ABSTRACT
There are concerted efforts in promoting cassava production in sub Saharan Africa as a staple food crop as well as income earner at both household and commercial level. These efforts can bear meaningful results if breeding strategies are properly coordinated. Efficient breeding methods require a thorough understanding of the genetic background of planting materials. Therefore, a genetic study was conducted to establish the genetic basis of cassava genotypes for root weight, root number/plant, root length (cm), above ground weight, harvest index, cassava mealy bug and cassava green mite by mating six cassava genotypes using a diallel method. The generated F1s were evaluated using a randomized complete block design (RCBD) replicated thrice. The analysis of variance revealed highly significant (P< 0.001) genetic variation among the genotypes for all the traits except for cassava mealy bug, indicating the presence of high variability among genotypes. Thus, selection among these genotypes could lead to good progress for the improvement of the target traits. Mean squares for both general combining ability (GCA) and specific combining ability (SCA) were highly significant for all traits except for harvest index and cassava green mite for GCA and SCA respectively which suggested involvement of additive and non-additive component of heritable variance in the inheritance of all these traits. However, the proportions of additive variance to the total genetic variances (that is, 2δ2gca/(2δ2gca + δ2sca) showed that crossing for these traits and making selection from such crosses, about 41 to 100% of the improvement would be expected to come from SCA and less than 59% from GCA which indicated a greater role played by non-additive genes. Overall, results indicated that it would be readily possible to breed for high yield from this set of genotypes.
Key words: Breeding, combining ability, gene action.
The importance as well as challenges of cassava production are well known worldwide. The acclaimed advantages accrued to cassava production include staple food crop and source of income for the rural communities, industrial raw material, and earner of foreign exchange (Cock, 1985). Furthermore, cassava is regarded as a ‘‘low risk crop’’ as it adapts readily to a wide range of agro ecological conditions, utilizes efficiently mineral reserves of marginal soils, withstands climatic variations and is highly efficient in the conversion of solar energy to starch. In addition, cassava has low requirements of inputs like fertilizers, its flexibility in planting and harvesting, convenient in ground storability, diversified modes of utilization and higher dry matter yield per hectare (Westby, 2002; Ministry of Agriculture, Irrigation and Food Security [MoAFS], 2007). Thus, cassava has tremendous potential for future exploitation to contribute to food security, rural income, and the economy in the African continent. However, major threats of cassava in terms of yield and quality are pests and diseases infestation (Mahungu et al., 1994). The major insect pests are cassava mealy bug (CMB) and cassava green mite (CGM) while cassava mosaic disease (CMD), cassava bacterial blight (CBB) and cassava brown streak virus disease (CBSD) are the important diseases (Dixon et al., 2003). These diseases can affect plant establishment and vigour, inhibit photosynthetic efficiency and cause pre-harvest or postharvest deterioration. Severe occurrences of both insect pests and diseases often lead to a considerable loss in yield potential of cassava. Insect pests can cause damage to cassava by reducing photosynthetic area, damaging stems, which inhibit nutrient transport causing low production (IITA, 1990). Combining ability is the relative ability of an inbred line or a clone, when crossed to another inbred line or clone, to transmit desirable traits or specific trait to the next generation (Chaudhari, 1971). It helps to predict the performance of a particular line when used as a parent in a hybrid and facilitates the selection of superior parents for hybrid combination and for studying the nature of genetic variation (Russel, 1985). It is reported that Griffing (1956) introduced a method of analyzing combining ability using the genetic estimates of the parent and hybrid components of diallel analysis through general and specific combining ability. Falconer and Mackay (1996) defined general combining ability (GCA) as the mean performance of the line in all its crosses and it is expressed as a deviation from the mean of all crosses. This average performance of parents in crosses GCA, estimates the breeding value of a given genotype due to additive gene effects (Ceballos et al., 2004). Specific combining ability (SCA) is defined as the deviation of individual crosses from the average performance of parents, and this is due to dominance effects. Understanding of gene action would be useful for the formulation of breeding strategies to improve desired traits. Therefore, information on combining ability is needed in order to identify suitable parents (among the following; Mulola, 01/1313, Depwete, 01/1316, Silira and Maunjili) and superior genotypes which can be hybridized for the development of elite cultivars and hybrid varieties that would ultimately ensure sustained production and productivity by smallholder farmers in Malawi.
The present study was conducted during 2011 to 2012 season at Chitala Agricultural Research Station. Chitala Agricultural Research Station lies on latitude 13°40’ South and on longitude 34°15’ East. It is at an altitude of 606 m above sea level. The station is on the lakeshore areas of Malawi and therefore has hot weather with mean annual temperatures of 28°C maximum and 16°C minimum. The soils are sandy clay to clay with the pH range of 5.8 to 6.4 (Figure 1).
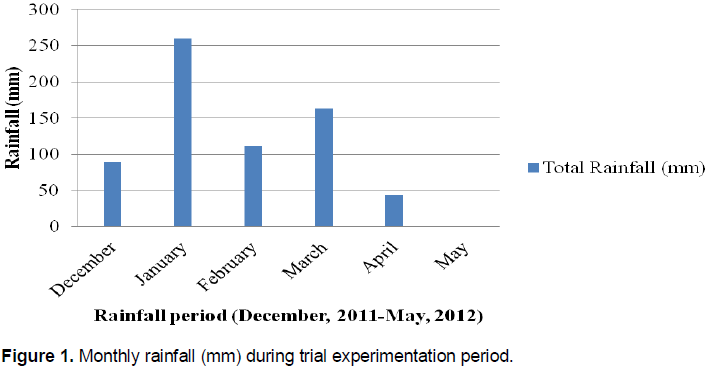
Plant materials
Six cassava genotypes namely, Mulola, 01/1316, 01/1313, Depwete, Silira and Maunjili and their F1 derived through half diallel during 2009 to 2010.
Seedling evaluation trial
Seeds were germinated and grown on 9th November, 2010 in a glasshouse in floating trays at Chitedze Agricultural Research Station. The water was given twice a day to ensure good germination and development. Two months after sowing, the seedlings were transplanted on 13th January, 2011 for field evaluation in a seedling trial. The seedlings were planted at spacing of 90 × 50 cm. Parents (Mature stems 25 cm long) were also planted at the same spacing but 20 plants per genotype/variety of each parent. No irrigation and fertilizers were applied at this stage.
Clonal evaluation trial
Fifteen crosses and six parents were used in the clonal trial. Twenty plants were selected from each cross. The selection of plants per cross from seedling field trial was based on the ability of the plant to produce six good vegetative cuttings to proceed with clonal evaluation. A randomized complete block design with three replicates was used to plant a total of twenty one entries consisting of fifteen F1s and six parents. The planting date was 14/01/2012. Each replication contained twenty one entries (parents and crosses), planted together in the respective plots of each replication. The plant spacing was 1 m between rows and 0.90 m within rows. The field was weeded manually and not irrigated or fertilized.
Agronomic characters measured
During the growing period, data was collected on CGM and CMB which were scored quarterly at 3, 6, 9 and 12 months after planting (MAP) using a scale of 1 to 5, where, 1 means no visible symptoms while 5, severely attacked (IITA, 1990). At harvest, 12 MAP, the individual plants were assessed for their number of storage roots per plant, root weight (kg per plant), above ground weight (kg/plant), root length (cm) and harvest index. Plants were hand-harvested individually and the results were averaged across plants. The roots were counted and weighed separately.
Data analysis
Data was analysed using SAS version 9.3 (
SAS Institute Inc, 2011). Different analytical tools were employed and these included general analysis of variance, analysis of variance for combining ability and estimates of genetic parameters.
General analysis of variance
The general analysis of variance was used to test if the sources of variation had any significant influence on the characters under study using the following model:
Yijk = µ + Τij + Bk +Eijk
Where: Yijk=observation of the ij cross in block k, µ =overall mean associated with the data, Tij =effect of the cross ij, Bk=effect of block and Eijk=effect of the random error associated with the ij cross in block k.
Combining ability effects
GCA effects and SCA effects were estimated according to Griffing (1956) model 1 method 2 using DIALLEL-SAS05 program developed by Zhang et al. (2005). Griffing’s fixed model for the second method is presented below as outlined by Mayo (1987).
Yij = µ + gcai + gcaj + scaij + Εij;
Where: Yij = is the observed response, µ = is population mean, gcai = the general combining ability (GCA) of the ith parent, gcaj = the general combining ability (GCA) of the jth parent, scaij = the specific combining ability (SCA) associated with the ith and jth cross and Εij = the error associated with each observation.
Test of significance for combining ability effects
Significance of combining ability effects were determined by using
t- test at 0.05, 0.01 and 0.001 levels of probability.
t (calculated) for GCA effect =

Standard error (S.E) was adapted from the combining ability analysis of variance output. The distribution of crosses in relation to GCA and SCA effects was determined by denoting significant positive combining ability effects as high, non-significant as average and significant negative as low for average root number per plant, fresh root weight (kg/plant), above ground weight (kg/plant), root length (cm) and harvest index. For CGM, significant positive combining ability effects were considered as low, non-significant as average and significant negative as high (Saleem, 2008). GCA and SCA values with negative effects show contribution towards resistance, and positive values show contribution towards susceptibility for CGM (Owolade et al., 2008). Baker’s prediction ratio (PR) (Baker, 1978) was used in determining progeny performance, that is,
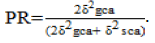
The closer this ratio is to one the greater the chances of predicting progeny performance based on GCA, that is, value less than 1, was taken as predominance of non-additive type of gene action, and greater than 1 as additive gene action.
The analysis of variance revealed highly significant (P<0.001) genetic variation among the genotypes for all the traits except for cassava mealy bug, indicating the presence of variability among genotypes (Table 1). High variability for different characters had also been reported in cassava (Munga, 2008; Da Silva, 2008; Mtunda, 2009; Akinwale et al., 2010; Kamau et al., 2010; Parkes, 2011). This implies that selection among these genotypes could lead a good progress for the improvement of the target traits.
.
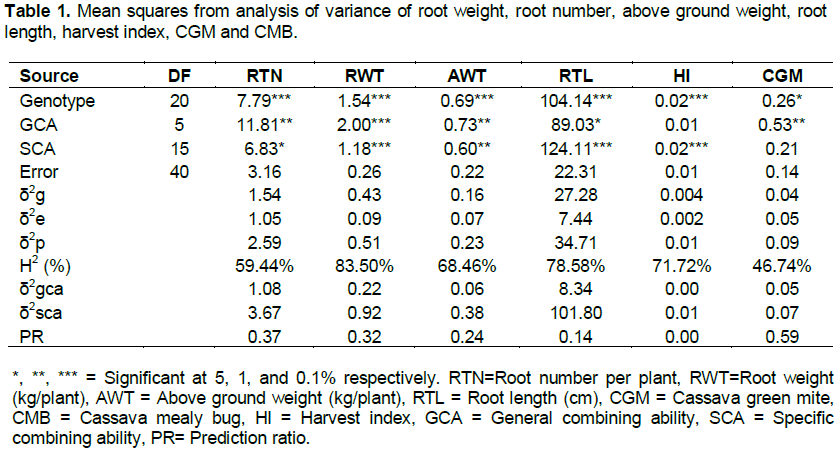
The significant values of mean squares for GCA and SCA for all traits except harvest index and cassava green mite were observed (Table 1). The significant differences among the GCA and SCA effects suggested the involvement of additive and non-additive component of heritable variance in the inheritance. GCA and SCA variance components were used to determine their relative importance in predicting progeny performance for the traits under study. The general combining ability variances (δ2gca) for all the traits were lower than specific combing ability variances (δ2sca) (Table 1) which indicated that SCA was more important in predicting progeny performance for expression of the traits. It corroborates with the findings of Da Silva (2008) and Parkes (2011) wherein higher SCA variances than GCA variances in cassava for various traits was reported and concluded that SCA was more important in predicting progeny performance.
To determine the relative importance of GCA and SCA in the genetic control of the different traits, as well as the expected amount of improvement from GCA and SCA, the proportions of additive variance to the total genetic variances were calculated (that is, PR= 2δ2gca/(2δ2gca + δ2sca) (Table 1). The ratios ranged from 0 to 0.59. It suggests that crossing for these traits and making selection from such crosses, about 41 to 100% of the improvement would be expected to come from SCA and less than 59% from GCA. Thus by far the largest part of the improvement for these traits comes from SCA and ultimately from non- additive genes. In other words, since the prediction ratio (PR= 2δ2gca/(2δ2gca + δ2sca) for all the traits was less than 1, it means that non additive gene effects were more predominant in the expression of the studied traits. Therefore, the study suggests that an efficient cassava breeding program should be based on breeding strategies that exploit dominance effects.
Similarly, the preponderance of non-additive gene effects for fresh root weight have been reported earlier (Da Silva, 2008; Kamau et al., 2010; Parkes, 2011). However, the predominance of additive gene effects for average number of roots was reported by Da Silva (2008), Mtunda (2009) and Kamau et al. (2010) which contradicted our findings.
General combining ability effects quantitatively measure the comparative performance of parents in relation to one another. Therefore, GCA effects were computed to show both the magnitude and direction of the genetic effects (Table 3). GCA effects for average root number showed significant positive values for Mulola (0.87) and 01/1316 (0.75), which means that these genotypes contributed most for the high root number so these were the superior genotypes for this particular trait, while 01/1313 had significant negative value (-1.00), which means it performed below average of this set of parents. Mulola and Maunjili were the good general combiners for fresh root weight with significant positive values. For above ground weight, Mulola was the best general combiner as it exhibited a significant positive value (0.20).
Silira proved to be the best combiner for root length as shown by a significant positive value (2.78) while 01/1313 performed below average of this set of parents as shown by a significant negative value (-3.57). Among the six parents, Maunjili performed above average harvest index with a significant positive GCA effect of 0.04 while 01/1313 and Silira exhibited significant negative GCA effect of -0.02. The estimates of GCA effects for CGM showed that Maunjiri had a significant negative value while Silira had a significant positive value. Therefore, Maunjili can be a desirable parent for hybrids as well as for inclusion in breeding program aimed at developing varieties resistant to CGM due to its significant negative value (Table 3). The foregoing suggestion is in tandem with the report by Owolade et al. (2008) and Da Silva (2008). The study has found that the best rated general combiners based on GCA effects were Mulola for average root number, fresh root weight and above ground weight and Maunjili for fresh root weight, harvest index and cassava green mite which means that these could be good parents to use when breeding for genotypes for the said traits. 01/1313 was the worst combiner for all the traits studied except cassava green mite.
The highest magnitudes of significantly positive and desirable SCA effects for average root number were recorded on four crosses (Table 4). The results also indicated that two hybrids had significant negative SCA effects. On average, 50% of the hybrids were desirable for the development of cassava genotypes with large number of roots. The distribution of hybrids based on their parental GCA effects revealed that best hybrids emerged from parents with varying levels of GCA effects such as Depwete × Maunjili (average × low parental combinations), Mulola × Silira (high × low), and 01/1316 × Depwete (high × average). These combinations suggest that best progenies might not only be derived from crosses with genotypes having the greatest positive GCA effects. This suggests that these hybrids could be exploited for heterosis in a breeding program in order to develop genotypes with high number of roots. Several crosses exhibiting significant SCA effects for average root number per plant in cassava have been reported in previous studies (DaSilva, 2008; Mtunda, 2009; Kamau et al., 2010).
Five of 21 crosses exhibited significant SCA effects for fresh root weight (Table 4). Of these, SCA effects were in desirable positive direction for two crosses, and in undesirable negative direction for three crosses. The distribution of crosses in relation to GCA effects of parental combinations revealed that almost all types of SCA effects were derived from any type of GCA values. For example, Mulola × 01/1316 obtained from (high × low), Depwete × Maunjili (average × high), parental combinations. Therefore, the performance of hybrids was independent of parents’. A similar trend was observed in several previous studies (Da Silva, 2008; Mtunda, 2009; Kamau et al., 2010). Based on mean performance (Table 2) and SCA effects; two hybrids namely, Mulola × 01/1316 and Depwete × Maunjili were designated as best hybrids and are suggested for hybrid vigour breeding.
For above ground weight, thirteen crosses had positive GCA effects but only one had a significant positive value (Silira × Depwete). On the other hand, two crosses exhibited significant negative values for above ground weight which means that these two (Table 4) were the most undesirable crosses for this trait. The most desirable cross for this trait was derived from average × average parental combination.
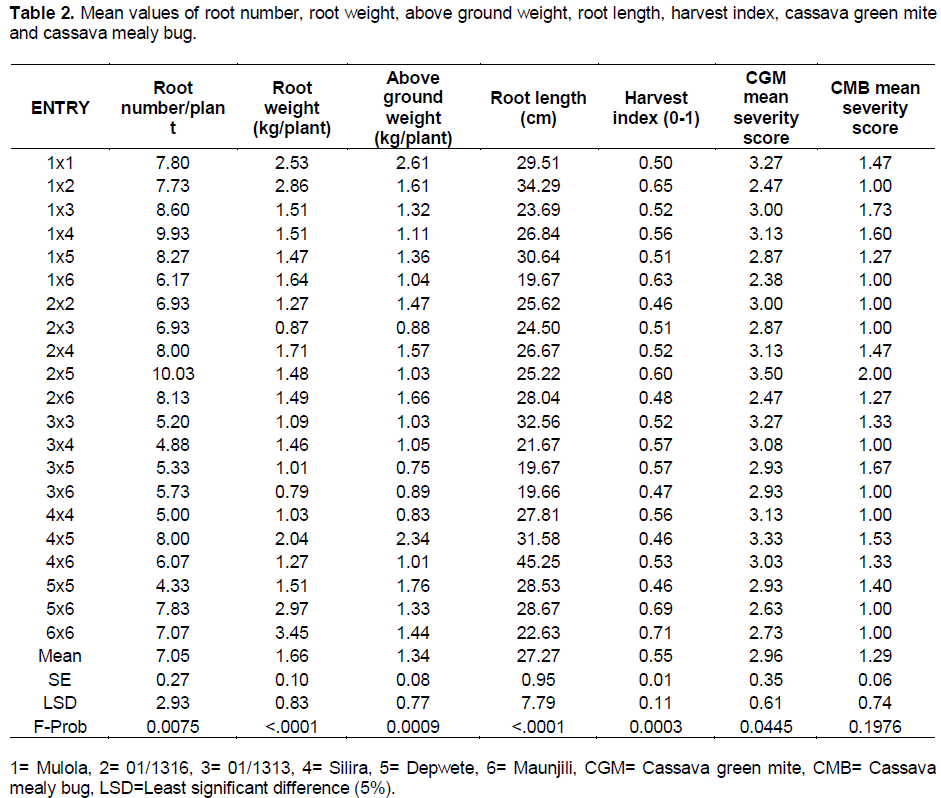
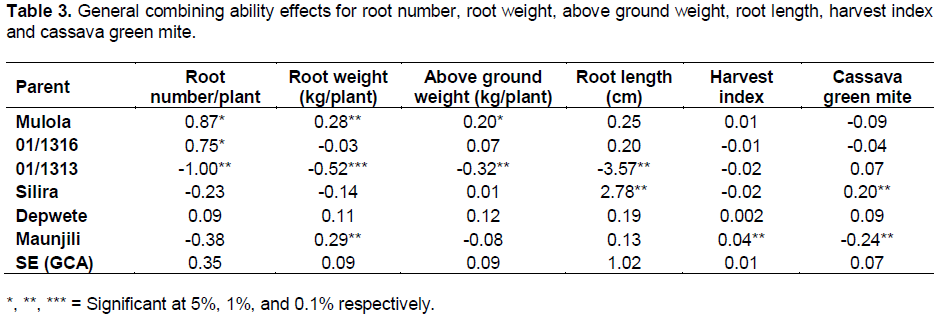
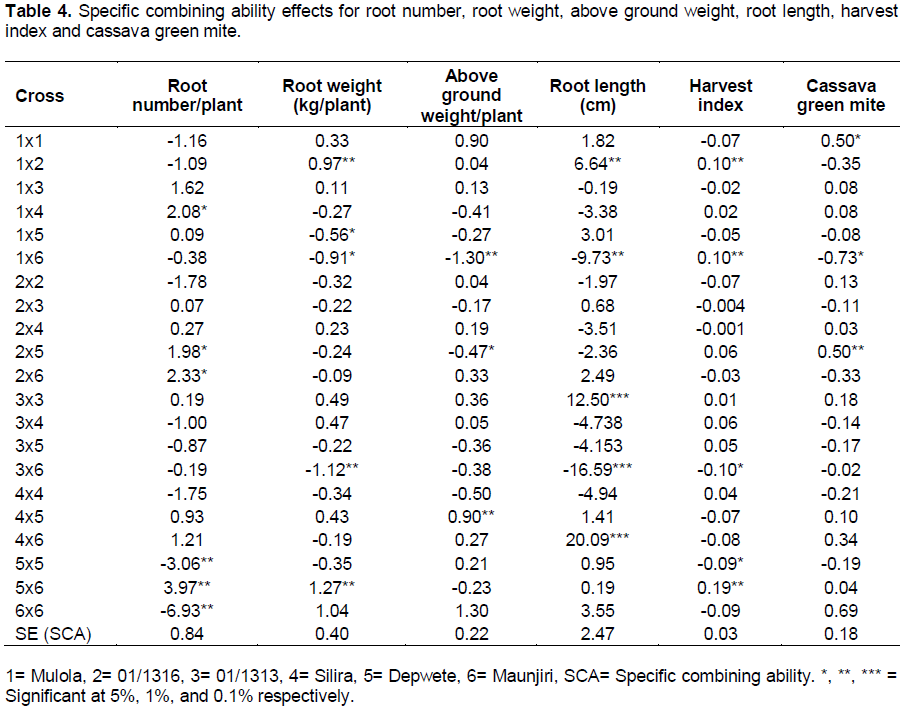
Magnitude and direction of SCA effects of crosses for root length were variable ranging from significant (five crosses) to non significant and negative to positive values (Table 4). The highest desirable SCA effects were observed on Silira × Maunjili (20.09), followed by 01/1313 × 01/1313 (12.50). Of the five significant crosses, two were in the undesirable direction, the worst being 01/1313 × Maunjili (-16.59). The distribution of the crosses in relation to the GCA effects of the parents showed that the best cross (Silira × Maunjili) emerged from high × average GCA parental combination and the second best cross (01/1313 × 01/1313) emerged from low × low parental combination. This means that the best crosses are not necessarily derived from the best general combiners.
The best SCA effects were recorded on three crosses for harvest index (Table 4), namely, Depwete × Maunjili (0.19), Mulola × Maunjili (0.10) and Mulola × 01/1316 (0.10). These best crosses were derived from any parental combination such as average × low (Mulola × 01/1316), and average × high (Depwete × Maunjili).
The crosses manifested varying degrees of resistance to CGM (Table 4). One cross (Mulola × Maunjili) exhibited desirable significantly negative SCA effects for this trait while two crosses recorded significantly positive SCA effects. One of the parents in the desirable cross (Maunjili) was the best general combiner for this trait.
The performance of the crosses with respect to the parental genotypes suggests that progeny performance cannot largely be dependent on performance of parents per se. Therefore, these results are consistent with previous studies by Ceballos et al. (2004) that not only additive effects are important in determining the performance of derived progenies but also that there is a large component of dominance effects that translate into significant heterosis for traits such as fresh root yield of cassava, and CIAT (1987) that any breeding method in cassava should maintain heterozygosity and take into account both additive and non-additive genetic variance. Ceballos et al. (2004) and Lebot (2009) argued that since cassava is a highly heterozygous crop, dominance effects are likely to play an important role in the performance of materials being selected and any breeding program should exploit dominance effects because, once an elite clone is identified, it can be propagated vegetatively thereby carrying along the dominance effects. This however, does not preclude the actuality that the predominance of the additive gene effects imply that the best progeny might be derived from crosses with genotypes having the greatest GCA effects (Arunga et al., 2010).
Significant GCA and SCA effects indicated importance of both additive and non-additive gene action in controlling the traits studied. Predominance of SCA effects, however, highlighted the greater role of non-additive relative to additive gene action. Overall, results indicated that it would be readily possible to breed for high yield from this set of genotypes.
The authors have not declared any conflict of interest.
Authors are grateful to the Alliance for a Green Revolution in Africa (AGRA) for supporting this research.
REFERENCES
Akinwale MG, Aladesanwa RD, Akinyele BO, Dixon AGO, Odiyi AC (2010). Inheritance of ß-carotene in cassava (Manihot esculenta Crantz). Int. J. Genet. Mol. Biol. 2(10):198-201. |
|
Arunga EM, Van Rheenen HA, Owuoche JO (2010). Diallel analysis of Snap bean (Phaseolus vulgaris L.) varieties for important traits. Afr. J. Agric. Res. 5(15):1951-1957. |
|
Ceballos H, Iglesias CA, Pe'rez JC, Dixon AGO (2004). Cassava breeding: Opportunities and challenges. Plant Mol. Biol. 56:503–516.
Crossref |
|
CIAT (Centro Internacional de Agricultura Tropical) (1987). Cassava breeding: Multidisciplinary review. Proceedings of a workshop held in the Philipines, 4-7 March, 1985. Clair H. Hershey (tech. Ed.). Cali, Colombia: Centro Internacional de Agricultura Tropical. |
|
Chaudhari HK (1971). Elementary principles of plant breeding, (2nd ed.). New Delhi, India: Oxford and IBH Publishing CO. |
|
Cock JH (1985). Cassava: New potential for a neglected crop. New York, USA: Westview Press. |
|
DaSilva AMZ (2008). Breeding potential of cassava (Manihot esculenta Crantz) in Mozambique (PhD Thesis). University of the Free State, South Africa. |
|
Dixon AGO, Bandyopadhyay R, Coyne D, Ferguson M, Ferris RSB, Hanna R, Ortiz R (2003). Cassava: From Poor Farmers' Crop to Pacesetter of African Rural Development, 3. Leuven, Belgium: International Society for Horticultural Science. |
|
Falconer DS, Mackay TFC (1996). Introduction to quantitative genetics, (4th ed.). England. UK: Longman Group Ltd. |
|
Griffing B (1956). Concept of general and specific combining ability in relation to diallel crossing systems. Austr. J. Biol. Sci. 9:463-493. |
|
IITA (International Institute of Tropical Agriculture) (1990). Cassava in Tropical Africa. A Reference Manual. London, UK: Chayce Publication Services, P. 176. |
|
Lebot V (2009). Tropical Root and Tuber Crops, cassava, sweet potato, yams and aroids. London, UK: CABI. P. 413. |
|
Kamau J, Melis R, Laing M, Derera J, Shanahan P, Ngugi E (2010). Combining the yield ability and secondary traits of selected cassava genotypes in the semi-arid areas of Eastern Kenya. J. Plant Breed. Crop Sci. 2(7):181-191. |
|
Mahungu NM, Dixon AGO, Mkumbira JM (1994). Breeding cassava for multiple pest resistance in Africa. Afr. Crop Sci. J. 2(4):539-552. |
|
Mayo O (1987). The theory of plant breeding, (2nd ed.). New York, USA: Oxford university press. |
|
Ministry of Agriculture, Irrigation and Food Security (2007). Cassava and Sweet potato Production Handbook, Lilongwe, Malawi: Ministry of Agriculture, Irrigation and Food Security. |
|
Mtunda KJ (2009). Breeding, evaluation and selection of Cassava for high starch content and yield in Tanzania. Ph.D. Thesis, University of Kwazulu-Natal, Pietermaritzburg. |
|
Munga TL (2008). Breeding for cassava brown streak disease resistance in coastal Kenya (PhD thesis). School of Agriculture and Agribusiness, University of KwaZulu-Natal, Pietermaritzburg, South Africa. |
|
Owolade OF, Dixon AGO, Alabi BS, Akande SR, Olakojo SA (2008). A combining ability analysis of cassava Manihot esculenta Crantz genotypes to anthracnose disease. Electr. J. Environ. Agric. Food Chem. 7(6):2959-2968. |
|
Parkes EY (2011). Assessment of genetic diversity, combining ability, stability and farmer preference of cassava germplasm in Ghana (PhD Thesis). University of the Free State, South Africa. |
|
Russel GE (ed.) (1985). Progress in plant breeding-1. London, UK: Butterworth and Co. Ltd. |
|
Saleem MY (2008). Genetic analysis of Basmati Rice (Oryza sativa L.) (PhD Thesis). Institute of Pure and Applied Biology, Bahauddin Zakariya University, Multan, Pakistan. |
|
SAS Institute Inc. (2011). Statistical Analysis Software/Stat Software, Version 9.3. 2nd ed. SAS Institute Inc., Cary NC North Carolina, USA. |
|
Westby A (2002). Cassava utilization, storage and small-scale processing. In RJ Hillocks, JM Thresh and AC Bellotti (Eds.). Cassava: Biology, production and utilization (pp. 281-300). London, UK: CABI Publishing.
Crossref |
|
Zhang Y, Kang MS, Lamkey KR (2005). DIALLEL-SAS05: A Comprehensive Program for Griffing's and Gardner–Eberhart Analyses. Agron. J. 97:1097–1106.
Crossref |