This innovative research aimed to study the potential of the use of solid wastes from cassava root processing industry (Manihot esculenta Crantz) (peel, bagasse and the mix peel + bagasse) as natural adsorbents of Cr3+ from waters. In a first step, the biosorbents were characterized chemically, structurally and morphologically. This way were performed infrared spectrum analysis, scanning electron microscopy, point of zero charge and chemical composition for all studied natural adsorbents. After that, studies evolving kinetics, equilibrium, thermodynamics and desorption of Cr3+ were performed. According to the obtained data the cassava adsorbents proved to be efficient in the removal of Cr3+, being found in Langmuir and D-R models the best fitting, indicating monolayer chemisorption, with endothermic character. The cassava materials presented lower rates of desorption also suggesting a chemisorption of Cr3+ molecules. By the obtained results it was concluded that the use of cassava biosorbents are viable for the decontamination of waters containing Cr3+, being the use of cassava wastes for adsorption a complementation of the final steps of cassava productive chain.
In The solid wastes produced by the industrialization of cassava roots, the peel of cassava roots and the fibrous material originated by the starch extraction (bagasse) do not have any other destiny than animal feeding (Menezes et al., 2004).
Only in Brazil, every year are produced approximately 1 million tons of cassava peel. It is estimated that globally this number can reach 11 million tons every year, being all these wastes a cause of major problems by the incorrect disposal frequently in industries and farms. In function of the many problems of chemical pollution which the environment is exposed, among them, the hydric pollution by heavy metals (Li and Bai, 2005), is from major importance the research for new technologies for low cost remediation of toxic metals from hydric bodies, because these metals cause severe risks to humans, animals and environment (Montanher, 2005).
One of the alternatives for natural resources decontamination is the process of adsorption, which regulates the mobility and availability of the pollutants in solution, being the molecules presents in a fluid, liquid or gas, which can spontaneously accumulate above the solid surface (Reddy et al., 2010).
The Cr3+ contamination is normally treated with minor attention when compared to Cr6+, due to its toxicity degree, however some researches relates that concentrations of Cr3+ cause severe perturbations in human erythrocytes (Suwalsky et al., 2008), being in this way the removal of Cr3+ from waters extremely important from the environmental and human point of view. The removal of heavy metals, such as Cr3+, presents in contaminated effluents can be performed using organic and inorganic materials, being this a very attractive option, mainly in function of the higher availability of the materials and for low cost (Demirbas, 2008).
Many authors relate the use of alternative biosorbents for the removal of pollutants from waters, as example of: pine bark (Argun and Dursun, 2008), cocoa husks (Meunier et al., 2003), rice brain (Montanheir et al., 2005); dry mass of Eichhornia crassipes (Gonçalves Jr. et al., 2009), sugar cane bagasse in natura and modified (Dos santos et al., 2011), however there are not any studies relating the use of cassava solid wastes and its use as natural adsorbents.
In this way, the objective of this study was to evaluate these renewable materials (Peel, bagasse and peel + bagasse) as natural adsorbents, studying its adsorption characteristics in function to Cr3+ remediation.
Obtaining of the natural adsorbents
The raw matter (peel, bagasse and peel + bagasse) was obtained in industry located at Toledo – Paraná. The material was dried at 60°C for 48 h, crushed and sieved, maintaining the final granulometry ranging from 14 to 60 mesh. Non treatment that modified chemically the adsorbent materials was performed, being these only crushed and sieved for standardization of particle size. It was also performed the chemical characterization of biosorbents, by nitropercloric digestion of the materials (AOAC, 2005), for determination of the concentrations of potassium (K), calcium (Ca), magnesium (Mg), copper (Cu), iron (Fe), manganese (Mn), zinc (Zn), cadmium (Cd), lead (Pb) and chromium (Cr) by flame atomic absorption spectrometry (FAAS) (Welz and Sperling, 1999). For the evaluation of functional groups responsible for the adsorption with Cr3+ was performed the infrared characterization of adsorbents, using a Shimadzu Infrared Spectrophotometer FTIR- 8300 Fourier Transform, in the region between 400 and 4000 cm-1. The spectrum was obtained using KBr tablets.
The material surface was also evaluated morphologically by scanning electron microscopy (SEM), with a FEI Quanta 200 microscope, operating in a voltage of 30 kV. The samples were placed in a double-sided tape attached to a carbon sample holder and then were metallized with gold to a thickness of about 30 nm by using a sputter coater Baltec Scutter SCD 050. It was also determined the zero point charge (pHPZC) of the adsorbent, which refers to the pH which the resultant of adsorbent superficial charges is null (Mimura et al., 2010).
Preparation of contaminated water
The monoelementar solution fortified with Cr3+ were prepared using chrome nitrate [Cr(NO3)3 9H2O P.A. ≥ 99,0% Sigma-Aldrich]
Adsorption studies in function of pH and adsorbent mass
The removal of chromium by the adsorption process is dependent from many factors, between them it can be highlighted the adsorbent quantity, pH of the contaminated water and contact time between adsorbent/adsorbate. This way, in this preliminary study were maintained constant the system stirring (200 rpm), stirring time (1.5 h) temperature (25°C) and chromium concentration (10 mg L-1), varying only the pH of the solutions and the adsorbent mass.
The Cr3+ solutions were adjusted in three pH conditions (4.0; 5.0 and 6.0). The pH values were adjusted using HCl solutions (Vetec, 37%) or NaOH (Vetec, 99%), both in the concentration of 0.1 mol L-1. The adsorbent masses were 200, 400, 600, 1000 and 1200 mg, being each adsorbent material evaluated separately.
In erlenmeyers of 125 ml, were added the adsorbent material and 50 ml of Cr3+ solution (10 mg L-1), being after 1.5 hours the samples were filtered with qualitative filter (Unifil), being determined the levels of Cr3+ by FAAS. (Welz and Sperling, 1999). By the obtained results for equilibrium concentration was calculated the adsorbed quantity in equilibrium Equation (1).
Qeq = [(C0 - Ceq).m] V (1)
Being: Qeq the quantity of Cr3+ adsorbed by adsorbent unit (mg g-1), m is the adsorbent mass used (g), C0 correspond to the initial concentration of Cr3+ in solution (mg L-1), Ceq is the concentration of Cr3+ in solution in the equilibrium (mg L-1) and V is the volume of solution used (L). The percentage of Cr3+ removal was calculated according Equation (2):
%R= 100 - (Ceq/C0) .100 (2)
Being: %R the percentage of Cr3+ removal by the adsorbent, Ceq the concentration of Cr3+ in equilibrium (mg L-1) and C0 the initial concentration in solution (mg L-1).
Kinetics and equilibrium studies
In order to determine the influence of contact time in the adsorption process, were used the best conditions found in the previous studies of adsorbent mass and pH in different stirring time intervals.
This way, 400 mg was the mass used for the three adsorbents and pH of 5.5 of Cr3+ solution. The stirring time intervals were 5, 10, 20, 40, 60, 80, 100, 120, 140 and 160 min, being after these intervals the samples were filtered and aliquots sampled for FAAS concentration determination (Welz and Sperling, 1999).
For the evaluation of the kinetic mechanism which controls the adsorption process, the following models were used, according to the literature citations and studies: Pseudo first order (Aksu, 2001), pseudo second order (Ho and Mckay, 1999), Elovich (Ho and Mckay,2004) and intraparticle diffusion (Witek-Krowiak et al., 2011). Adsorption isotherms were also built, using initial concentrations (C0) ranging from 5 to 200 mg L-1, these results were linearized by
the models of Langmuir, Freundlich and Dubinin-Radushkevich (D-R) ().
Desorption process
For the desorption process, the adsorbents used for the construction of isotherms were separated by filtration process, washed in ultrapure water and dried at 60 ± 2 °C for 24 h. The adsorbent mass recovered was in contact with 50 mL of HCl 0.1 mol L-1, with stirring at 200 rpm for 60 min at 25°C. The final concentration of the desorbed Cr3+ was determined by FAAS and calculated by Equation (3):
D=[Ceq(des)/Ceq(ads)] . 100 (3)
Being Ceq(des) and Ceq(ads) (mg L-1) refer to the Cr3+ concentration desorbed and quantity adsorbed of Cr3+ in equilibrium by the adsorbents.
Comparative studies with active coal (AC)
With the purpose of comparison the obtained results between the cassava adsorbents and one commercial adsorbent, the active coal P.A. (Synth) powder, which is wildly used for the removal of pollutants from water bodies (Oliveira et al., 2008) was used. This way, the same conditions used for the construction of isotherms and desorption tests were used for the comparison with active coal.
Thermodynamics of adsorption
The influence of the temperature in the adsorption process was evaluated in five conditions: 25, 35, 45, 55 and 65°C. For this purpose 50 mL of Cr3+ solution at 50 mg L-1 concentration. The solution was added in erlenmeyers of 125 ml with 400 mg of adsorbent material. After that period of time was performed the determination of Cr3+ concentration in equilibrium by FAAS (Welz and Sperling, 1999).
By the obtained data the thermodynamics parameters were evaluated being also investigated the nature of the adsorption process. For that purpose the Gibbs free energy (ΔG), enthalpy (ΔH) and entropy (ΔS) were calculated (Sari et al., 2007; Gonçalves et al., 2008). All adsorption and desorption processes described in the items above were performed in triplicate.
Biosorbents characterization
Concentrations of lead were found in the adsorbent materials (Table 1), which is an indicative of the presence of this heavy metal in soil where the plants were cultivated. The lead concentration found in the biosorbents is possibly originated from cassava plants from soil contaminated with this heavy metal.
According to Gonçalves Jr. et al. (2011) evaluating the fertilization residual effect of wheat crop with contaminated fertilizers, found soil contaminated with Pb and consequently absorption of this metal by the wheat plants.Peels from vegetal origin are basically constituted of cellulose, hemicelulose and lignin (Pehlivan et al., 2009). Figure 1 show the micrographs of the adsorbents in magnifications until 20.000 times.
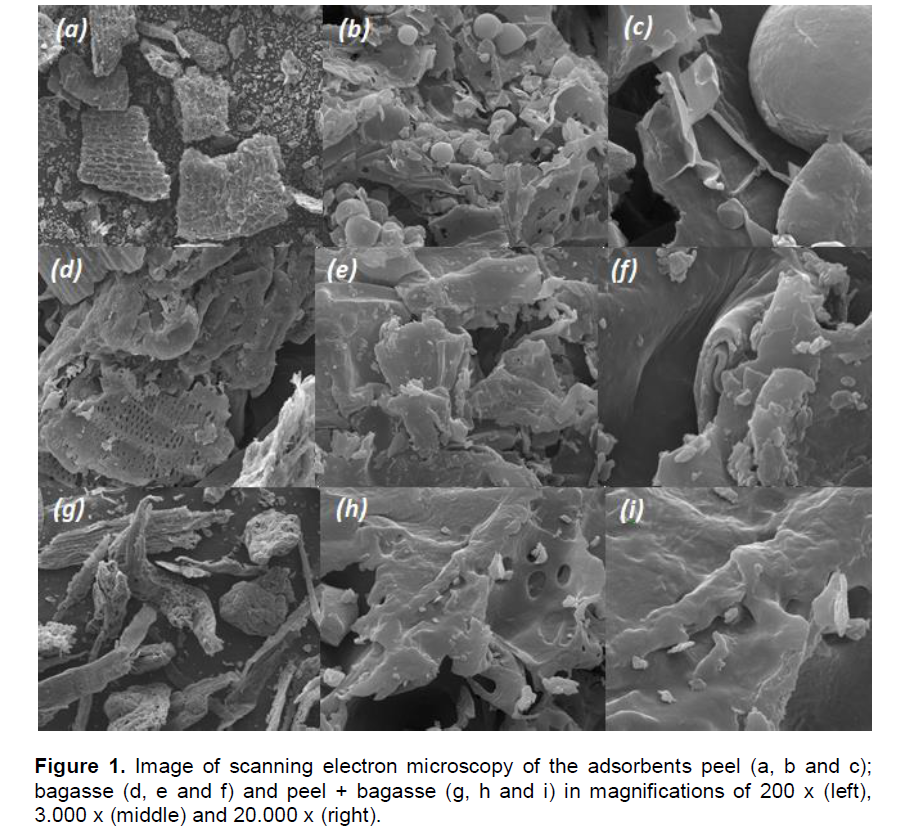
The results (Figure 1) show an adsorbent surface of fibrous and spongy aspect, with irregular and heterogeneous structure. Many of these cavities and cracks can be highlighted, showing a material with high superficial area, with favorable characteristics of adsorption. The pHPZC, according to Mimura et al. (2010), is defined as the pH which the surface of the solid possesses neutral charge. In solutions with pH below pHPZC, in adsorbents surface predominate positive charges and, in solutions with pH above pHPZC, the superficial liquid charge is negative, providing better conditions for cations adsorption.
The obtained results of pHPZC (Figure 2) show that the equivalence point of positive and negative charges for the adsorbents is 6.00 for peel, 6.17 for bagasse and 6.24 for peel + bagasse. This way, the adsorption of cations, in this case Cr3+ is favored by pH values above than the pHPZC (Tagliaferro et al., 2011).
Adsorption process in function of pH solution and adsorbent mass
The pH is one of the parameters with great importance in the adsorption process, because it interferes in the solid-solution interface, causing influence in the active sites charges of biomass and also in the adsorbate behavior (Gao and Wang 2007; Fávere et al., 2010). As observed in Figure 3, adsorbent masses higher than 400 mg were not found higher removal rates of chromium, in this manner, for the posterior tests were used 400 mg of the adsorbent material with solution Cr3+ at pH 5.5.
It is also possible to observe in Figure 3 that for lower concentrations (10 mg L-1) the materials peel, bagasse and peel + bagasse were capable of removing more than 90% of the Cr3+ in solution. It is observed that in the studied pH range, the removal % was superior to 90% in all adsorbent materials, emphasizing the adsorbent peel + bagasse, which removed great quantities of chromium from solution. The value of 8 g of adsorbent per litter of contaminated water is the ideal proportion.
Many materials equally from vegetal origin have the adsorption influenced by pH of contaminant solution, as verified in mesocarp and endocarp macadamia (Vilas Boas et al., 2012), bark of Pinnus elliottii (Gonçalves Jr. et al., 2012), rice rusk (Mimura et al., 2010), rice bran (Montanher et al., 2005), peanut husk (Runping et al., 2008), among others.
Influence of contact time between adsorbent/adsorbate
The influence of contact time between adsorbent/adsorbate for the materials peel, bagasse and peel + bagasse is illustrated at Figure 4. It is shown in Figure 4, the occurrence of an increase of Cr3+ adsorption by the materials in the course of time, being after 60 min of stirring the dynamic equilibrium is reached, not occurring higher adsorption rates for periods of time higher than 60 min, indicating a fast adsorption.
The obtained results, according to the kinetic models of adsorption are shown in Table 2. For the interpretation of the parameters in Table 2, besides the values of the coefficient of determination (R2) present best fit, the values of Qeq obtained by the model must be very close from the obtained experimentally (Qeq exp.) (Febrianto et al., 2009). Comparing the values obtained in Table 2, the model of pseudo first order, which suggests physisorption predominance, does not prove convincingly the observed adsorption rates, with unsatisfactory R2 values.
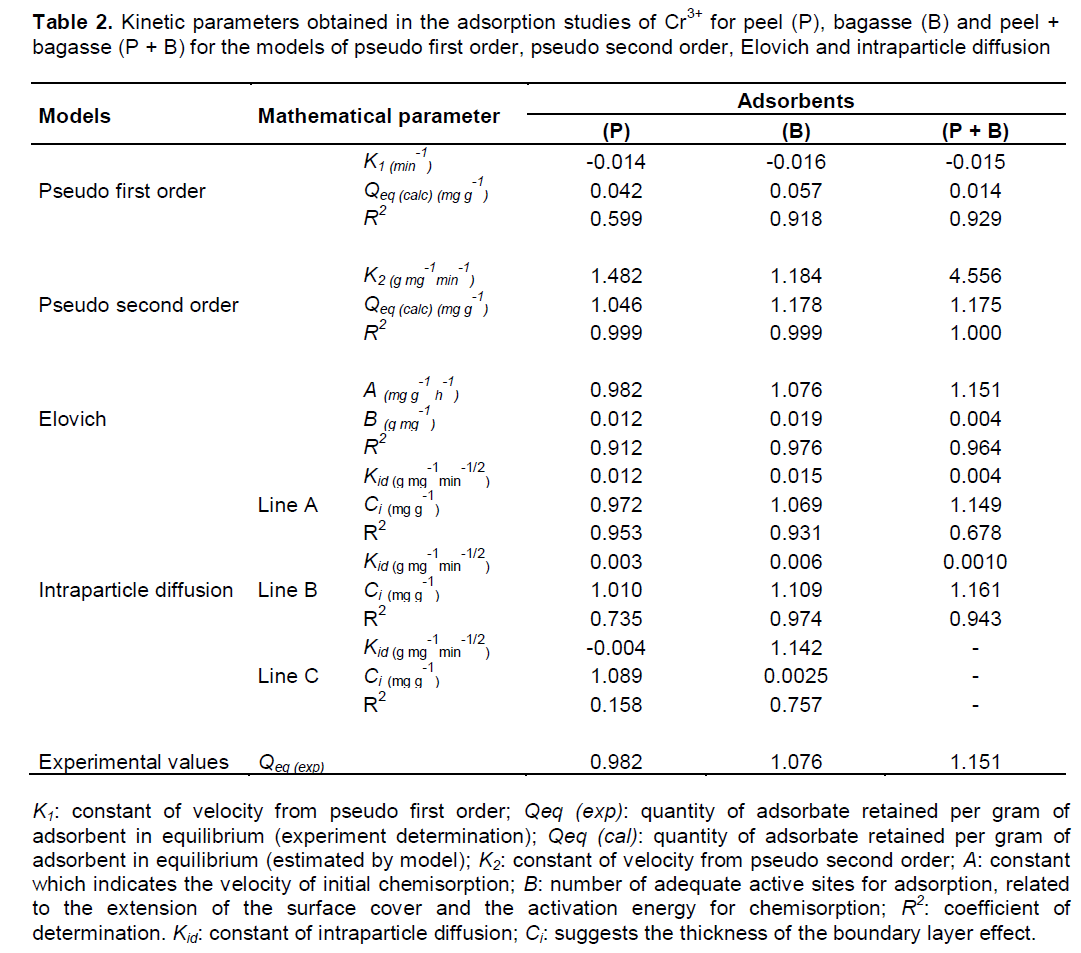
According to Farooq et al. (2011), the pseudo first order model do not fit in most of the biosorption systems, what explain why this model does not presented satisfactory fitting in the present study. However, the results of R2 and Qeq calculated by the pseudo second order are satisfactory, suggesting a chemisorption of Cr3+ by the materials ‘P’, ‘B’ and ‘P + B’, as observed in Table 2. The model of Elovich dos not explain the behavior observed by the adsorbents (Table 2), in function of the lower values of R2 obtained. When observed the values of R2 for the intraparticle diffusion (Table 2), it is verified a multilinear division, this was performed in order to find some step of adsorption which is conducted by the model of intraparticle diffusion. However all obtained R2 values found were unsatisfactory.
Influence of initial concentrations of Cr3+
The adsorption isotherms describe the relation between the ion adsorbed quantity by adsorbent mass related to the solution concentration in dynamic equilibrium (Witek-krowiak et al., 2011), being these information important for the interpretation of biosorption process. It is observed in Figure 5 high adsorption rates for cassava biosorbents, being these values very close to the adsorption rates obtained for active coal. It is worth pointing out the obtaining of active coal is from abrupt physical-chemical structure modifications, which result in a higher cost of production, while the natural adsorbents such cassava adsorbents (P, B and P + B) present low cost and high availability in most tropical countries.
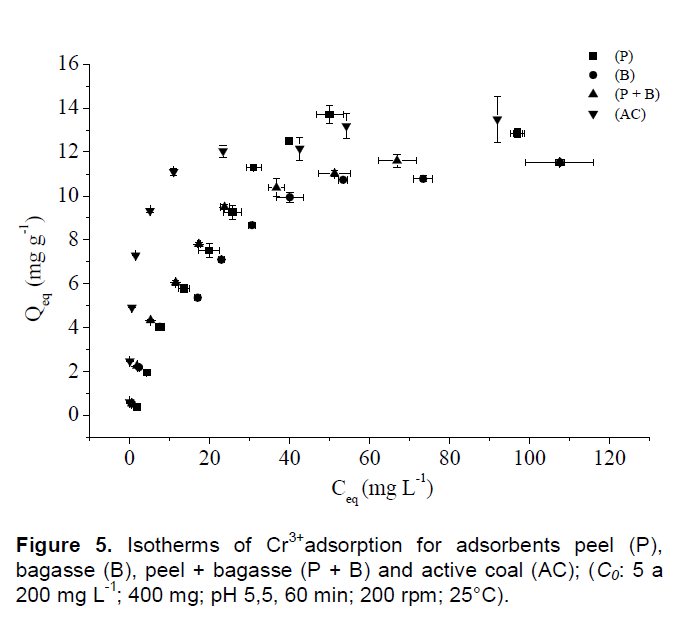
It was observed that the efficiency in the removal by the biosorbents decrease rapidly with the increase of C0, very similar behavior found with active coal (AC). It is important to emphasize that AC is an adsorbent obtained by abrupt physical-chemical modifications, that is, it is more expensive in the monetary point of view (Girods et al., 2009), however presenting lower adsorption rates than cassava biosorbents in higher C0, such as concentrations of Cr (III) higher than 40 mg L-1. It worth point out that in solutions contaminated with Cr3+ in C0 higher than 140 mg L-1, the natural adsorbents present themselves efficient as active coal (Table 4; Figure 5).
In Figure 5 it is noted a lower efficiency of Cr3+ adsorption in lower C0 by the natural adsorbents when compared to active coal (AC), however in higher C0 (higher than 140 mg L-1) the natural adsorbents presented high efficiency as active coal (AC). As observed in Figure 5, the obtained isotherms for adsorbents presented typical behavior of isotherms from “L group” (Langmuir type), with initial curvature facing down, due to the decrease of availability of active sites (Giles et al., 1960).
It was also identified in Figure 6 isotherms from “L group” (Langmuir type) and subgroup “2”, what indicates the occurrence of surface saturation which the adsorbate has more affinity by the solvent than adsorbed molecules (Giles et al., 1960).
For C0 until 80 mg L-1 of Cr3+, the active coal (AC) is the best material, adsorbing great quantities of this ion, however, in concentrations superior of this, in function of active sites saturation, this material chemically modified become equally efficient to the cassava natural adsorbents. The parameters obtained for linearization of adsorption isotherms for peel, bagasse, peel + bagasse and active coal in removal of Cr3+ are shown in Table 3.
The materials ‘B’, ‘C+B’ e ‘AC’ presented better fitting by Langmuir, suggesting adsorption of Cr3+ in monolayer.In relation to Langmuir parameters (Table 3), the max adsorption quantity (Qm) presented satisfactory results for the cassava adsorbents, with values very close to those obtained for active coal (AC).
Evaluating the values of “RL” of Langmuir (Table 3), it can be stated that in the experimental conditions the adsorptive process is favorable for all cases, once “RL” values shown between ‘0’ e ‘1’ (Lin and Juang, 2002).
According Sodré et al. (2001), the “n” parameter from Freundlich indicates the reactivity of active sites, when this variable assume values superior than 1, this is a strong indication of the presence of highly energetic sites, suggesting that these sites are occupied by Cr3+ in first place. This behavior of high energetic interaction and high reactivity it is observed in Table 3 for biosorbents “P”, because in this case the model of Freundlich present satisfactory fitting.
The model of D-R explains satisfactorily the adsorption of Cr3+ by the natural adsorbents (Table 3). According to Wan Ngah and Hanafiah (2008), the average sorption energy (E), related to the mathematical model of D-R, is the free energy evolved in the transfer of 1 mol of solute for surface of adsorbente, this parameter suggests the reaction character as chemical or physical adsorption. Values of “E” between 1 and 8 kj mol-1 indicates physical adsorption, whereas values above 8 kj mol-1 indicates chemical nature of the adsorption process (Romero-Gonzalez et al., 2005; Fávere et al., 2010). In Table 3, the values of E in most cases assume values above 8 kj mol-1, suggesting predominance of chemisorption for biosorbents, exception only for material “P” in Cr3+ adsorption, with values of E inferior to 8 kj mol-1.
Thermodynamics of adsorption
For the comprehension of the temperature effect in the process of Cr3+sorption by the biosorbents and to analyze the nature that rules this process, some thermodynamic parameters were evaluated, as is shown in Table 4. According to Qeq values observed in Table 4 for biosorbents (P, B and P + B), it is noticed the occurrence of a gradual increase in Qeq values, what evidence the influence of temperature in the biosorption process of Cr3+. As shown in Table 4, the values of ΔH are positive, indicating an endothermic system (Wan Ngah and Fatinathan, 2010).
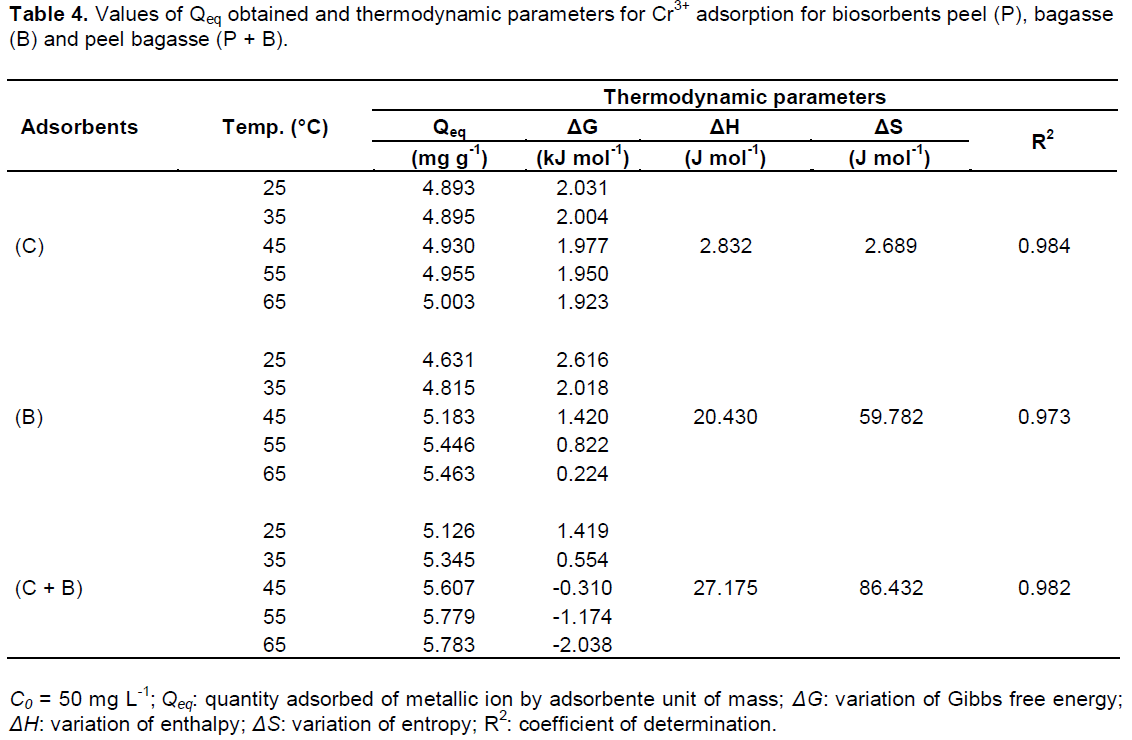
According to Crini and Badot (2008), the laws of thermodynamics indicate that at constant temperature and pressure, the value of ΔG is the main criteria for indication of spontaneity of the system. According to Wan Ngah and Hanafiah (2008), negative values for ΔG indicate the spontaneous nature of the reaction, while positive values for ΔS indicate the increase of the disorder and randomness of the interface solid/solution during the sorption process, as occurred for the evaluated biosorbents (Table 4). By the obtained results, higher temperatures result in higher adsorption of Cr3+, however the adsorption process is not usually operated at higher temperatures, because this would increase the operational costs (Crini and Badot, 2008).
Characterization of the adsorbent´s functional groups after adsorption
The infrared spectrum after the adsorption of Cr3+ was conducted in order to observe which were the possible functional groups responsible for adsorption process (Figure 6). The bands comprehended at 1730 cm-1 still indicate the starch presence and carbonyl groups present in lignin and holocellulose (Horn et al., 2011).
The bands at 1420 to 1650 cm-1 are still present in the materials peel (P) and peel + bagasse (P + B), suggesting the presence of amides and carboxylic groups from lignin molecules (Han et al., 2010). However, for the material bagasse (B) the bands at 1420 to 1030 cm-1 are missing (dash area at Figure 6 right) or with a decrease of transmittance percentage, suggesting that these adsorption sites were responsible for the binding with Cr3+ (Argun and Dursun 2008), probably carboxyl groups (C-O) (Han et al., 2010) and lignin (Guo et al., 2008).
Desorption
According to Mimura et al. (2010), the desorption corresponds to removal of the metal from the binding site from the adsorbent surface and it is expected that H+ ions can replace the adsorbed cations by the ionic exchange mechanism. The recovery of the adsorbed metal can be performed by many procedures as, for example, the desorption process. This practice is fundamental for the knowledge of characteristics of interaction between adsorbate and adsorbent concerning to its resistance for reuse in new adsorption processes.
Desorption results for biosorbents and activated coal reveal Cr3+ low desorption rates, especially for biosorbents. Results may be explained by the fact that Cr3+ adsorption by cassava materials appear to be a chemical process.
According to Namasivayam et al. (1998), low desorption rates generally suggest that the adsorbate is strongly linked to the adsorbent´s active sites by chemisorption. It must be underscored that an increase in HCl concentration or the use of other compounds, such as nitric acid (HNO3), sulfuric acid (H2SO4) and sodium hydroxide (NaOH) or even complexant EDTA to promote desorption may provide better results (Sekhar et al., 2004; Bernardo et al., 2009).