ABSTRACT
The study evaluated the chemical stabilization of soils with cement toward application in improving forest roads, as constructive alternative of low cost. The study was carried out with fine soils from the region of Niquelândia-GO, where the sand-clay-silt particle proportion was predominant, and Portland cement CP-II-Z-32 used as a stabilizing agent at 2% under dry soil weight. Geotechnical tests were conducted in different compaction energies (normal, intermediate and modified) and curing periods (1, 7 and 28 days), and the data were analyzed statistically by Analysis of Variance (ANOVA) and Tukey test. The soil-cement mixture presented higher unconfined compressive strength (UCS) than the compacted local soil, reaching a highest value of 650.52 kPa on modified compaction energy. Also, California bearing ratio (CBR) with values ​​of 44.1% in the intermediate compaction energy and 41.7% in the modified compaction energy reached higher values compared to lateritic soil sampled. Soil-cement mixture was prescribed as improved layer for forest road surface, as well as reached indication for subgrade reinforcement material and sub-base in conventional paved roads.
Key words: Forest management, chemical stabilizing, lateritic soil, unpaved roads.
Road infrastructures for forest activities are complex engineering structures that involve design, building, and maintenance (Sessions, 2007). The technical constraints for planning and building a forest road network are the condition and type of traffic, terrain, climate and building standard. Also, importance should be given to soil type, especially for its availability and bearing capacity as material of road construction (Machado and Malinovsky, 1986; Nogami and Villibor, 1995).
Forest roads serve a single purpose and have a distinct function from other roads. The low traffic volume of long and heavy trucks in one direction characterizes these routes. In addition, they have differentiated structure in their cross section, with two almost in distinct layers, which are the surface or natural soil, and an improved layer, which should support the demands of forest transport and resist hazardous weather (Sessions and Heinrich, 1993).
Dias Junior et al. (2005) pointed out the vehicle and machinery traffic in the wood harvest and transport operations, and the low constructive pattern of forest roads as constraints to achieve sustainable forest development. For the authors, the improvement in the conditions of these roads, seeking greater durability and bearing capacity, represents an important component for economic sustainability of forestry undertakings. Forest road infrastructure should include economic viability in addition to technical parameters, prioritizing the acquisition of materials suitable for road construction with longer service life, minimizing maintenance costs of roads and forest transport vehicles and ensuring the efficient flow of forest goods.
The expansion of forest activities and increase in wood demand have required a forest road network of good quality, which is drivable all year round and has longer service life (Sessions, 2007). However, in many situations, the problem is in the local soil, which does not have the conditions required for roadwork for being little resistant, very compressible or having characteristics that fall short economically (Trindade et al., 2008). For Ingles and Metcalf (1972) this fact leads to the need to develop stabilization processes which make it possible to improve certain geotechnical properties of these soils, especially at a regional level, in order to fit them within the technical specifications applicable to the paving of roads.
Soil stabilization represents any artificial modification introduced in its behavior in order to make it employable in engineering works, acquiring a quantitative character through parameters inherent to certain design criteria, such as shear strength and deformation under the action of loads (Lima, 1981). From the road point of view, soil stabilization refers to the construction methods in which soils are treated so that they have surface, subbases and bases, occasionally coatings able to withstand traffic loads during the road life, resisting to the weather without degradation (Baptista, 1978). Mechanical and chemical stabilization techniques may be employed for improving the local soil properties in forest road construction. Mechanical stabilization is achieved by soil compacting, granulometric correction, and chemical stabilization, by adding luting materials to the soil.
Cement is used as a chemical stabilizer, and its addition to soil produces a mixture or material known as soil-cement. The results of the chemical processes of soil, cement and water are decreased porosity, plasticity and increased mechanical strength and durability (Kézdi and Rétháti, 1988). Small cement content, of the order of 1 to 2% is sufficient to reduce volume changes and increase the soil bearing capacity (Ingles, 1968). Portelinha et al. (2012) mentioned that the addition of 2 and 3% of lime or cement to laterite soil was sufficient to alter its workability characteristics and mechanical strength for road paving purposes.
Cement addition becomes an attractive technique when the design requires improving the local soil. Cement and local soil can be applied in the construction of pavement foundations, in the earth dam slope protection, and as a support for the layer of shallow foundations (Consoli et al., 2007). However, it is essential to carry out experimental programs in laboratory to get to know the mechanical behavior of soils when adding cement.
The objective of this study was to investigate in the laboratory the technical potential of cement use in improving geotechnical characteristics of a local soil from the surface of an unpaved forest road, as an economic alternative for the construction of flexible pavement layers.
Study area
The study was performed in a section of a forest road located in the municipality of Niquelandia, Goias State, Brazil (14°26’41”S; 48°44’26”W). This section was set up along of main access of a road network used for timber transportation by trucks loading about 25 tons. The origin and destination of the logs was the eucalyptus plantation (1566 ha) and iron-nickel alloy beneficiation plant, respectively, managed by Anglo American Company.
The materials used were lateritic soil, with sand-clay-silt particle proportion and classified by Brazilian Agricultural Research Corporation - Embrapa (2009) as an Oxisol (LVAd30) from the forest road and cement. Nineteen soil samples were collected, 45 m from each other, at a depth of 20 cm in three collecting points by sample, roadside and center, using a pick due to the high degree of soil compaction. Samples were packed in plastic bags, identified and sent to the Geotechnical Laboratory of University of Brasilia - UnB. The cement used was Portland CP-II-Z-32 of chemical composition clinker + gypsum (76 to 94%), limestone (0 to 10%) and pozzolan (6 to 14%). Before starting the laboratory tests, gross twigs, herbs, and organic debris were removed manually. Additionally, the soils were homogenized and sieved (2 mm mesh), as described in preparing sample methods for soil characterization tests (DNER – National Department of Roadways, 1994a).
Laboratory experimental program
In the laboratory, soil samples were first air-dried for over 72 h (ABNT, Brazilian Association of Technical Standards, 1984a) toward determining the following physical parameters: (a) Unit weight of soil grains (ABNT, 1984b); (b) Full grain-size distribution (ABNT, 1984c); (c) Liquidity limit (ABNT, 1984d); and (d) Plasticity limit (ABNT, 1984e). By knowing these parameters, the soil and the mixture were classified as to the geotechnical behavior by the American road system TRB (Transportation Research Board), according to National Department of Terrestrial Infrastructure (DNIT, 2006), and by the MCT Brazilian method (Miniature Compacted Tropical) according to Nogami and Villibor (1995).
The limits established by ABNT (1995) were adopted for the granulometric distribution of the soil fractions, which were: (a) clay: - f ≤ 0.002 mm; (b) silt: 0.002 mm <f ≤ 0.06 mm; (c) fine sand: 0.06 mm <f ≤ 0.2 mm; (d) medium sand: 0.2 mm <f ≤ 0.6 mm; (e) coarse sand: 0.6 mm <f ≤ 2 mm; and (f) gravel: f> 2 mm.
The soil-cement mixture was made by adding cement and water to the soil in pre-defined quantities to achieve the desired moisture contents, followed by hand homogenization and screening (# 4.8 mm). The mixture produced was wrapped in a plastic bag and sealed for later use in trials. The cement content was 2% calculated on the dry soil mass, based on minimum amount of material to be added for soil improvement by chemical stabilization according to National Department of Terrestrial Infrastructure (DNIT, 2006).
After making the soil-cement mixture, the following mechanical tests were performed: (a) Compaction (ABNT, 1986); (b) Unconfined compressive strength - UCS (DNER, 1994a), and (c) California bearing ratio - CBR (DNER, 1994b). These trials were also carried out for the local soil without addition of cement.
The determination of compaction curves and optimal parameters, maximum dry unit weight (gdmax) and optimal humidity (â±³opt) were performed according to the Proctor method. Proctor compaction was carried out through a dynamic process using a cylinder mold (10-cm internal diameter, 12.7-cm height and 1,000-cm³ volume). The compaction energies tested were normal, intermediate, and modified in quest of acceptable performance in terms of support capacity and low cost building for forest road application.
To determine the UCS, cylindrical soil proofs (f = 5 cm and height = 10 ± 0.05 cm) were molded in accord to compaction parameters (â±³opt and gdmax), applying static compaction by using an electrical press, with compaction degree of 0.3%. After preparation, the proofs were packaged, named and submitted to damp curing periods of 1, 7 and 28 days in a chamber with 100% humidity and temperature of 21°C. Three proofs were molded for each compaction energy and curing time. The proofs have been compressed yet break or point of maximum compression strength of the soil, using a simple compaction press at the speed of 1.143 mm/min. The UCS values were obtained by averaging the three breakdown tension points for energy and curing.
The values ​​of expansion and CSI were obtained from compacted mixture proofs based on the compaction parameters (â±³opt and gdmax), on the modified and intermediate energies and for the cure period with best response in the UCS trial. Compaction was performed in five inner layers with a socket of 4.5 kg, in California cylinder (f = 15.2 cm and height = 12.7 cm), and the proof bodies prepared like this were subjected to a period of 96 h of immersion in water (DNER, 1994b) for the daily measurement of expansion values. Later, proof bodies were broken in triplicate at a specific press, through penetration of a standardized piston (area = 3 in²), at the speed 1.143 mm/min, obtaining the CSI value.
Statistical analysis
To determine the best results of the UCS trials, the analysis of variance (two-way ANOVA) was used, and the averages with significant effects were compared by the Tukey test at 1% significance, using the GENES software (Cruz, 2013). The factors considered were compaction energy and curing periods for local soil and soil-cement mixture, as follow: (a) Local soil + water + compaction + 0% cement + uncured; (b) Local soil + 2% cement + water + compaction + 1 curing day; (c) Local soil + 2% cement + water + compaction + 7 curing days; and (d) Local soil + 2% cement + water + compaction + 28 curing days. The results of the CSI trials were compared with the recommendations of DNIT (2006) for materials to be used in road paving layers, which are: (a) Sub-roadbed (CSI ≥ 2% and expansion ≤ 2%); (B) Strengthening of the sub-roadbed (CSI ≥ 2% and expansion ≤ 1%); (c) Sub-base (CSI ≥ 20% and expansion ≤ 1%); and (d) Base (CSI ≥ 80% and expansion ≤ 0.5%).
Physical parameters and road soil classification
The granulometric characteristics of the local soil led to the following results in average percentage of its fractions, each with its standard deviation: (a) Clay (21.8 ± 7.15%); (b) Silt (12.7 ± 2.43%); (C) Fine sand (46.6 ± 7.56%); (d) Medium sand (9.9 ± 2.58%); (e) Coarse sand (3.74 ± 1.27%); and (f) Gravel (5.19 ± 2.58%). There was a predominance of the sand fraction in samples (> 60%), and its texture was characterized as sandy-clayey-silty. This soil did not fit in any of the granulometric ranges recommended by the DNIT (2006) for use in granular base pavements, because it does not have 25% of particles larger than 2 mm, referring to the gravel fraction (5.19%).
The average value of soil grains unit mass was 2.69 ± 0.03 g cm-3. Pinto (2000) mentioned that this value is important in the calculation of other physical indices and varies little from soil to soil, with values ​​close to 2.75 g cm-3. There is an association between the typical vs values and the ​​indication of the mineralogical nature of soils.
The average values ​​of liquid limit, LL (25.2 ± 4.13%), plasticity limit, PL (18.6 ± 0.56%) and plasticity index, PI (6.6 ± 3.78%) of the soil corroborate with the typical values ​​of Brazilian soil "variegated loamy sand of São Paulo" (Pinto, 2000). LL and PI values ​​are related to fluctuations in the volume of compacted soils, by contraction or expansion, undesirable characteristics for the construction of roads. The maximum acceptance limits established by the DNIT (2006) for flexible pavements base materials are LL, 25% and IP, 6%.
The local soil was classified as A-4 (1) by the TRB system, indicating a satisfactory overall quality as material to be used in sub-roadbeds, and LA' (sandy laterite) by the MCT system, and the preferred order of use as the basis for local roads, sub-roadbed reinforcement, and compacted sub-roadbed.
Physical characterization and local soil classification in the category LA', according to Nogami and Villibor (2009), indicate that this material presents ease of compaction and adequate cohesion. Bernucci et al. (2006) highlighted the importance of sand in the soil stabilization cost with the use of cement, since a very high percentage of clay in the soil may require considerable increase of the cement content to be used and increased process costs.
Soil compaction and soil-cement mixture
The effect by adding cement in the soil caused slight downward shift of all soil compaction curves (Figure 1). Trindade (2006) noted that the effect of the addition of chemical stabilizers to a soil followed by compaction process can cause changes in the format of its compaction curve, shifting it to the right or to the left, causing changes in its optimal parameters (â±³opt and gdmax). According to Specht et al. (2002) the optimum moisture content (â±³opt) leads to maximum density values ​​in soil-cement mixtures, which reflects in full mechanical strength. The addition of 2% cement produced a less expansive material with higher bearing capacity, improving thus the geotechnical parameters of local soil. It happened because pozzolanic reaction with the surface of particles, increasing adhesive, cohesion and hardness while filling the pores between soil aggregation. The pozzolanic reaction happens even in low cement contents added to the soil as 1 to 4% mixture, increasing durability and water resistance (Baleato and Amorena, 1968; Kézdi and Rétháti, 1988).
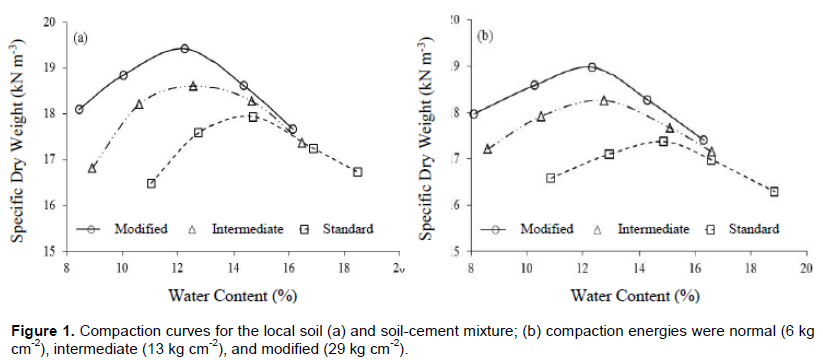
The addition of cement to the soil changed ​​ⱳopt to lower values than natural soil when the same compaction energy was used. Also, the increase in compaction energy led to the reduction of ⱳopt for both the soil and the soil-cement mixture. The maximum soil dry weight (gdmax) reached on compaction curves was reduced by the addition of cement over 3.1, 1.9 and 2.3% for normal, intermediate, and modified energies, respectively. The increase in compaction energy provided greater gdmax values ​​for both the soil and the soil-cement mixture.
Pereira (2012) found no significant differences in â±³opt and gdmax values when adding 3% of cement to a soil classified as A-4 typical from the Parnamirim-RN, located in northwestern of Brazil. This soil had the following granulometric distribution: (a) Clay, 22%; (b) Silt, 39%; (c) Sand, 36%; and (d) Gravel, 3%.
Soil mechanical resistance and soil-cement mixture
By observing the stress-strain curves of USC shown in Figure 2, the increase in compaction energy has improved the compressive strength of both natural soil and mixture. The compaction energy also resulted in sharper apex of curves where the proofs broke, especially for soil-cement mixture. This behavior indicated stronger particle aggregation structure of the mixture compared to the natural soil, which provided stronger cohesion among aggregated particles by chemical reaction. When the highest point of supported strength was reached, soil-cement mixture proofs ruptured more abrupt than natural soil. In other words, natural soil deformation was higher (4%) than the mixture (3%) at the time of breaking point or deflection in relation to its height.
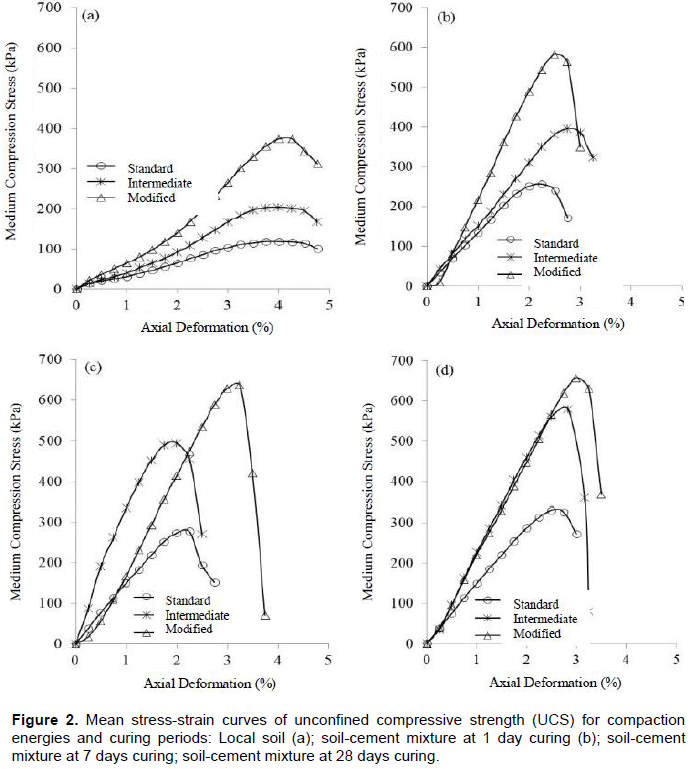
The chemical reaction acting between cement and soil can also be represented in the overall longitudinal rupture and sharp drop of UCS curves, which meant higher degree of soil particles aggregation (Figure 2). Compacting over modified energy, this soil-cement mixture in energy would resist about 675 kPa after 28 days of curing without traffic, deforming 3% in thickness; higher energies than modified can cause disruption and degradation of the pavement. The local soil in its best response, that is, compaction in modified energy, would withstand 385 kPa with 4% deformation.
The mean values ​​of highest UCS have been increased ​​as compaction energies grows for natural soil and soil-cement mixture. Compaction energies did not affect the deformation at highest stress point in local soil. For the mixture, deformation has been changed with compaction energies without comprehensive relationship between stress and strain (Figure2).Therefore, increase in compaction energy produced a positive effect in terms of UCS gains in local soil, which meant higher strength for supporting the traffic of forest transportation. It can be improved by adding 2% of cement and leaving 28 days curing before starting the traffic. Using soil-cement mixture, higher values of UCS can be found compacting on intermediate energy, which produced statistical similar result to modified energy (p<0.05) (Table 1).
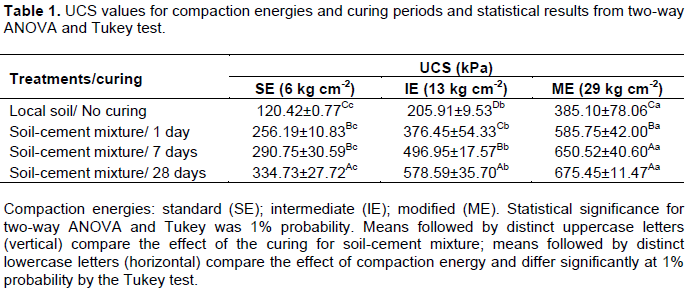
The coefficient of variation (CV) of the variance analysis was low (Table 1), considering the classification proposed by Gomes (2000), which demonstrated high experimental control for compaction proceedings. It was observed that all energy levels were significantly different when compared to the mixtures (p<0.01). The best UCS responses for the standard and intermediate compaction energies were found after 28 days of curing. For modified compaction energy, UCS at 7 and 28 days were statistically equal, at 1% probability by the Tukey test, thus demonstrating that in the 7-day curing period, the soil-cement mixture has reached maximum value of mechanical resistance.
The unconfined compression strength test (UCS) has been used routinely in many experimental programs described in literature, when the objective is to verify the effect of cement in soil stabilization, because it is a simple test, of rapid execution, low cost and it is reliable (Consoli et al., 2007). Jaritngam et al. (2012) mentioned that the cement mixture to a laterite soil is able to produce a material with higher mechanical strength when compared with a soil in its natural state. Bagui (2012) mentioned that the consumption of aggregate material is lower for the case of a base stabilized with cement compared to the conventional method. This feature is extremely important because of the granular materials shortage. Jaritngam et al. (2014) also reported that adding 3% of cement to a laterite soil resulted in a soil-cement mixture with mechanical resistance as high as that of granular materials, demonstrating that the laterite soils reinforced with cement are a viable replacement for aggregate in road pavement construction.
Classification of soil-cement mixture as road materials
CBR trials and expansion of soil-cement mixture were performed for the best results of UCS trials, that is, compaction in intermediate and modified energies, and 28 curing days (Table 2).
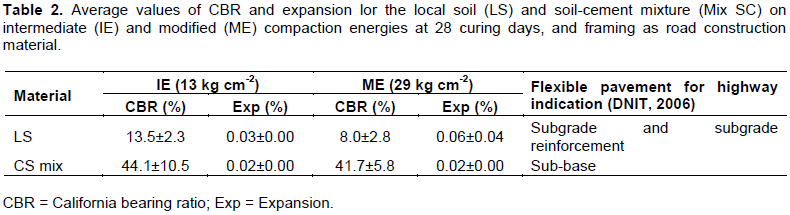
The addition of cement to local soil followed by compaction brought increased bearing capacity to the soil, a fact evidenced by the increase of the CSI value of 226% in intermediate energy and 422% in modified energy. By increasing energy compaction on soil-cement mixture, CBR has not been increased and it probably can be explained by the breakdown of soil particles when compacted with higher energy, causing slight deformation and a rearrangement of particles which resulted in a lower CSI value. The CBR behavior seems to be related to particle-sized distribution and mineral composition of laterite soil rather than cement reaction itself. More studies on the physical and chemical properties of this soil must be performed. For the expansion, cement reduced the values ​​relative to the local soil in numerical terms. However, the compaction energy did not affect the expansion values ​​in the soil-cement mixture. As for the classification of the soil-cement mixture as a material to be used in conventional road paving, according to the DNIT (2006), the local soil can be used in sub-roadbed, while the soil-cement mixture has its use in the sub-base. Therefore, soil-cement mixtures with low stabilizer content, acting as modifier of soil properties, might represent an efficient and economical solution for flexible pavements projects (Sariosseiri and Muhunthan, 2009; Solanski et al., 2009).
The local soil had its engineering properties modified by treatment with cement as stabilizer, producing a material with geotechnical characteristics desirable for forest road construction. The soil-cement mixture showed higher mechanical strength and lower expansion when compared to natural-state, a lateritic soil. This improvement enables its use as construction material of base course in forest roads.
The authors have not declared any conflict of interests.
REFERENCES
ABNT - Associação Brasileira de Normas Técnicas (1984a). NBR 6457: amostras de solos - preparação para ensaios de caracterização e compactação. Rio de Janeiro, Abnt. P. 9.
|
|
ABNT - Associação Brasileira de Normas Técnicas (1984b). NBR 6508: grãos de solos que passam na peneira 4,8 mm - determinação da massa específica. Rio de Janeiro, Abnt. P. 8.
|
|
|
ABNT - Associação Brasileira de Normas Técnicas (1984c). NBR 7181: Solo - análise granulométrica. Rio de Janeiro, Abnt. 13p.
|
|
|
ABNT - Associação Brasileira de Normas Técnicas (1984d). NBR 6459: solo - determinação do limite de liquidez. Rio de Janeiro, Abnt. 6p.
|
|
|
ABNT – Associação Brasileira de Normas Técnicas (1984e). NBR 7180: solo - determinação do limite de plasticidade. Rio de Janeiro, Abnt. 3p.
|
|
|
ABNT - Associação Brasileira de Normas Técnicas (1986). NBR 7182: solo - ensaio de compactação. Rio de Janeiro, Abnt. 10p.
|
|
|
ABNT – Associação Brasileira de Normas Técnicas (1995). NBR 6502: rochas e solos. Rio de Janeiro, Abnt. 18p.
|
|
|
Bagui SK (2012) Pavement design for rural low volume roads using cement and lime treatment base. Jordan J. Civ. Eng. 6:293-303.
|
|
|
Baleato MT, Amorena LS (1968) Estabilizacion de suelos: suelo/cemento. Barcelona, Espanha: Editores Técnicos Associados, S.A. P. 152.
|
|
|
Baptista CFN (1978) Pavimentação: Tomo I: ensaios fundamentais para pavimentação, dimensionamento dos pavimentos flexíveis. 3ª ed. Porto Alegre, Globo. 253p.
|
|
|
Bernucci LB, Motta LMG & Ceratti JAP (2006) Pavimentação asfáltica: Formação básica para engenheiros. 1ª ed. Rio de Janeiro, Petrobras-Abeda. 504p.
|
|
|
Consoli N, Foppa D, Festugato L & Heineck KS (2007) Key paramaters for strength control of artificially cemented soils. J. Geotech. Geoenviron. 133:197-205.
Crossref
|
|
|
Cruz CD (2013). GENES - A software package for analysis in experimental statistics and quantitative genetics. Acta Sci., Agron. 35:271-276.
Crossref
|
|
|
DNER - Departamento Nacional de Estradas de Rodagem (1994a) -DNER. DNER-ME 041/94: Solos: preparação de amostras para ensaios de caracterização. Rio de Janeiro, P. 4.
|
|
|
DNER - Departamento Nacional de Estradas de Rodagem (1994b) ME 129: Solos - compactação utilizando amostras não-trabalhadas. Rio de Janeiro, Dner. 7p.
|
|
|
DNIT - Departamento Nacional de Infraestrutura de Transportes (2006). Manual de Pavimentação. 3ª ed. Rio de Janeiro, Dnit. 274p.
|
|
|
Dias Junior MS, Leite FP, Lasmar Junior E. & Araújo Junior CF (2005). Efeito do tráfego nas pressões de preconsolidação do solo devido as operações de colheita do eucalyptus. Sci. Agric. (Piracicaba, Brazil). 62:248-255.
|
|
|
EMBRAPA – Brazilian Agricultural Research Corporation (2009). CNDPDS. Sistema Brasileiro de Classificação de Solos. 2ª. ed. Rio de Janeiro: EMBRAPA-SPI. P. 412.
|
|
|
Gomes FP (2000). Curso de estatística experimental. 14ª ed. Piracicaba, Degaspari. 477p.
|
|
|
Ingles OG (1968) Advances in soil stabilization. Rev. Pure Appl. Chem, Aust. 18:201-309.
|
|
|
Ingles OG, Metcalf JB (1972). Soil stabilization: Principles and practice. 1ª ed. Sydney, Butterworths. 374p.
|
|
|
Jaritngam S, Somchainuek O, Taneerananon T (2012). An investigation of lateritic Soil cement for sustainable pavements. Indian J Sci Technol. 5:3603-3606.
|
|
|
Jaritngam S, Somchainuek O, Taneerananon T (2014). Feasibility of laterite-ciment mixture as pavement base course aggregate. IJST-T CIV ENG. 38:275-284.
|
|
|
Kézdi Á, Rétháti L (1988). Handbook of soil mechanics: Soil mechanics of earthworks, foundations nd highway engineering. 3ª ed. Amsterdam, Elsevier. 361p.
|
|
|
Lima DC (1981). Algumas considerações relativas a aspectos da estabilização dos solos, em particular à estabilização solo-cal. Dissertação de Mestrado. Escola de Engenharia de São Carlos, São Carlos. 171f.
|
|
|
Machado CC, Malinovski JR (1986). Rede viária florestal. Curitiba, Fundação de Pesquisas Florestais do Paraná. 157p.
|
|
|
Nogami JS, Villibor DF (2009). Pavimentos econômicos: Tecnologia do uso dos solos finos lateríticos. São Paulo, Arte & Ciência. 782p.
|
|
|
Nogami JS & Villibor DF (1995). Pavimentação de baixo custo com solos lateríticos. São Paulo, Vilibor. 213p.
|
|
|
Pereira KLA (2012). Estabilização de um solo com cimento e cinza de lodo para uso em pavimentos. Dissertação de Mestrado. Universidade Federal do Rio Grande do Norte, Natal. 125f.
|
|
|
Pinto CS (2000). Curso básico de mecânica dos solos em 16 aulas. São Paulo, oficinas de textos. 247p.
|
|
|
Portelinha FHM, Lima DC, Fontes MPF & Carvalho CAA (2012). Modification of a laterictic soil with lime and cement: An economical alternative for flexive pavement layers. Soils and Rocks 35:51-63.
|
|
|
Sessions J (2007) Forest road operations in the tropics. . 1ª ed. Oregon, Springer. 170p.
Crossref
|
|
|
Sessions J, Heinrich R (1993). Forest roads in the tropics. In: Pancel L (Ed.) Tropical Forestry Handbook. Berlin, Springer-Verlag. pp.1269-1324.
Crossref
|
|
|
Sariosseiri F & Muhunthan B (2009) Effect of cement treatment on geotchnical properties of some Washington State soils. Eng. Geol. 104:119-125.
Crossref
|
|
|
Solanski P, Hhoury N, Zaman MM (2009). Engineering properties and moisture susceptibility of silty clay with lime, class C fly ash, and cement kiln dust. J. Mater. Civ. Eng. 21:749-757.
Crossref
|
|
|
Specht LP, Heineck KS, Ceratti JAP, Consoli NC (2002) Comportamento de misturas solo-cimento-fibra submetidas a carregamentos estáticos e dinâmicos. ABMS/ABGE. 25:15-34.
|
|
|
Trindade TP (2006) Caracterização tecnológica de três solos residuais estabilizados quimicamente com vistas às aplicacões em geotecnia rodoviária e ambiental. Tese de Doutorado. Universidade Federal de Viçosa, Viçosa. 245f.
|
|
|
Trindade TP, Carvalho CAB, Lima DC, Barbosa PSA, Silva CHC & Machado CC (2008). 1a ed. Compactação de solos: Fundamentos teóricos e práticos. Viçosa, Editora UFV. 95p.
|
|