There is increasing global concern with environmental food production and sustainability to maintain high carbon stocks in soil biomass. The biomass produced in crop-livestock integration system increases soil organic matter, acts in nutrient cycling, improves the physical, chemical and biological characteristics of soil and increases grain production. Moreover, this soil management system mitigates greenhouse effect and preserves the environment. However, in the Savannah of Central Brazil region, an efficient mulching production of biomass is one of the factors limit sustainability of direct seeding of soybean, mainly due to accelerated decomposition of crop residues. Thus, this study aimed to evaluate biomass dynamics of pearl millet and Paiaguas palisadegrass in different forage systems and sowing periods on soybean yield. The experiment followed a randomized block design with a 5 x 2 factorial arrangement and three replications, under five forage systems (monocropped pearl millet, monocropped Paiaguas palisadegrass, pearl millet intercropped in rows with Paiaguas palisadegrass, pearl millet intercropped between rows of Paiaguas palisadegrass and pearl millet oversown and intercropped with Paiaguas palisadegrass) at two sowing periods (February and March). The results showed that Paiaguas palisadegrass in a monocropped system generated the highest biomass production and lowest carbon/nitrogen ratio. The highest carbon/nitrogen ratio and cumulative biomass loss occurred with millet in monocropped and intercropped with oversown Paiaguas palisadegrass in which biomass production was reduced by plant competition. Paiaguas forage systems in palisadegrass monocropped and intercropping on and between rows supported higher yield of soybean. The second sowing periods resulted in higher production of remaining biomass and grain yield, in all forage systems. Intercropping in crop-livestock integration systems showed a promising cultivation technique to maintain a sustainable stock of soil carbon.
There is a growing global concern about climate changes on the planet, result mainly from rising levels of carbon dioxide (CO2) and other greenhouse gases, such as methane (CH4) and nitrous oxide (N2O). However, some systems of land management in different biomes of Brazil, such as the no-tillage system (NTS), the crop-livestock integration system (CLIS) under no-tillage, the adoption of reforestation and management of rangelands, may significantly alter the carbon inventory and other greenhouse gas emission from the soil to the atmosphere and therefore be important in relation to the mitigation of global warming (Carvalho et al., 2010).
In this sense, the consortium in integrated crop-livestock system is a soil management system mitigating greenhouse gas emissions and preserving environmental sustainability due to an increased efficiency in storing carbon in the soil (sequestration of atmospheric carbon). When carried out correctly it can be efficient in soil carbon storage and modulating soil Carbon to Nitrogen ratio, by operating through the root plant system. It also increasesbiomass production to better cover the ground for establishingtillage system to increase sequential crop productivity (Chioderoli et al., 2010) without interfering with the productivity of the intercropped annual crop (Ribeiro et al., 2015; Costa et al., 2016).
So, the production of biomass in no-tillage systems increases diversification when using intercropping, which minimizes the risk of crop losses and provides options for the adoption of crop succession and rotation (Horvathy Neto et al., 2012). In addition, biomass improves the physical, chemical, and biological conditions of the soil, aiding in weed control and in the stabilization of production as well as in the recovery and maintenance of soil quality. One of the ways to achieve these results is intercropping by means of an integrated crop-livestock system (Boer et al., 2008).
However, the correct choice of plant species for the production of biomass on the soil surface is extremely important for the success of a no-tillage system because the climatic factors characteristic of each region and soil type must be considered (Costa et al., 2015). In the region, the climate is characterized by a dry winter, high temperatures throughout the year, and a long dry season. These climatic conditions hinder the establishment of cover crops, mainly by hindering the production of biomass in the crop area, and constitute some of the greatest obstacles to maintaining a no-tillage system (Pacheco et al., 2011).
Therefore, the provision of an efficient biomass soil cover is one of the factors that limit the sustainability of no-tillage systems in the Brazilian savannah region, mainly due to the fast decomposition of residues. This situation reinforces the need for producing plant residues with a slower decomposition rate to maintain the residue on the ground for a longer duration, especially in the off-season (Costa et al., 2015).
Several crops have been tested and utilized for the purpose of producing vegetation cover to ground and for adoption of no-tillage system. Among the most promising highlight millet crop, for presenting resistance to water stress, good biomass production and high carbon/nitrogen ratio, which provides greater persistence of straw on the soil surface (Soratto et al., 2012). However, the grasses of the genus Urochloa, are also widely used, with promising results, and may or may not be under intercropping (Costa et al., 2016). Forage plants are efficient in organic matter supply, determine the improvement in physical structure and soil chemistry, favors the conservation of soil moisture and increases the biodiversity, a fact clearly observed in areas of crop-livestock integration (Krutzmann et al., 2013).
Although known the benefits of intercropping systems and tillage system, more appropriate is scarce information about the vegetation cover, especially the millet intercropped with Urochloa spp. in off-season conditions and with regard to the recommendations of construction and operation of grain production. Thus, this study aimed to evaluate the dynamics of biomass of millet [Pennisetum glaucum (L.) R. Br.] and Paiaguas palisadegrass (Urochloa brizantha cv. BRS Paiaguas) in different forage systems and sowing periods in yield of soybean in crop-livestock integration.
The experiment was conducted in the field (17°48’ S; 50°55’ W; and 748 m altitude), in the municipality of Rio Verde, Goiás, Brazil, during the 2014 off-season in a Latossolo Vermelho Distroférrico (Embrapa, 2013), an Oxisol. Soil samples were collected before planting to determine the physical and chemical characteristics of the 0-20 cm soil layer. The results were as follows: 600, 140 and 260 g kg-1 of clay, silt and sand, respectively; pH in CaCl2: 6.02; Ca: 3.50 cmolc dm-3; Mg: 1.43 cmolc dm-3; Al: 0.05 cmolc dm-3; Al+H: 5.90 cmolc dm-3; K: 0.35 cmolc dm-3; CEC: 11.18 cmolc dm-3; V: 47.22%; P (Mehlich): 2.29 mg dm-3; Cu: 3.50 mg dm-3; Zn: 5.10 mg dm-3; Fe: 34.1 mg dm-3; O.M.: 37.06 g kg-1.
The experiment followed a randomized block design with a 5 x 2 factorial arrangement and three replications, comprising five forage systems (monocropped pearl millet, monocropped Paiaguas palisadegrass, pearl millet intercropped in rows with Paiaguas palisadegrass, pearl millet intercropped between rows of Paiaguas palisadegrass and pearl millet oversown and intercropped with Paiaguas palisadegrass) and two sowing periods (February and March). The ADR 8010 pearl millet hybrid, which is medium-sized and dual purpose (grain production and forage) was used.
Before implementing the experiment, the area was cultivated with saccharine sorghum and Urochloa brizantha cv. Marandu, which, after desiccation, led to an increase in organic matter (OM) in the soil. The area was prepared by desiccating the weeds using 3.5 L ha-1 glyphosate (480 g L-1 acid equivalent), with a spray volume of 150 L ha-1. At 30 days after desiccation, harrowing was conducted with a disc harrow to eliminate weeds not controlled by the herbicide, followed by subsoiling and level harrowing. Soil acidity was corrected with calcitic lime with 100% TRNP (total relative neutralizing power), with the application of 675 kg ha-1 at 30 days before sowing.
One week before implementing the experiment, level harrowing was conducted again, and the field was sown in furrows using a seeder with 0.50 m between rows spacing. The furrows for sowing Paiaguas palisadegrass in between rows and for oversowing pearl millet were manually dug to a 3 cm depth using hoes. Sowing was carried out on February 12 and March 4 for the first and second periods, respectively, using 240 kg ha-1 of P2O5 (single superphosphate form) and 20 kg ha-1 of FTE BR 12 fertilizer.
Monocropped and intercropped pearl millet was sown at a 3 cm depth. Paiaguas palisadegrass was sown in the rows at a 6 cm depth and in between rows at 0.25 m from the pearl millet rows, and in the oversown system, it was sown 15 days after sowing pearl millet in the between rows at 0.25 m. For pearl millet, 12 kg of seeds ha-1 were used, seeking to obtain a final population between 250 and 300 thousand plants ha-1, and 5 kg of pure viable seeds per hectare were used for the forage species. The plots comprised eight 3.0 m long rows in all forage systems. The usable area was obtained by only considering the four central rows and eliminating 0.5 m from the end of each row. At 30 and 50 days after sowing (DAS), 60 kg ha-1 nitrogen and 40 kg ha-1 K2O (in urea and potassium chloride forms, respectively) were applied by casting.
Manual weeding was conducted weekly until 50 DAS for post-emergence weed control. The fall armyworm (Spodoptera frugiperda) was controlled using two applications of chlorpyrifos (1 L ha-1) and teflubenzuron (50 ml ha-1), performed at 40 and 50 DAS, and two applications (37 and 44 DAS) of azoxystrobin + cyproconazole (0.5 L ha-¹). Pearl millet grains were manually harvested at 115 and 118 DAS for the first and second sowing periods, respectively, when the plants were at the physiological maturity stage. The remainder of the plants (stems and leaves) were left at the site for the evaluation of biomass.
After the pearl millet was harvested, the dry weight production of the Paiaguas palisadegrass was evaluated in the off-season crop (simulating grazing) over successive cuttings, in which 1 m2 samples were collected by randomly placing a quadrat within each plot and cutting at a 0.20 m height from the ground to maintain an adequate residue height to promote grass regrowth. The first cutting was performed at the time of the pearl millet harvest, on 06/04/2014 and 06/24/2014 for the first and second periods, respectively. The second cutting occurred 79 days after the first, on 08/22/2014 (first period), and 72 days after the first, on 09/04/14 (second period). After both cuttings were performed, standard cutting of all plants at the experimental site was carried out, at the same height as for the evaluated plants, and the resulting residue was removed from the site. Next, the Paiaguas palisadegrass was left to rest for regrowth, to allow it desiccate it to form biomass for soybean planting during the next crop season.
Desiccation was conducted on 10/31/2014 through application of the herbicide glyphosate at a dose of 4.5 L ha-1 (588 g/L) and spray volume of 150 L ha-1. To quantify biomass production, biomass samples were collected one day before planting of the soybean crop by randomly placing a 1m2 quadrat within each plot. The plant material inside the quadrat was cut, using a height of 0.05 m from the soil surface as a reference. The cut material was weighed, and the samples were dried to constant weight in a forced-air oven at 55°C, and the amounts were extrapolated to kg ha-1.
Intacta RR 2 PRO soybean cultivar M 7110 plants were sown under a no-tillage system above the biomass of the forage systems. At sowing, the soybeans were inoculated with strains of Bradyrhizobium japonicum (SEMIA 5079 – CPAC 15 and SEMIA 5080 – CPAC 7), with a minimum guarantee of 7.2 x 109 CFU/g (Biomax Premium Turfa commercial brand) in the following proportion: 60 g / 50 kg of seeds. Sowing was carried out on 11/20/2014 using a seeder, with the application of 120 kg ha-1 P2O5, 30 kg ha-1 K2O, 2 kg ha-1 boron, 0.4 kg ha-1 molybdenum and 6 kg ha-1 zinc, in the form of single superphosphate, potassium chloride, boric acid, molybdenum sulfate and zinc sulfate, respectively.
Weeds were controlled using the herbicide Transorb (3.5 L ha-1), with a spray volume of 150 L ha-1, on 12/17/2014. One application of the insecticide chlorpyrifos (L ha-1) was performed to control the soybean caterpillar (Anticarsia gemmatalis), and one preventive application of the fungicide azoxystrobin + cyproconazole (0.3 L ha-¹) was performed on 01/13/2015. To quantify biomass production, straw samples were collected by randomly placing a 1m2 - square within each plot. The plant material was cut, using a height of 0.05 m from the soil surface as a reference. The cut material was weighed, and the samples were dried to constant weight in a forced-air oven at 55°C, and the amounts were extrapolated to kg ha-1.
After this management procedure (cutting), the fresh biomass from each plot was placed in nylon litter bags for decomposition (Thomas and Asakawa, 1993). The bags consisted of a 2 mm mesh and measured 15 x 20 cm. Four bags containing residues of the studied species in an amount proportional to the dry biomass produced per hectare were deposited in direct contact with the soil. At 30, 60, 90 and 120 days after cutting, one litter bag was removed from each plot to evaluate the remaining biomass and determine the decomposition time during the 120 day period (soybean harvest).
After cleaning the material in the laboratory to remove adhered soil, it was dried in an oven at 55°C for 72 h to obtain the dry biomass. Next, samples of the plant material were ground to determine the concentration of the following macronutrients according to the method proposed by Malavolta et al. (1997): nitrogen (N) and carbon (C).
Assessments of agronomic characteristics of soybean were held on 03.17.2015 to 117 DAS. They were evaluated in the final stand of soybean plants (plant number count sequenced in 3 samples of 1m linear, the usable area of the plot); plant height and height of insertion of the first and final pod (measures of distance between the ground surface and the apical end and between the soil surface to the insertion of the first and last pod on the main stem, respectively); number of pods per plant (counting all pods with grain in 10 plants in the useful area of the plot, calculating the average number of pods per plant).
To estimate the weight of 100 seeds and grain yield in kg ha-1 were collected in the useful area of each parcel, a sample of all plants contained in three core lines with a meter. These plants, after dried in the sun, were mechanically weighted and threshed by a stationary threshing. The grains obtained were weighed on a precision scale (0.01 g). Then was pulled out a grain sample to determine the moisture carried out with the aid of a digital moisture determiner and later held the mass of the correction of the output of 13% moisture, turning it into kg ha-1. During the experiment, daily rainfall and the mean monthly temperature were monitored.
Data of agronomic characteristics and soybean yield, biomass production and C / N ratio subjected to analysis of variance and the means were compared using Tukey’s test, with a significance level of 5%. Statistical analyses were performed using SISVAR 4.6 statistical software (Ferreira, 2011).
To describe the decomposition of plant residues, the data were fit to an exponential mathematical model, using the SigmaPlot application. For regression equation comparisons, the procedure described in Snedecor and Cochran (1989) was used after data linearization.
To calculate the half-life (t1/2), that is, the time required for 50% of the remaining biomass to be decomposed, the Paul and Clark (1989) equation was used: t1/2=0.693/k, where t1/2 is the half-life of the dry biomass, and k is the decay constant of the dry biomass.
Production and decomposition of biomass
The production of biomass was affected (P<0.05) by the sowing period and forage system. However, there was no effect (P>0.05) of the interaction between forage system and sowing period (Table 1).
For the first and second sowing periods, the highest biomass was obtained in the Paiaguas palisadegrass monocropped, which showed biomass production satisfactory for use in the no-tillage system. After the pearl millet harvest, two cuttings of the Paiaguas palisadegrass plants were performed in the forage systems, and new tillers sprouted at the beginning of the rainy season (September). This resulted in the production of 3.810 and 2.766 kg ha-1 of biomass for the first and second periods, respectively, thus forming the soil cover for soybean planting (Table 1). These results indicate that Paiaguas palisadegrass has favorable features for use in integrated crop-livestock systems, such as forage production during the dry season, with good regrowth. Franchini et al. (2009) reported that the higher total soil cover can bring benefits, such as nutrient recycling and improvements in soil physical and biological conditions.
Similar results were obtained from biomass by Costa et al. (2015), who evaluated the production of straw forage species (pearl millet, sorghum and Xaraes palisadegrass) verified biomass of Xaraes palisadegrass of 3607 and 3867 kg ha-1, for the harvest of 2009/2010 and 2010 / 2011 respectively. The lowest biomass was obtained when the Paiaguas palisadegrass was intercropped with pearl millet under oversowing (Table 1), thus showing that this type of sowing system affects grass development. This result occurred because the Paiaguas palisadegrass was established 15 days after pearl millet sowing, increasing the shading by the pearl millet plants of the Paiaguas palisadegrass during its early germination stages (Costa et al., 2016). Thus, there was a decrease in forage production, directly affecting the production of biomass in the no-tillage system. A similar result was obtained by Gazola et al. (2013), who showed that shading by corn plants affected dry biomass production by Urochloa brizantha cv. Marandu and Urochloa ruziziensis due to the delayed emergence of the Urochloa plants. Seidel et al. (2014) evaluated dry biomass production by MG-4 palisadegrass and observed a decrease of 81.70 and 62.56% when the grass was sown in row and between rows, respectively, 25 days after corn sowing. Notably the Paiaguas palisadegrass among many Urochloa cultivars, can be one of the most suitable in crop-livestock integration systems to settle with less water availability and produce during the off-season (Costa et al., 2016). Thus, it can be considered excellent choice as producing crop biomass, aimed at soybean sowing in no-tillage system in the Southwest region of Goiás, Brazil.
In addition to these favorable features, the results obtained by Machado and Vale (2011) showed that in the three years of evaluation, Paiaguas palisadegrass (B 6 lineage) was not only the most productive option but also the one showing the highest desiccation efficiency, when compared with the genotypes Marandu, MG-4, Xaraes, Piata, and Arapoty. This feature is important because at least 21 days are needed after herbicide is applied to the forage before soybeans can be sown. Regarding to the sowing date (Table 1) in all forage systems the second sowing periods showed higher production of biomass and biomass remaining at 30, 60, 90 and 120 days. This result may be correlated with the uneven distribution of rainfall in February, with frequent dry spells observed at the beginning of emergence, which impaired the initial plant development forage systems (Costa et al., 2016), which was influenced at final production of biomass.
Table 1 shows that for the remaining biomass at 30, 60, 90, and 120 days, the forage systems containing pearl millet in monocropped and intercropped with Paiaguas palisadegrass in row and between rows showed similar results but differed (P<0.05) from those containing oversown and intercropped pearl millet and monocropped Paiaguas palisadegrass. The cespitose growth habit observed in both Paiaguas palisadegrass and pearl millet most likely contributed to a similar decomposition rate in these systems, as it enabled not only a better biomass distribution on the soil but also a similar biomass production, thus resulting in less contact of the biomass with the soil. Given the above, it can be concluded that these sowing methods did not affect the remaining biomass when compared with that of the monocropped pearl millet because the latter showed a lower biomass loss due to its greater amount of lignified material. This again shows the importance of intercropping for biomass production within no-tillage systems in the Cerrado region.
Assessing the consortium corn with Urochloa brizantha cv. Xaraes and Urochloa ruziziensis in the Mato Grosso do Sul State, Costa et al. (2014) found that between 90 and 120 days after handling, decomposition of the remaining biomass showed decreasing effect, and the temperature and rainfall favored the rapid decomposition of the straw on the soil surface in Brazilian savannah region. Similar results were obtained in this study for the two sowing dates.
Regarding the sowing periods, lower remaining biomass amounts were observed for the second sowing period at 30 and 60 days for Paiaguas palisadegrass in monocropped and intercropped in row and between rows as well as at 90 days for the Paiaguas palisadegrass in monocropped and intercropped in between rows. Moreover, at 120 days, only the Paiaguas palisadegrass in monocropped showed a lower amount of remaining biomass (Table 1). This result can be explained by the longer forage rest duration during the first period (79 days) compared with the second period (72 days), thus resulting in better use of the September rains and allowing better regrowth. With the exception of pearl millet in monocropped and oversown as well as intercropped with Paiaguas palisadegrass, the first sowing period provided higher biomass production. This result can be related to the uneven distribution of rainfall from May to August, when low rainfall was observed, hindering plant development when the sowing was conducted in March.
When evaluating the cumulative percentage loss (Table 2) for the first sowing period, all the assessments showed the lowest loss for pearl millet oversown and intercropped with Paiaguas palisadegrass, which differed from the losses in the other forage systems. However, for the second period, the values were similar, with no significant effect (P>0.05) of forage system on the loss. The same result was observed when the sowing periods were compared. The greatest percentage decrease in biomass occurred at 30 days after management due to the initial decomposition of the less lignified leaves and other materials, which were easily decomposed (Wolf and Wagner, 2005). Thus, the presence of Paiaguas palisadegrass in the intercropped systems contributed to a more rapid decomposition of the biomass because of the high leaf production of this grass. Conversely, the smallest percentage loss values were found in the monocropped pearl millet and pearl millet oversown and intercropped with Paiaguas palisadegrass, which mostly contributed greater amounts of lignified material to the biomass due to the predominance of pearl millet.
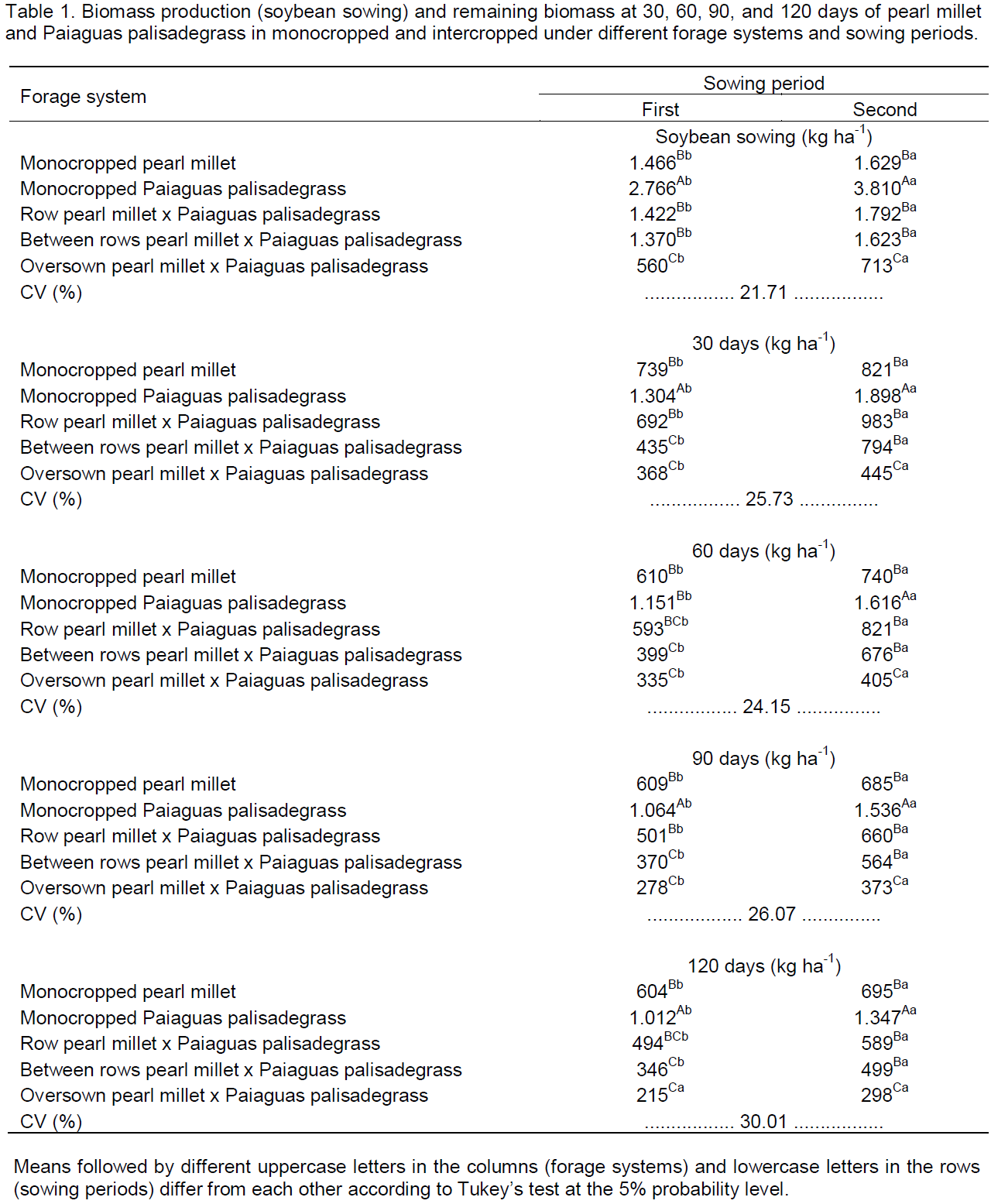
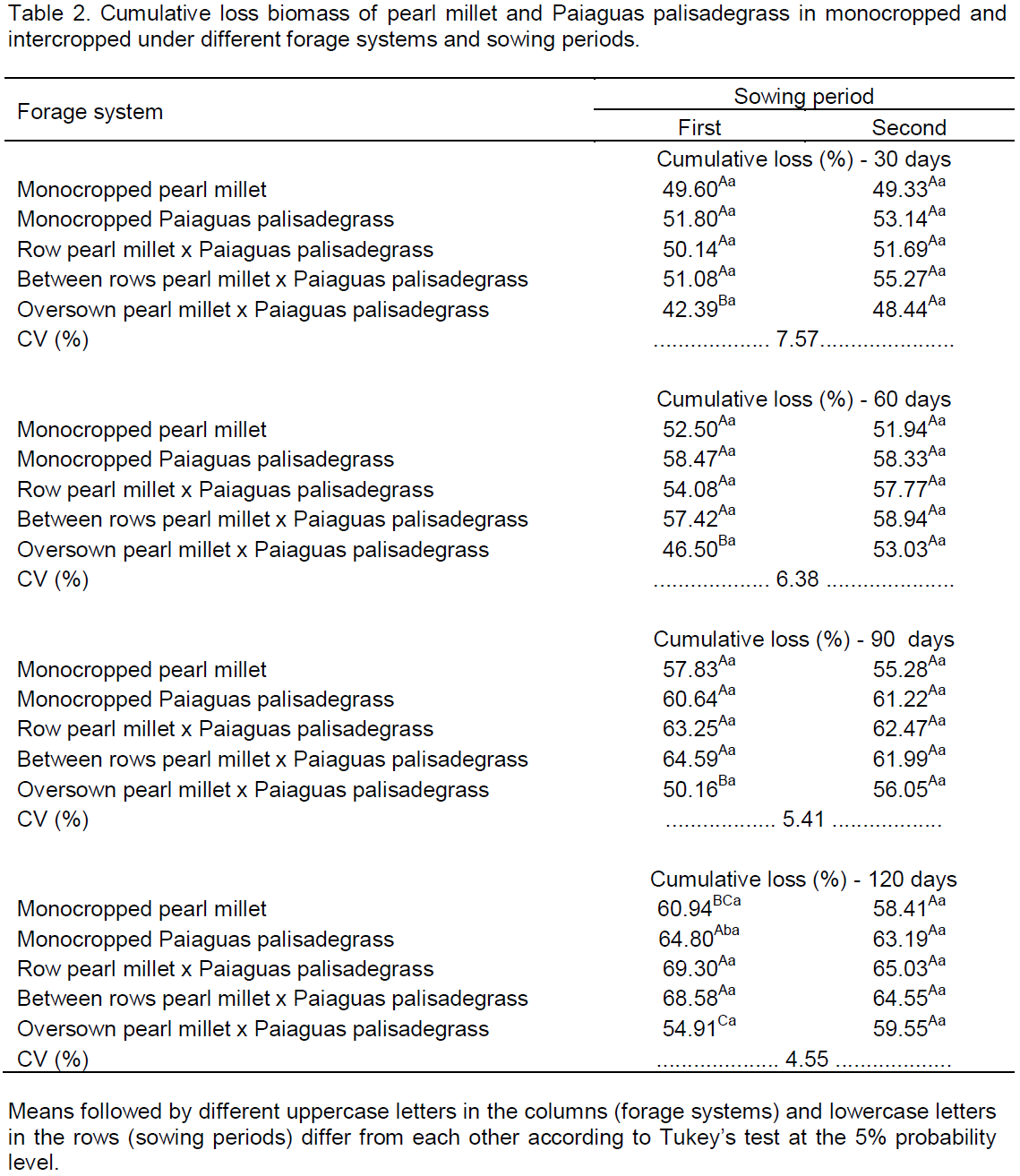
In general, the forage plant biomass durability is evaluated using the carbon/nitrogen ratio of the plant, with higher decomposition below a ratio of 25:1 (Costa et al., 2015). Thus, the higher carbon/nitrogen ratio found in pearl millet is responsible for the lower biomass decrease found in these systems.
Studies evaluating the cumulative biomass loss for the no-tillage system are still scarce for intercropped systems of pearl millet with Urochloa species. However, for the corn crop, some studies have already evaluated intercropping with species of Urochloa, and Costa et al. (2014) found that the cumulative loss percentage of the remaining biomass was 15 to 60% at 120 days. Kliemann et al. (2006) observed a relative loss of 56% by 150 days, and Santos et al. (2014a) found that one half of the dry cumulative biomass had decomposed by 115 days.
Compared with the other forage systems, the monocropped pearl millet showed a higher carbon/ nitrogen ratio (P<0.05) for both sowing periods at soybean planting at 30 and 90 days. At 60 and 120 days, the carbon/nitrogen ratio of the pearl millet was similar (P<0.05) to that in the intercropping with oversown Paiaguas palisadegrass (Table 3) because of the greater competition in this system.
Boer et al. (2008) found a carbon/nitrogen ratio of 34:1 for the ADR500 pearl millet at the full flowering stage, a ratio lower than that found in the present study. The decomposition rate is directly related to the carbon/ nitrogen ratio of the residue on the soil. This relationship indicates the potential of these cover plants for soil cover maintenance due to the greater soil residue persistence, especially in the off-season.
The intercropping of pearl millet with Paiaguas palisadegrass in various forage systems provided lower carbon/nitrogen ratio values because of the higher production by Paiaguas palisadegrass of leaves, which rapidly decompose, influencing the end result of the carbon/nitrogen ratio. Pacheco et al. (2011) found that the lower carbon/nitrogen ratio (34:1) found in Urochloa ruziziensis compared with pearl millet (61:1) at 200 days after sowing was caused by the higher proportion of leaves to stems of the former in response to high regrowth rates after the early summer rains.
A downward trend was observed in the carbon/nitrogen ratio when comparing the soybean sowing assessments through 120 days. This tendency was due to decreases in the biomass C concentrations arising from an increase in the microbial population during the early stages of decomposition because these organisms use the N available in the soil to metabolize C, thus immobilizing it. As the decomposition continues, the increase in soil microbiota leads to a higher C consumption in the biomass and to a decrease in the carbon/nitrogen ratio, with greater mineralization being observed when this ratio is near 20:1.
Note that a lower carbon/nitrogen ratio can shorten the duration of biomass retention on the soil surface, especially under the cerrado climate conditions, charac-terized by high temperatures and humidity (Kliemann et al., 2006). However, a lower carbon/nitrogen ratio at the end of the off-season provides a faster mobilization of nutrients to the soil after its desiccation, thus favoring the annual crops in rotation (Pacheco et al., 2011). Nevertheless, in all the forage systems of the present study, the carbon/nitrogen ratio was above 30:1, a reference value for the carbon/nitrogen ratio considered by Trinsoutrot et al. (2000) to be high enough to characterize residue as suitable for use in a no-tillage system.
Certain studies indicate that intercropping may favor an increase in dry biomass, which is essential for the no-tillage system, providing an increase in the carbon/ nitrogen ratio and contributing to a reduction in the biomass decomposition rate (Kliemann et al., 2006; Seidel et al., 2014). In these studies, higher carbon/ nitrogen ratios were observed for the intercropping of corn with the grasses Urochloa brizantha cv. Marandu and Urochloa brizantha cv. MG-4, and such ratios were conditioned by the higher amount of stem and lignified material in corn compared with pearl millet in the present study, which favored the intercropping system.
Table 4 shows the regression equations for the decomposition of remaining biomass and the coefficients Po and k for the regression equation P=Po−kt and R2 as well as the coefficients of determination (R2) for the biomass decomposition of the different forage systems, which were determined in both sowing periods from 0 to 120 days after management. The half-life was lower in both sowing period treatments for pearl millet intercropped with Paiaguas palisadegrass in row and between rows, when compared with the other forage systems. These results are due to the lower carbon/ nitrogen ratio (Table 3) of these combined materials, caused by a higher leaf production by Paiaguas palisadegrass and consequent increase in the rate of biomass decomposition.
The highest half-life values were observed in the pearl millet oversown and intercropped with Paiaguas palisadegrass (first period) and in monocropped pearl millet (second period) (Table 4). These results are explained by the relatively large amount of lignified material in pearl millet, which thus stays on the ground for a relatively long time, and its short development cycle. Floss (2000) reported that residues decompose more slowly as their lignin contents and carbon/nitrogen ratios increase.
The half-lives of pearl millet were also evaluated by Boer et al. (2008), who found a value of 105 days when the millet was managed at full flowering, and by Assis et al. (2013), who found a half-life of 187 days for ADR 500 pearl millet. The differences among the half-lives observed by these authors and detected in the present study are due, among other factors, to the genotype examined: ADR 500 is a variety used specifically for the production of soil biomass cover, whereas ADR 8010 has a dual purpose, being used for both grain and biomass production. In addition, the pearl millet was desiccated in these previous studies during the flowering stage, when there was a greater stem diameter and consequently a greater amount of lignified material in the biomass, which slowed its decomposition and increased its half-life.
The relatively high biomass loss during the first days for both sowing periods. This high loss can be explained by the large amount of leaves, which are easily decomposed, increasing the microbial activity and the decomposition of the easily degraded soluble components, such as sugars, starches, and proteins that are quickly used by decay-causing organisms (Wolf and Wagner, 2005).
The monocropped Paiaguas palisadegrass showed a higher production of remaining biomass for both sowing periods, as well as faster initial decomposition caused by the high leaf: stem ratio of this forage plant (Machado and Valle, 2011). These results show the importance of the production of biomass with a greater resistance to decomposition for maintaining the soil cover during the off-season.
The decomposition rate of the remaining biomass determines the persistence of the soil cover, which is influenced by the lignin content and carbon/nitrogen ratio of the residue. Grasses generally yield relatively large amounts of biomass that is characterized by a high carbon/nitrogen ratio, which helps increase the persistence of the soil cover (Noce et al., 2008). Kliemann et al. (2006) found that compared with monocropped corn, intercropping corn with Marandu grass contributed to a lower biomass loss at 150 days after sowing. For all the forage systems, the second sowing period provided the highest remaining biomass production.
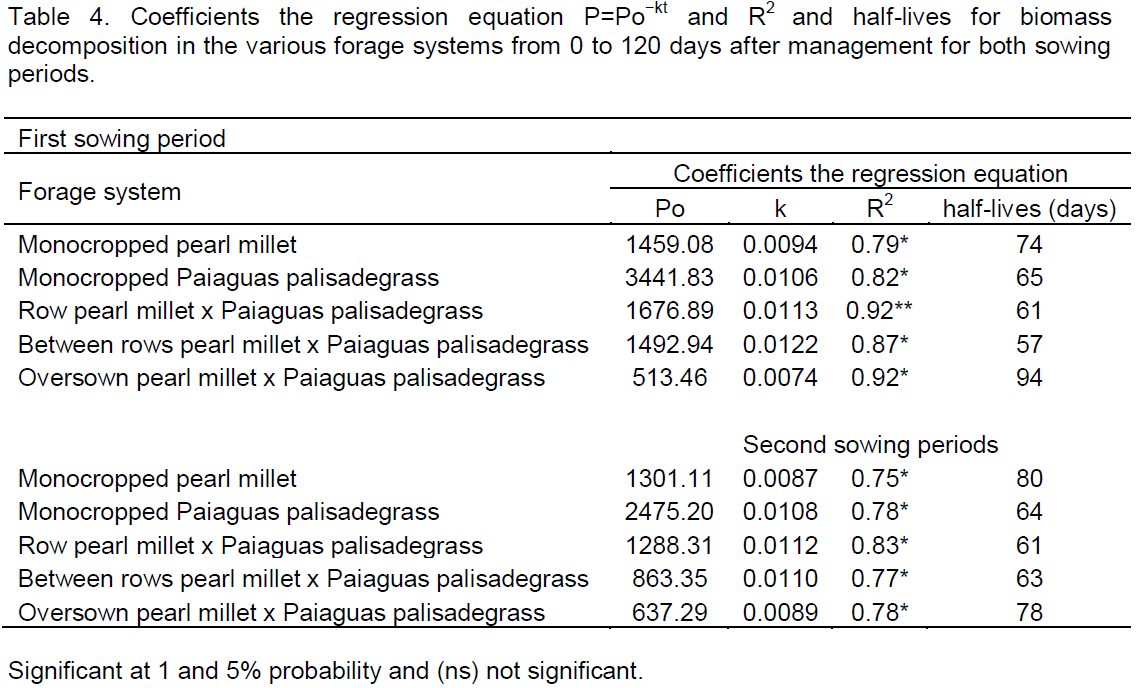
The results obtained with Paiaguas palisadegrass showed that this grass provides a promising option for the production of high-quality biomass in the off-season. In the Brazilian savannah, efficient mulching with biomass is one of the factors needed for the sustainability of no-tillage systems, mainly because of fast residue decomposition. Under these conditions, the use of Paiaguas palisadegrass, which showed a relatively slow decomposition rate, represents a strategy to increase the soil cover efficiency, especially in the planting prior period of soybeans in the summer season.
Agronomic characteristics and yield of soybean
The height of the soybean plants was not influenced (P>0.05) by the interaction of forage systems and sowing period. However, there was significant effect (P<0.05) for forage systems in the first planting date. The greatest heights of plants were obtained in monocropped Paiaguas palisadegrass e intercropped in rows and between rows (Table 5). These results are correlated with higher biomass produced in these systems, associated with a high carbon/nitrogen ratio (Tables 1 and 3), increasing the durability of the dry matter in soil (Table 2), which may have contributed to a slow decomposition and greater accumulation and availability of nutrients for the soybean crop. Among the characteristics observed, stay on soil also brings physical benefits (airier soil) and biological (increased microbial activity for decomposition) that promote the best establishment of soybean plants as recommended (Barbosa et al., 2011).
Notably, the biomass importance of tropical grasses to keep soil moisture, especially in the Central Brazilian savannah conditions (Torres et al., 2006), resulting in sustainability and efficiency, storing carbon in the soil. In this study, the vegetative stage of soybean development in January was dry spell of 20 days. In this sense, the forage systems pearl millet monoculture and intercropped with Paiaguas palisadegrass in oversowing, soybean development was hampered, influencing in a smaller plant height (Table 5) due to lower production of biomass (Table 1) in these systems.
However, when evaluated the second sowing periods, there was no significant effect (P>0.05) in plant height between forage systems (Table 1), averaging the height of 84.43 cm. The same occurred in comparison to sowing period where forage systems did not influence the plant heights. The effect of vegetation on the agronomic characteristics of soybean were also evaluated in some studies as the Santos et al. (2014b) found that soybean plants heights ranging from 85 to 90 cm in different covers of perennial pastures under no-tillage system, being similar to those obtained in this study.
However, Barbosa et al. (2011) found higher height values of 108.27 and 108.75 cm soybean plants, the straws of pearl millet and Marandu grass in exclusive crop, respectively. Borges et al. (2015) evaluated agronomic traits soybean under different vegetable toppings, found lower values when compared to this study, plant height ranging from 47 to 52 cm on the biomass of Pennisetum americanum and 47 to 51 cm on biomass of Urochloa ruziziensis.
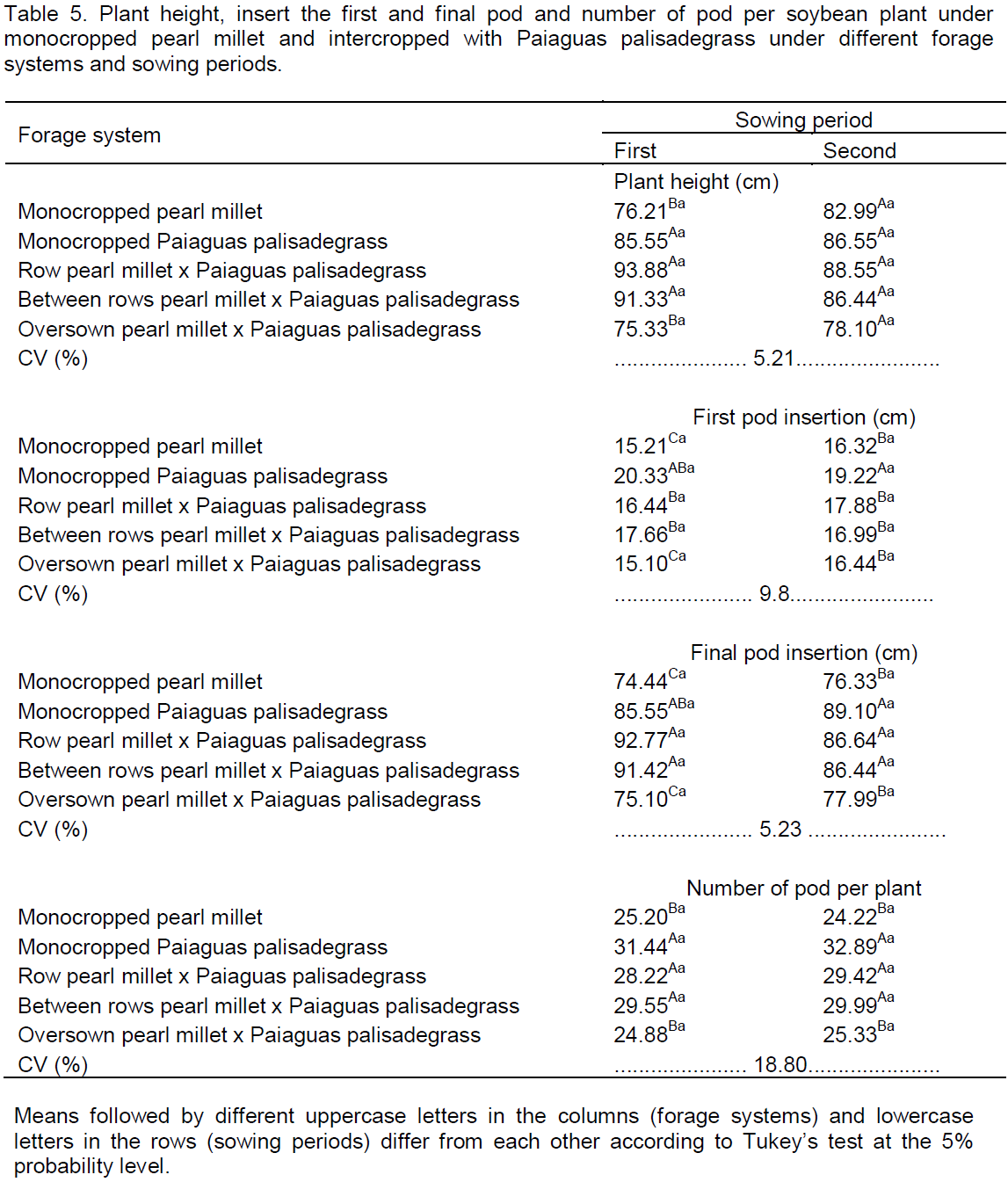
The forage systems affected (P<0.05) at the time of insertion of the first and end soybean pod in both periods (Table 5). In the first date, the greatest heights were obtained studying Paiaguas palisadegrass in mono-cropped and intercropping system on row and between rows, differing pearl millet monocropped and intercropped oversown. Probably the highest yields of biomass accumulated in these systems (Table 1) influenced the date of insertion of the first and final soybean behavior. Thus it is evident the importance of intercropping systems through crop-livestock integration, to increase the height of insertion of string beans, which is directly related to grain yield. In the second sowing, for the first pod height, only the Paiaguas palisadegrass monocropped system differentiated from other systems with greater height. And for the insertion height of the final pod, pearl millet mono-cropped and intercropped with Paiaguas palisadegrass in oversown, showed lower height.
The first pod insertion height is an important agronomic trait, because it is related to the efficiency of mechanical harvesting of grain operations, it is recommended that this variable present at least 13 cm to reduce the losses during harvest (Medina et al., 1997). Thus, considering the mean height of insertion of the values of the first pod observed in this study, regardless of the forage systems, it appears that there is no limitation to the mechanical harvesting of soybean, with the lowest average of this characteristic was observed system pearl millet mono-cropped and intercropped with Paiaguas palisadegrass in oversown the first date, with an average value of 15.15 cm. Results similar to these forage systems were obtained by Barbosa et al. (2011) found that insertion height of the first pod of 15.81 and 15.31 cm on the exclusive biomass pearl millet and Marandu palisade grass respectively. Assessing the sowing periods of the first insertion height and last pod, it appears in Table 5 that the times did not influence (P>0.05) at the time all forage systems, showing similar results. The total number of pods per plant was influenced (P>0.05) by the forage systems, however, there was no significant effect on the sowing dates (Table 5). The highest values were observed in Paiaguas palisadegrass systems in monoculture and intercropping on the row and between rows sowing periods. Again, these results show that these systems favored for best soybean development due to increased biomass production (Table 1) which resulted in a higher moisture and lower soil temperature.
Rather, the pearl millet system in monocropped and intercropped with Paiaguas palisadegrass in oversown resulted in lower soybean development, which can be correlated with the lower ground cover due to the lower production of biomass and to pass a water stress period in the vegetative phase, hindered the development of soybean. It is noteworthy that the number of pods per plant is considered the main component that controls the soybean crop production.
The number of pods observed in this study was higher than that found by Lima et al. (2009) with values of 10.8 pod per plant of pearl millet biomass and lower than that observed by Barbosa et al. (2011) with 68.65 and 66.75 values for pearl millet covers and Marandu palisadegrass, respectively.
It is also possible to verify that there is a positive correlation between the number of pods per plant with the other characteristics discussed previously, where the larger plant height, insertion height of the first and last pods provided in a larger number of pods per plant. Probably a larger rod provided greater area for tillering and string soybeans production. It is noteworthy that the highest values were observed in all of these charac-teristics are also related to higher biomass of Paiaguas palisadegrass systems in monoculture and intercropping on rows and between rows (Table 1), which indicates the efficiency in the adoption of these systems for suitable biomass production for the no till system.
The population density of the soybean plants final stand was influenced (P>0.05) by forage systems (Table 6). It is observed that the intercropped with pearl millet with Paiaguas palisadegrass in rows showed higher final stand with 31.92 plants m-1, and 26.51% more plants than the over seeded system, which presented the lowest final stand for both seasons. However, there was no seeding date effect (P>0.05) in all forage systems for this characteristic.
Probably the biggest biomass production (Table 1), and the lowest decomposition, may have favored the early development of seedlings and also their survival until the end of the cycle. Among the beneficial factors of this accumulation and retention of biomass in the soil are: increased moisture retention, greater control of soil temperature range (Torres et al., 2006), control weeds, and (Nunes et al., 2010) the release of nutrients to the decomposition of biomass for soybeans also providing for greater productivity (Boer et al., 2008).
Lemos et al. (2003) observed that the largest soybean production occurred in conditions of greater production of biomass. Thus the Paiaguas palisadegrass monocropped and intercropping on the rows and between rows, because they showed a higher biomass production (Table 1) may have favored the population density of soybean plants of the final stand. As for the weight of 100 grains, there was no significant effect (P>0.05) for forage systems and sowing period (Table 6). Many studies also found no effect of different vegetation cover on the weight of 100 seeds, as shown in the research of Lima et al. (2009); Santos et al. (2014b) and Borges et al. (2015). The results obtained for grain yield were influenced (P<0.05) by forage systems and sowing period (Table 6). The Paiaguas palisadegrass system in monocropped and intercropped in rows between rows, provided the best results in both evaluation periods. This result shows the importance of Urochloa for biomass as ground cover, for soybean yield in no-tillage system.
Among Urochloa cultivars is worth mentioning that Paiaguas palisadegrass is one of the most indicated in the crop-livestock integration system to establaish with lower water availability and produce well during the period of low rainfall and may be considered an excellent option as producing crops biomass (Machado and Assis, 2010), aiming at the implementation of no-tillage system in the Central West of Brazil (Costa et al., 2016), a fact clearly observed in grain yield data. According to Lopes et al. (2009) the addition of plant residues in the soil in areas under use of livestock farming system integration by direct seeding is very important for maintaining and increasing the levels of organic matter in the soil, which plays a key role in maintaining sustainability of production over time and also in carbon stock, resulting in more sustainable soy production. The soybean yield values obtained in this study were similar to those observed by Borges et al. (2015) who found significant effects on the type of coverage of grain production, where millet coverage provided grain production ranging from 4218 to 4613 kg ha-1 and Urochloa ruziziensis 4396 to 4679 kg ha-1.
Regarding the sowing period, in all forage systems the second season periods had higher grain yield (Table 6). This result may be correlated with increased biomass production in this period (Table 1), which reflected positively on soybean productivity.