to avoid border effects. In addition, yield of both crops from sole and from the intercropped plots were evaluated by using land equivalent ratio (LER) as described by Mead and Wiley (1980):
Where Yab is seed yield of common bean in intercrop with sorghum; Yaa seed yield/ha of sole common bean; Yba is grain yield/ha of sorghum in intercrop with common bean Ybb is grain yield/ha of sole sorghum.
Meteorological data
Weather data of each location were collected from the nearby meteorological station (as means of five years) for both experimental stations. Weather variables of minimum temperature at 09:00 h and maximum daily air temperature at 18:00 h (°C), relative humidity (%) at 06:00 h, 09:00 h, 12:00 h, 15:00 h and 18:00 h, and daily rainfall (mm) were collected and the averages were calculated. In addition, the data of soil moisture (%) and soil temperature (°C), minimum temperature at 09:00 h and maximum temperature at 15:00 h from sole planting or control and most integrated (row intercropping + compost application + furrow planting) plots of both experimental sites were monitored and recorded with soil thermometer during 2013 cropping season every week from August to October. The data of soil moisture was also recorded using soil sample collector (Auger) from the depth of 20 to 40 cm at 09:00 h from the most integrated and sole planted plots of both sites during of 2013 cropping season every week. The monthly averages for each variable were calculated. The water content of soil was calculated based on wet weight of soil sample obtained immediately after sample collection and dry sample recorded after drying the soil in the oven at 105°C for 24 h. The percentage of water content was calculated based on gravimetric method.
Percentage moisture= (Wet wt-dry wt.)/dry wt. x100.
Data analysis
Data of plant height, number of pods per plant, seeds per pod, 100-seed weight and yield were subjected to analysis of variance using PROC GLM procedure of SAS version 9.1, (SAS Institute. 2003) to determine treatment effects. Homogeneity of variances was tested using the procedure described by Gomez and Gomez (1984) and as the test showed heterogeneity of variances, separate analysis of the two-year and two location data was performed. Differences among treatment means were compared using the Fisher's least significant difference test at 5% level of significance.
Common bean parameters
Plant height
Plant height of common bean was significantly (P<0.05) affected by climate change resilience strategies compared to sole planting (SP) in 2012 cropping season at Babile and Haramaya. Plant height was also significantly (P<0.01) affected by varieties at Haramaya during 2012 (Table 3). The plant height of variety Gofta at Haramaya was higher by 54% than the plant height of Gofta at Babile whereas variety Mexican 142 at Haramaya had 57% higher plant height compared to the plant height of Mexican 142 at Babile during 2012.
Plant height was highest (47 cm) in the row intercropping + compost application + furrow planting (RI + CA + FP) at Haramaya in 2012 cropping season and was significantly lowest in row intercropping + furrow planting (RI + FP) (18 cm) at Babile in 2012 season (Table 2). With regard to the climate change resilience strategies, plant height was highest in the row intercropping + compost application + furrow planting (RI + CA + FP) at Haramaya in 2012 and significantly lowest in the row intercropping + furrow planting (RI + FP) (18 cm) at Babile in 2012 season. The mean plant height of both varieties was higher at Haramaya than Babile in 2012 cropping season. Relatively higher mean plant height of both varieties was obtained during 2012 at Haramaya and during 2013 at Babile. At Babile, in 2013 the plant height of Gofta exceeded by 33% of 2012 and the height of Mexican 142 was more by 39% than that of 2012.
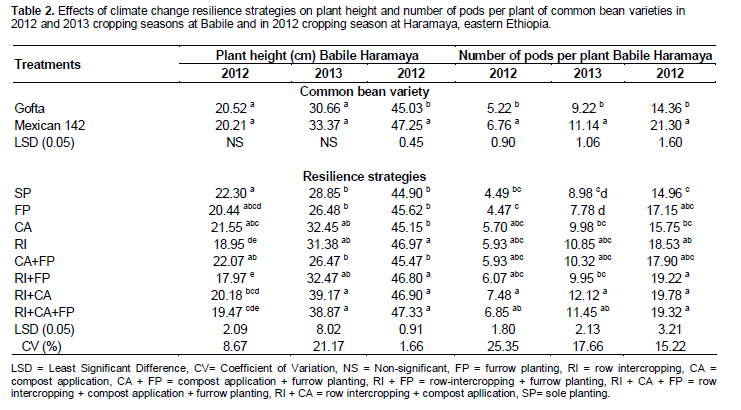
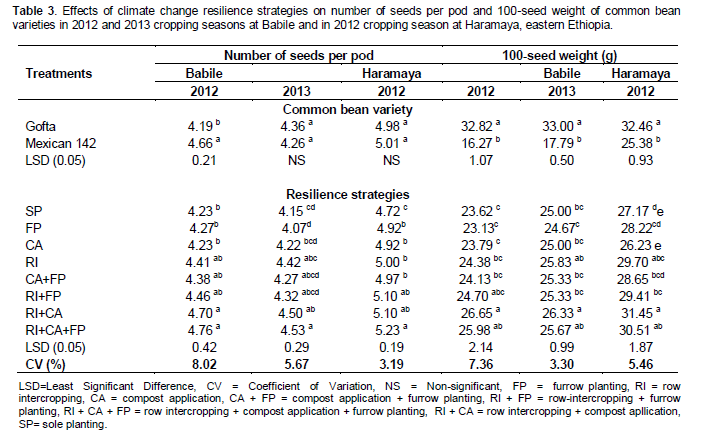
There was a decreasing trend in mean plant height from the most integrated climate change resilience strategies to the less integrated and sole planting (Table 2) except at Babile in 2012 cropping season on both varieties. Relatively higher mean plant height of both varieties was recorded during 2013 cropping season than 2012 at Babile in all treatments while more or less similar mean plant height was recorded from all treatments in 2012 cropping season at Haramaya (Table 2). Furrow planting and compost application + furrow planting increased the mean plant height by 8 and 4.9%, respectively, compared to sole planting at Babile in 2012. Row intercropping + compost application and row intercropping + compost application + furrow planting significantly increased the mean plant height by 26.3 and 25.8%, respectively, compared to sole planting at Babile in 2013 cropping season (Table 2).
Number of pods per plant
Number of pods per plant was significantly (P<0.05) affected by integrated climate change resilience strategies in 2012 at Haramaya and in 2013 at Babile. Likewise, the number of pods per plant was also significantly (P<0.05) affected by variety in both cropping seasons and locations. Significantly, higher number of pods per plant was obtained from Mexican 142 than Gofta at Babile in both cropping seasons and at Haramaya in 2012. At Babile, the number of pods per plant in Mexican 142 was higher by 22.8 and 17.2% than Gofta in 2012 and 2013 cropping seasons, respectively. Higher number of pods per plant was recorded in 2013 than 2012 cropping season at Babile. Varieties Gofta and Mexican 142 had 43 and 39% higher number of pods per plant, respectively, in 2013 compared to 2012 cropping season at Babile. The number of pods per plant of variety Gofta at Haramaya was higher by 63.6% compared to that of Babile in 2012 (Table 2).
With respect to climate change resilience strategies, higher number of pods per plant (7.48) was found in row intercropping + compost application and lower (4.47) was obtained from furrow planting followed by sole planting (4.49) at Babile in 2012. In 2013, higher number of pods per plant (12.1) was recorded from row intercropping + compost application and lower (7.8) from furrow planting at Babile. In 2012 at Haramaya, the mean number of pods per plant was highest in the row intercropping + compost application (19.78) and was significantly lowest in the sole planting (14.96). Row intercropping + compost application and row intercropping + compost application + furrow planting increased the mean number of pods per plant significantly by 40 and 34%, respectively, compared to sole planting in 2012 cropping season and by 25.9 and 21.6%, respectively, compared to sole planting in 2013 at Babile. Row intercropping + compost application and row intercropping + compost application + furrow planting increased the mean number of pods per plant by 24.4 and 22.6%, respectively, compared to sole planting at Haramaya in 2012 cropping season.
Number of seeds per pod
Number of seeds per pod was significantly (P<0.05) affected by integrated climate change resilience strategies in 2012 at Haramaya and in 2013 at Babile. On the other hand, the number of seeds per pod was not significantly affected by variety in both cropping seasons and locations. Significantly, higher number of seeds per pod was obtained from Mexican 142 than Gofta at both locations in 2012 and lower number of seeds per pod from Mexican 142 in 2013 at Babile. At Babile, the number of seeds per pod in Mexican 142 variety was higher by 10% than Gofta variety during 2012. Higher number of seeds per pod of Gofta was recorded in 2013 than 2012 cropping season at Babile (Table 3). In 2012 at Haramaya, the mean number of seeds per pod was higher in the row intercropping + compost application + furrow planting and was significantly lower in the furrow planting, sole planting and compost application. At Babile, the mean number of seeds per pod was significantly higher in the row intercropping + compost application + furrow planting and row intercropping + compost application and was significantly lower in the sole planting and compost application in 2012 cropping season. At Babile, the mean number of seeds per pod was higher in the row intercropping + compost application + furrow planting and row intercropping + compost application, respectively, and was significantly lower in the furrow planting and sole planting, respectively,in 2013 cropping season (Table 3).
Hundred seed weight
Hundred seed weight was significantly (P<0.05) affected by integrated climate change resilience strategies during both cropping seasons and locations. It was also significantly (P<0.0001) affected by variety in both cropping seasons and locations. Hundred seed weight was highest (31.45 g) in the row intercropping + compost application at Haramaya in 2012 and was significantly lowest (23.1 g) in the furrow planting at Babile during 2012. At Babile, the mean 100-seed weight was highest in the row intercropping + compost application and was significantly lowest in the furrow planting in 2013 cropping season.
Hundred seed weight was significantly higher for variety Gofta than Mexican 142 in both cropping seasons and locations (Table 3). The mean 100-seed weight of variety Gofta was higher by 50.4 and 46% in 2012 and 2013 cropping season, respectively, at Babile and by 21.8% in 2012 at Haramaya. Row intercropping + compost application increased the mean 100-seed weight significantly by 11.4 and 5% at Babile in 2012 and 2013 cropping season, respectively, and by 13.6% at Haramaya in 2012. Furrow planting at Babile during both cropping seasons and compost application at Haramaya in 2012 reduced the mean 100-seed weight as compared to sole planting (Table 3).
Grain yield
Grain yield weight was significantly (P<0.0001) affected by climate change resilience strategies in the 2012 and 2013 cropping seasons at both locations (Table 4). Likewise, grain yield was significantly (P<0.0001) affected by the common bean varieties where variety Gofta had significantly higher average grain
yield (t ha-1) than variety Mexican 142 at both locations and in both cropping seasons at Babile. At Babile, in 2013, the average yield of variety Gofta was higher by 60% compared to that of 2012 and the average yield of variety Mexican 142 was higher by 63.2% than that of 2012. Likewise, at Haramaya the average yield of variety Gofta was higher by 25% compare to the average yield of Mexican 142 in 2012. The average yield of both varieties was significantly higher at Haramaya compared to Babile (Table 4).
In 2012 at Haramaya, the mean grain yield (t ha-1) was highest (3.33) in the furrow planting and was significantly lowest (1.56) in the row intercropping + furrow planting. At Babile, the mean grain yield was significantly highest (0.65) in the compost application + furrow planting and was lowest (0.33) in the row intercropping + furrow planting in 2012 cropping season. At Babile, the mean grain yield (t ha-1) was highest (1.46) in the compost application and was significantly lowest (1.05) in the row intercropping + furrow planting in 2013 cropping season (Table 4). Compost application + furrow planting increased the average yield of common bean by 20 and 8.7%, respectively, in 2012 and 2013 compared to sole planting at Babile. Compost application also increased the average yield by 27.9% in 2012 at Haramaya compared to sole planting. Relatively lower yield was obtained from intercropping plots than sole plots at both locations and in both seasons.
Land equivalent ratio
The four intercropping climate change resilience strategies provided yield advantages over the sole cropping climate change resilience strategies such as compost application, furrow planting, compost application + furrow planting and sole planting treatments. Relatively higher land equivalent ratio (LER) was obtained from the most integrated (row intercropping + compost application + furrow) and row intercropping + compost application at Babile in both cropping seasons and at Haramaya, in 2012 where the row intercropping + compost application had the highest LER (1.74). On the other hand, the lowest LER (1.50) was found in the row intercropping at Babile in 2012 cropping season and 1.51 at Haramaya in 2012 (Table 5).
Response of soil moisture and soil temperature to the resilience strategies
The most integrated climate change resilience strategy significantly reduced the average soil temperature in both varieties of common bean during all the growth period with the exception of month of August at Babile. The soil average temperature was significantly (P<0.01) reduced by the most integrated climate change resilience strategy (row intercropping + compost application + furrow planting) at Babile in 2013 cropping season (Table 6). On the average, 2, 4.84 and 8.24% reduction of average soil temperature was recorded in August, September and October, respectively. The varieties did not show any significant difference in soil temperature. The soil moisture was significantly (P<0.01) affected by the most integrated climate change resilience strategy on both varieties at Babile during 2013 cropping season (Table 6). Soil moisture content was increased by 16.7, 15.4 and 15% during August, September and October, respectively, at Babile. Variety Mexican 142 had significantly higher soil moisture than Gofta in August 2013.
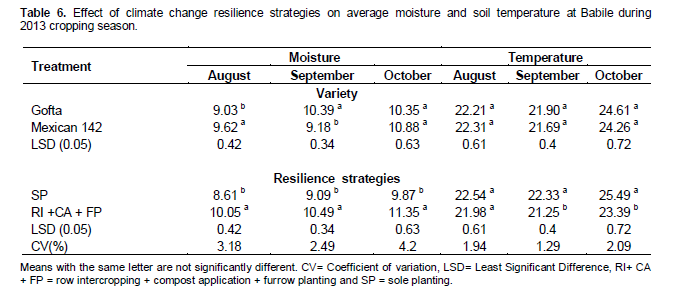
Plant height, number of pods per plant, number of seeds per pod, 100-seed weight and grain yield were significantly affected by climate change resilience strategies. The highest plant height was obtained from the row intercropping + compost application of Mexican 142 variety at Haramaya in 2012 might be due to the indeterminate growth and climbing nature of Mexican 142 to component crop, the sorghum and the availability of nutrients from the compost. There was a decreasing trend in yield and yield components from the most integrated climate change resilience strategies to the less integrated and sole planting.
The result of this study revealed that there is the synergic effect of the climate change resilience strategies, in increasing yield and yield components. It is important to note that the benefits of climate change resilience strategies discussed above are location specific that is why compost application + furrow planting and furrow planting increased plant height by 16 and 14%, respectively, compared to sole planting on variety Gofta at Babile in 2012. Although the water holding capacity of soil at Babile is very low due to its light texture (sandy loam), integration of compost with furrow planting improved the productivity of common bean. In the shortage of rainfall especially in 2012 cropping season, intercropping common bean with sorghum, did not significantly increase yield and yield components of common bean. It might be because of the competition for limited water available between the two crops, such that the canopy of common bean in the intercropping system was very low since sorghum had higher water absorption capacity. In 2013, row intercropping + compost application + furrow planting and row intercropping + compost application, significantly increased yield and yield components at Babile. The intercropped climate change resilience strategies, significantly increased plant height, number of pods per plant, number of seeds per pod and 100-seed weight and grain yield of both crops than sole planting at Babile in 2013, which might be due to relatively higher rainfall and lower mean temperature during 2013 compared to 2012 at Babile.
The climate change resilience strategies were more effective when they were integrated and when the risk factors are minimized. At Haramaya, there was heavy frost on 14 October 2012, when the crop was at physiological maturity, which might be the cause for reduction in yield and yield components, especially 100 seed weight of variety Mexican 142, the late maturing variety. It is highly observed that relatively lower yield obtained from intercropping was compensated by the yield of the component crop (sorghum) when evaluated using land equivalent ratio (LER) and all of the intercropping plots had additional yield advantage over sole crop of common bean.
The present study revealed that the integrated climate change resilience strategies (row intercropping + compost application + furrow planting, row intercropping + compost application) tested at Babile and Haramaya are feasible in conserving soil moisture, increasing productivity of common bean, averting crop failure risks, hence increasing climate change resilience. This result was in agreement with the reports of Niggli et al. (2009) that described sustainable land management practices such as compost application, crop diversification and water harvesting can improve soil carbon sequestration, crop yields and enhances resilience to climate change and increasing crop productivity (Bryan et al., 2011). Compost application had increased yield and yield components of both crops might be due to the availability of major elements of plant nutrients. In addition to its long-term effect of maintaining soil chemical property and physical structure, reduces emissions of greenhouse gases by compensating utilization of inorganic fertilizers harmful to the atmosphere (Niggli et al., 2009). The chemical analysis of compost used in this study had higher organic content; higher nitrogen content and higher available phosphorus, hence might have improved soil fertility, which agreed with the reports of Bryan et al. (2011), where the application of compost to fields provides benefits in terms of crop yields, increases climate change resilience. Furrowing could be one of the factors that increased yield and yield components, reduced soil temperature and increased soil moisture when integrated with intercropping and compost application based on the soil type and rainfall amount. This result is also agreed to report of Qin et al. (2014) where furrowing increased yield and soil moisture and to the report of Grose et al. (2012) where furrowing can reduce yield variability and heat stress in dry areas.
Overall, the results of present study showed that the most integrated and row intercropping + compost application can be applied to increase the climate change resilience at higher extent, although the optimal integration of strategies depends on a number of factors including crop variety, soil type and agroecological zone. Generally integrating climate change resilience strategies reduced climate variability and significantly increased yield and yield components of common bean: However, the application rate of compost and furrow preparation needs further investigation for common bean production.
The soil moisture and temperature data showed that the most integrated climate change resilience strategy gave promising result in reduction of soil temperature and retention of water in the soil, hence, might be responsible for mitigating climate change. The results obtained from this study suggested the importance of climate change resilience strategies alone and in combination for increasing of yield and yield components of common bean in eastern Ethiopia and in areas with similar agro-ecological conditions. The compost application rate and furrow preparation need to be further investigated for common bean and other crops in the study area. The importance of solar radiation, temperature, relative humidity, and wind speed for calculation of reference evapotranspiration need to be investigated for proper utilization of furrow planting.
The authors have not declared any conflict of interest.
Authors would like to thank Masresha Lebie, Woinishet Feleke, Birhanu Asfaw, Azeb Tegenu and the late Cholamu Faltamo, field assistants in the School of Plant Sciences of Haramaya University, for their assistance in data collection and fieldwork. The project was financed by Haramaya University.
REFERENCES
Bindera J (2009). Technical Report on Analysis of Haricot Bean Production, Supply, Demand and Marketing Issues in Ethiopia. Ethiopia Commodity Exchange Authority, Ethiopia. pp. 10-22. |
|
Bryan E, Ringler C, Okoba B, Koo J, Herrero M, Silvestri S (2013). Can agriculture support climate change adaptation, greenhouse gas mitigation and rural livelihoods? Insights from Kenya. Clima. Chan. 118:151–165.
Crossref |
|
Buruchara R, Chirwa R, Sperling L, Mukankusi C, Rubyogo JC, Muthoni RA, Abang MM (2011). Development and Delivery of Bean Varieties in Africa: The Pan-Africa Bean Research Alliance (PABRA) Model. Afr. Crop Sci. J. 19(4):227-245. |
|
CSA (Central Statistical Agency) (2013). Statistical Bulletin on Area and Production of Crops, (Private Peasant Holding, Meher Season). Central Statistical Agency, Ethiopia. pp. 1-126. |
|
EARO (Ethiopian Agricultural Research Organization) (2004). Directory of Released Varieties and Their Recommended Cultural practices. EARO, Addis Ababa, Ethiopia. pp.1-36. |
|
Ensminger AH, Ensminger ME, Konlande JE, Robson JRK (1994). Food and Nutrition Encyclopedia. 2nd Eds. CRC Press, Florida. |
|
Fininsa C, Yuen J (2001). Association of bean rust and common bacterial blight epidemics with cropping systems in Hararghe Highlands, Eastern Ethiopia. Int. J. Pest Manage. 47(3):211-219.
Crossref |
|
Fininsa C (2003). Relationship between common bacterial blight severity and bean yield loss in pure stand and bean-maize intercropping systems. Int. J. Pest Manage. 49(3):177-185.
Crossref |
|
Garette KA, Dendy SP, Frank EE, Rouse MN, Travers SE (2006). Climate change effects on plant disease: genomes to ecosystems. Ann. Rev. Phytopathol. 44(1):489–509.
Crossref |
|
Ghini R, Hamada E, Bettiol W (2008). Review on climate change and plant diseases. Sci. Agric. J. 65(1):98-107.
Crossref |
|
Gomez KA, Gomez AA (1984). Statistical Procedures for Agricultural Research. 2nd Edition. Willey Interscience Publication, New York. |
|
Grose PJ (2012). Restoring Seasonal Wetland Using Woven Black Plastic Weed Mat to Overcome a Weed Threshold. Ecol. Manage. Rector. 13:191–195.
Crossref |
|
IPCC (Inter-governmental Panel on Climate Change) (2007). Fourth Assessment Report on Climate Change Mitigation. Contribution of Working Group of the Intergovernmental Panel on Climate Change. Cambridge University Press, Cambridge, New York, USA. |
|
Katungi E, Farrow A, Chianu J, Sperling L, Beebe S (2009). Base Line Research Report on Common Bean in Eastern and Southern Africa: A Situation and Outlook Analysis of Targeting Breeding and Delivery Efforts to Improve the Livelihoods of the Poor in Drought Prone Areas. ICRISAT, Uganda. pp. 1-126. |
|
Lemessa F, Sari W, Wakjira M (2011). Association between Angular Leaf Spot (Phaeoisariopsis Griseola (Sacco) Ferraris) and Common Bean (Phaseolus vulgaris L.) Yield Loss At Jimma, Southwestern Ethiopia. Plan. Pathol. J. 110(2):57-65. |
|
Li R, Hou X, Jia Z, Han Q, Yang B (2012). Effects of Rainfall Harvesting and Mulching Technologies on Soil Water, Temperature, and Maize Yield in Loess Plateau Region of China. Soil Res. 50:105–113. |
|
Martin-cabrejas MA, Eseban RM, Perez P, Maina G, Waldron KW (1997). Changes in Physico Chemical Properties of Dry Beans (Phaseolus vulgaris L.) during Long-Term Storage. J. Agric. Food Chem. 45(1):3223-3227.
Crossref |
|
Matusso JMM, Mugwe JN, Mucheru-Muna M (2012). Research Application Summary on Potential Role of Cereal-Legume Intercropping Systems in Integrated Soil Fertility Management in Smallholder Farming Systems of Sub-Saharan Africa. Kenyatta University, Kenya. |
|
Mead R, Wiley RW (1980). The concept of a 'Land Equivalent Ratio' and Advantages in Yield from Intercropping. J. Exp. Agric. 16(1):217-228.
Crossref |
|
Newton AC, Johnson SN, Gregory P (2011). Implications of Climate Change for Diseases, Crop Yields and Food Security. Euph. 179(1):3–18.
Crossref |
|
Niggli U, Fliebbach A, Hepperly P, Scialabba N (2009). Low Greenhouse Gas Agriculture: Mitigation and Adaptation Potential of Sustainable Farming Systems. FAO, Italy. |
|
Qin S, Zhang J, Dai H, Wang D, Li D (2014). Effect of Ridge Furrow and Plastic Mulching Planting Patterns on Yield Formation and Water Movement of Potato in a Semi-Arid Area. Agric. Water Mange. 131:87-94.
Crossref |
|
Rahimi S, Ali M, Sefidkouhi G, Raeini-Sarjaz M, Valipour M (2014). Estimation of actual evapotranspiration by using MODIS images (a case study: Tajan catchment). Arch. Agric. Soil Sci. 61(5):695-709.
Crossref |
|
SAS (Statistical Analysis System) (2003). SAS/STAT Guide for Personal Computers, version 9.1 Eds. SAS Institute Inc., NC. |
|
Sanginga N, Woomer PL (2009). Integrated soil fertility management in Africa: Principles, Practices and Development Process. Institute of the International Centre for Tropical Agriculture, Kenya. |
|
Seran TH, Brintha I (2010). Review on Maize Based Intercropping. J. Agron. 9(3):135-145.
Crossref |
|
Shehata SA, Ahmed YM, Emad AS, Omaima SD (2011). Influence of Compost Rates and Application Time on Growth, Yield and Chemical Composition of Snap Bean (Phaseolus vulgaris L.). Aust. Bas. Appl. Sci. 5(9):530-536. |
|
Shimelis E, Meaza M, Rakshit S (2006). Physico-Chemical Properties, Pasting Behavior and Functional Characteristics of Flours and Starches from Improved Bean (Phaseolus vulgaris L.) varieties grown in East Africa. Agric. Eng. Int. 8(1):05-15. |
|
Stutz J, Donahue S, Mintzer E, Cotter A (2003). Technical Report on Compost in Landscaping Applications. Tellus Institute, MA. pp. 1-16. |
|
Sullivan P (2003). Agronomy Systems Guide on Intercropping Principles And Production Practices. Appropriate Technology Transfer for Rural Areas (ATTRA) Butte, California. |
|
Tana T, Fininsa C, Worku W (2007). Agronomic Performance and Productivity of Common Bean (Phaseolus Vulgaris L.) Varieties in Double Intercropping with Maize (Zea mays L.) in Eastern Ethiopia. Asia. J. Plant Sci. 6(1):749-756. |
|
Valipour M (2014a). Use of average data of 181 synoptic stations for estimation of reference crop evapotranspiration by temperature-based methods. Water Resour. Mange. 28:4237–4255.
Crossref |
|
Valipour M (2014b). Analysis of potential evapotranspiration using limited weather data. Appl. Water Sci. DOI 10.1007/s13201-014-0234-2.
Crossref |
|
Valipour M (2014c). Assessment of different equations to estimate potential evapotranspiration versus FAO Penman Monteith method. Acta Adv. Agric. Sci. 2(11):14-27. |
|
Valipour M (2015). Importance of solar radiation, temperature, relative humidity, and wind speed for calculation of reference evapotranspiration. Arch. Agro. Soil Sci. 61(2):239–255. |
|
Wang Q, Zhang EH, Li FM, Li FR (2008). Runoff Efficiency and the Technique of Micro Water Harvesting With Ridges and Furrows, For Potato Production in Semi-Arid Areas. Wat. Resour. Mange. 22:1431–1443.
Crossref |
|
Yoseph T, Sorsa Z (2014).Evaluation of sorghum (Sorghum bicolor L.) Moench) varieties, for yield and yield components at Kako, Southern Ethiopia. J. Plan. Sci. 2(4):129-133. doi:10.11648/j.jps.20140204.12 |
|
Zhao H, Xiong YC, Li FM, Wang RY, Qiang SC, Yao TF, Mo F (2012). Plastic Film Mulch for Half Growing-Season Maximized WUE and Yield of Potato via Moisture-Temperature Improvement in A Semi-Arid Agroecosystem. Agric. Water Mange.104:68–78.
Crossref |
|
Zhou LM, Jin SL, Liu CA, Xiong YC, Si JT, Li XG, Gan YT, Li FM (2012). Ridge–Furrow and Plastic-Mulching Tillage Enhances Maize–Soil Interactions: Opportunities and Challenges in a Semiarid Agroecosystem. Fie. Crop. Res. 126:181–188.
Crossref |