ABSTRACT
Many researches have performed on gypsum application to correct high exchangeable aluminum content in acidic soils, especially in subsurface. Although, they have found increases in the exchangeable calcium and sulfur contents, exchangeable aluminum has not been decreased. The exchangeable aluminum buffering by organic matter may contribute to non-significant reduction in exchangeable acidity. The objective of this study was to evaluate the effect of mineral gypsum on the aluminum compartments of Hardsetting Ultisol and monitor the capacity of gypsum to provide exchangeable calcium and sulfur in subsurface. Increasing amounts of mineral gypsum (0, 2, 4, 6, and 8 Mg ha-1) were applied with broadcast at the field. The experimental design consisted of randomized block with four replicates. Aluminum compartments (Al-organic matter, Al-amorphous and Al-crystalline) were evaluated at 0 and 15 days after gypsum application and the exchangeable calcium and sulfur contents were evaluated at 0, 15, 64 and 90 days. The evaluations were performed in three different layers. Gypsum application increased the exchangeable calcium and sulfur contents in subsurface and did not reduce the exchangeable aluminum content. Al-organic matter in the surface layers decreased along the incubation time and application gypsum. Al-amorphous in the subsurface layers increased with the increased amounts of gypsum. Al-crystalline decreased as soil depth increased. The exchangeable aluminum buffering in surface layers was performed by Al-organic matter and, in subsurface, by Al-crystalline.
Key words: Aluminum compartments, aluminum fractionation, soil acidity, soil pH, exchangeable calcium.
The soil exchangeable Al determination is not an appropriate indicator for evaluation of the performance of the gypsum on soil exchangeable acidity. Many researches with gypsum application to correct high exchangeable Al content in acidic soils have been performed in Brazil, without the expected success (Saldanha et al., 2007; Rocha et al., 2008; Santos et al., 2013b). There are reports stating that one of the main actions of gypsum result in the formation of Al(SO4)+ (Santos et al., 2013b). Most of this complex is leached, but may be a considerable fraction is removed by KCl extractor, making exchangeable Al determination after the gypsum important in the evaluation of soil acidity, because Al in application to be overestimated.
The determination of Al-oxyhydroxides, Al-sulfate minerals of low crystallinity and Al-organic matter is very these compartments can buffer the exchangeable Al (Takahashi et al., 2006). Al of these compartments may be converted in exchangeable Al and, depending on the converted amount Al may be toxic to plants.
Gypsum application in the soil can promote modifications in these different Al compartments (Takahashi et al., 2006; Li et al., 2010; Álvarez et al., 2012). This is important because plants have different tolerance to the presence of exchangeable Al in the soil (Reyes-Díaz et al., 2011). Decrease in soil Al contents occurs due to the formation of Al-hydroxides and Al-sulfate minerals of low crystallinity resulting from the gypsum application (Takahashi et al., 2006). These compounds leach to soil deeper layers, distant from the zone of highest absorption by the roots. Childs et al. (1983) claimed that the determination of amorphous Al-oxyhydroxides with solution of ammonium acid oxalate (AAO) satisfactorily estimated the amount of Al-amorphous leached from the soil upper layers.
Thus, techniques that enable the quantification of the Al in different Al-compartments (Al-crystalline, Al-organic matter and Al-amorphous), allow investigating more accurately, the influence of gypsum on Al and its translocation along soil profile.
In this context, this study aimed to evaluate the effect of mineral gypsum application on the Al-compartments of an Hardsetting Ultisol in Pernambuco, Brazil, and monitor the capacity of mineral gypsum to supply exchangeable Ca and S-SO42- in subsurface.
The experiment was installed in the field in January 2012 at Sugarcane Experimental Station of Carpina (EECAC/UFRPE), in Pernambuco, Brazil (Figure 1). The soil was classified as dystro-cohesive Red Yellow Argisol (Santos et al., 2013a), corresponding to Hardsetting Ultisol (Soil Survey Staff, 1998). Soil tillage consisted of harrowing, without limestone application. Five soil samples were collected in the experimental plots, in order to form a composite sample of the layers of 0.0-0.2, 0.2-0.4 and 0.4-0.6 m, for chemical and physical characterization (Donagema et al. 2011) (Table 1).
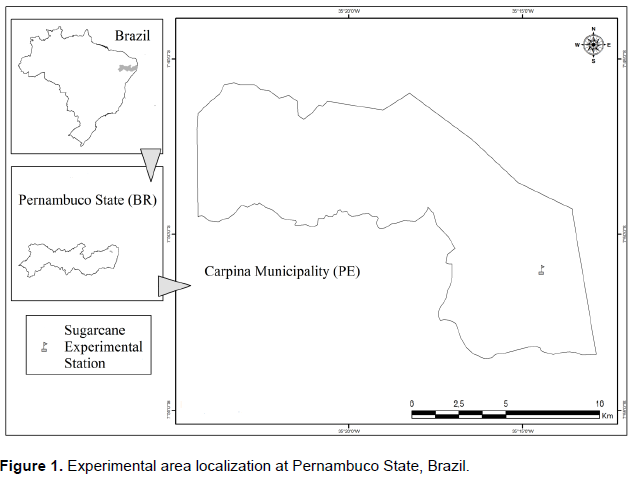
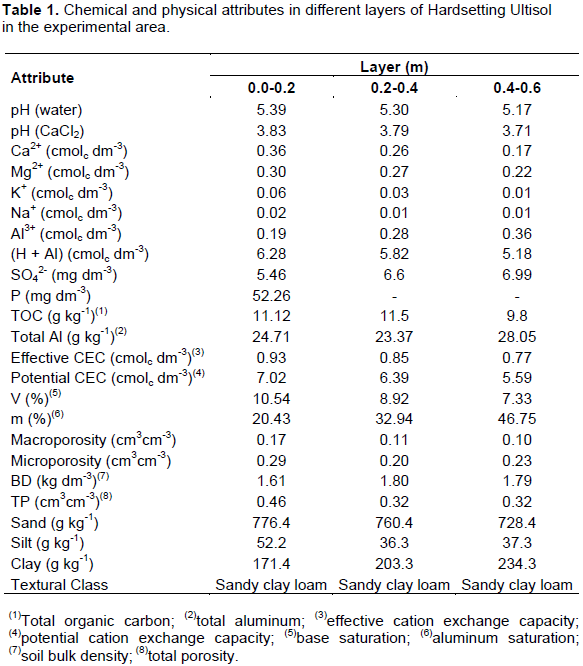
The treatments corresponded to the application of 0, 2, 4, 6 and8 Mg ha-1 of mineral gypsum (18% of Ca and 23% of S-SO42-) and were distributed in a randomized block design with four replicates, totaling 20 experimental plots. The plots had dimensions of 6.0 x 12.0 m, with 72.0 m2 of total area. The evaluated area corresponded to 32 m2 (4.0 x 8.0 m), disregarding 1.0 m on each side along the width of the evaluated area and 1.4 m along the length of the plot (Figure 2). Gypsum application was performed by broadcast on soil surface, without incorporation. After treatments applying, the experimental area was subjected to irrigation for 90 days, in order to maintain the soil at field capacity and solubilize the gypsum.
Irrigation depths of 25 mm were weekly applied and, combined with the rainfalls, totaling 322.7 mm. This amount of water was calculated to solubilize the highest dose of mineral gypsum (8 Mg ha-1), considering its solubility as approximately 2.5 g L-1 (Ramos et al., 2006).
Effect of gypsum on the exchangeable Ca, exchangeable Al and S-SO42- contents in the soil was evaluated at 0, 15, 64 and 90 days after its application. Five individual samples were collected in the tips and in the center of two diagonals drawn in the evaluated area of the experiment, in the layers of 0.0-0.2, 0.2-0.4 and 0.4-0.6 m. Soil pH in water and CaCl2 also was evaluated.
Soil pH was determined in a proportion, 1:2.5 (solo : water). In 10 mL of soil were added 25 mL of water or 25 mL of CaCl2 0.01 mol L-1. The sample was shaken and then rested for one hour. Soil pH was measured in potentiometer (Digimed® DM-22) (Donagema et al., 2011).
Exchangeable Ca and Al were extracted with KCl 1 mol L-1 from 10 mL soil sample after shaking for 15 min and overnight decantation. Exchangeable Al was determined through titration of a aliquot of 25 mL of the supernatant with NaOH 0.025 mol L-1 and 3 drops of bromothymol blue as indicator, and exchangeable Ca was dosed through atomic absorption spectrophotometry (PerkinElmer® AAnalyst 200) using half of the total volume with SrCl2 to control readings interferences (Donagema et al., 2011).
Soil S-SO42- was extracted from 10 mL of soil sample using 25 mL of Ca(H2PO4)2 solution containing 500 mg L-1 of P and CaCl2 0.01 mol L-1. After extraction, S-SO42- was dosed by turbidimetry (Biospectro® SP-22) based on the optical density of the solution (420 nm) after addition of 500 mg of BaCl2.2H2O (Alvarez et al., 2001). From 0 to 15 days after gypsum application, Al-compartments were evaluated in the treatments under the application of 0, 4 and 8 Mg ha-1 of gypsum. These Al-compartments were determined using the selective dissolution of Al.
Al-crystalline was extracted with dithionite-citrate-bicarbonate solution (Mehra and Jackson, 1960). Al-amorphous compounds were extracted with solution of ammonium acid oxalate (Schwertmann, 1964). Al-organic matter was extracted with pyrophosphate solution (McKeague, 1967). After extraction, the Al was dosed through atomic absorption spectrophotometry (PerkinElmer® AAnalyst 200). Al-total was determined through titration with ZnSO4 0.0156 mol L-1 after sulfuric digestion (20 mL of H2SO4 diluted 1:1) for half hour in heater plate at 70°C (Donagema et al., 2011). Analysis of variance was performed in order to evaluate the effect of gypsum application, incubation time and the interaction between these factors in each layer individually. When significant qualitative effects were observed, the Scott-Knott test (p<0.05) was applied.
For significant quantitative effects, regression analysis (p<0.05) was used and the models were selected based on the significance of the regression coefficients and on the magnitude of the coefficient of determination.
Gypsum incubation for up to 90 days and the amount of water used in the irrigation, combined with the rainfall that occurred along the experiment, allowed the calcium and sulfate from the mineral gypsum applied on surface to move to soil deeper layers (Figure 3). The content of exchangeable Ca increased linearly with gypsum incubation time in all soil layers (Figure 3A) and S-SO42- content also increased over time, but stabilized at different levels according to the soil layer (Figure 3B).
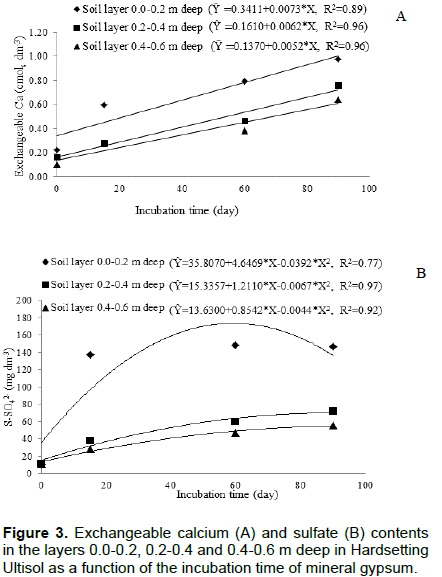
In 90 days, the exchangeable Ca content was more than double of the initial content in the layer 0.4 to 0.6 m deep (Figure 3A). The curvilinear behavior S-SO42- content as a function of incubation time revealed migration of the anion, particularly in the layer 0 to 0.2 m deep. From about 59 days after gypsum application, the upper layer started to provide S-SO42- to the deeper soil layers (Figure 3B). Maintaining the curvilinear behavior S-SO42- content as a function of incubation time of the gypsum in the final layer showed that the S-SO42- continue moving in the soil profile. The increasing gypsum applications caused a high increment in the exchangeable Ca and S-SO42- contents in the layer of 0.0 to 0.2 m deep and a short increment in the layer 0.2 to 0.4 m deep (Figure 4). In the layer of 0.4-0.6 m deep,there was no effect of the applied amount of gypsum on the exchangeable Ca content (Figure 4A). The increment in the S-SO42- content by gypsum application stabilized with 8.79 Mg ha-1 in layer 0.2-0.4 m deep and 6.90 Mg ha-1 in layer 0.4-0.6 m deep (Figure 4B). Thus, based on the measurements of these two variables, it could be observed that gypsum moved vertically in the soil (Figure 3), but the applied amounts did not cause differences in this movement (Figure 4).
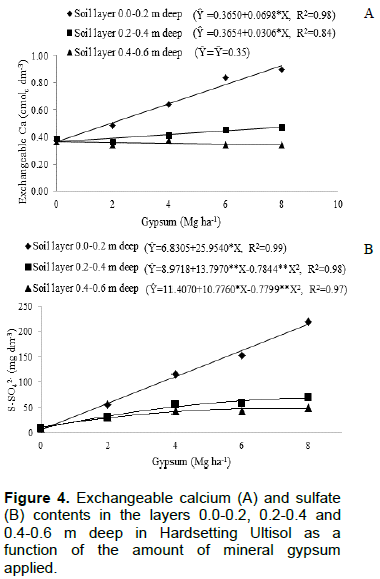
Increasing amounts of gypsum applied caused no effect on soil pH (Figure 5A), but soil pH (water) decreased with gypsum application time in all evaluated layers (Figure 5B). The decrease was curvilinear reaching a minimum value and then rise. The pH (water) decrease was greater in soil deeper layers, but this decrease in all layers only occurred until about 60 days after gypsum application (Figure 5B).
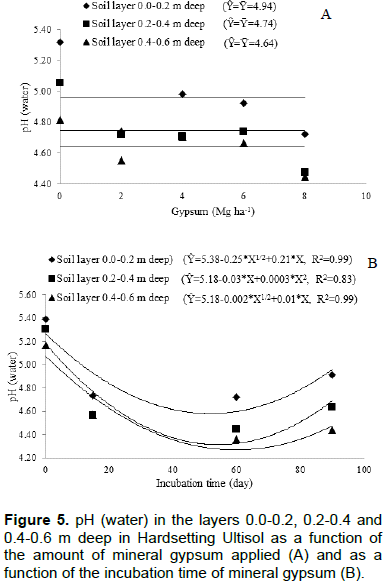
The pH (water) data in short periods of time are variable, especially when using gypsum or lime in the soil. To avoid this and to ascertain whether gypsum promotes or not change in soil pH, it was determined the pH in CaCl2 0.01 mol L-1 solution. Thus, it can be seen that neither time nor increasing amounts of applied gypsum changed soil pH (Figure 6). The amount of applied gypsum little modified exchangeable Al, showing effect only on layer 0.4 to 0.6 m deep (Figure 7A). The reduction in exchangeable Al content was 0.006 cmolc dm-3 for each Megagram of applied gypsum. The contents of exchangeable Al as a function of gypsum application time did not change in any soil layer, despite the increase in contents of exchangeable Ca and S-SO42- caused by application time of gypsum (Figure 7B).
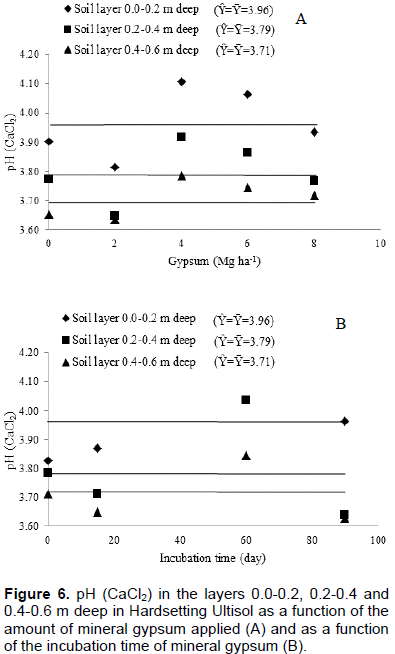
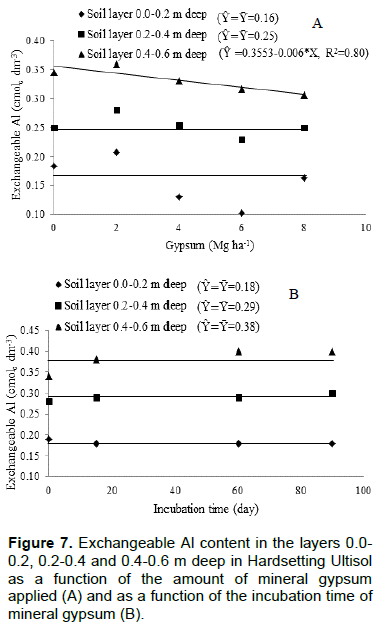
In superficial layer, 15 days after gypsum application, the content of exchangeable Ca increased by approximately three times and the content of S-SO42- by approximately seven times, in relation to initial contents in the soil (Table 1 and Figure 3); however, the content of exchangeable Al did not change (Figure 7). Specifically in this soil layer, Al-organic matter was responsible for buffering the Al, because there was a significant decrease in the Al of this compartment (Table 2).
The reduction of Al-organic matter also occurred in the layer, 0.2 to 0.4 m deep (Table 3), where C contents remained high, as in the superficial layer (Table 1). Thus, this compartment, also in this layer, continued buffering the soil, because exchangeable Al content did not change with gypsum application (Figure 7).
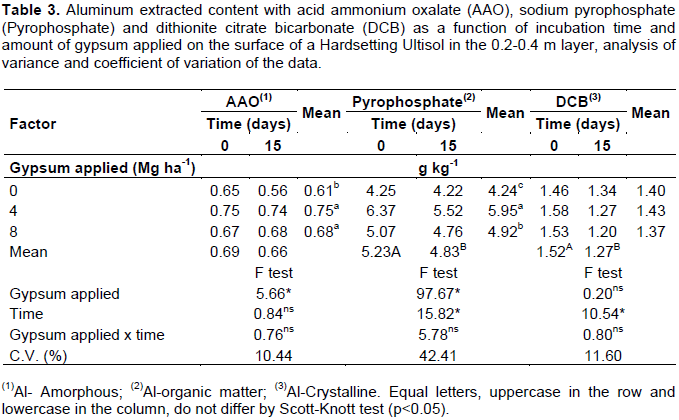
In the subsequent layer, since C content decreased (Table 1), there was no significant reduction in Al-organic matter (Table 4). As soil depth increased, this compartment stopped being responsible for buffering the exchangeable Al. Gypsum application did not influence the Al-organic matter content in the superficial layer, although the use of 4 and 8 Mg ha-1 gypsum has reduced 0.56 and 1.08 g kg-1 the Al-organic matter content, respectively, as compared to treatment where gypsum was not applied (Table 2). Gypsum incubation time this layer was more effective in exchangeable Al buffering by Al-organic matter than the amount gypsum applied. In 0.2 to 0.4 m layer, Al-organic matter compartment increased with increment in the applied amounts of gypsum (Table 3). This compartment, besides buffering the soil can also complex this same Al in reactive less forms of organic matter.
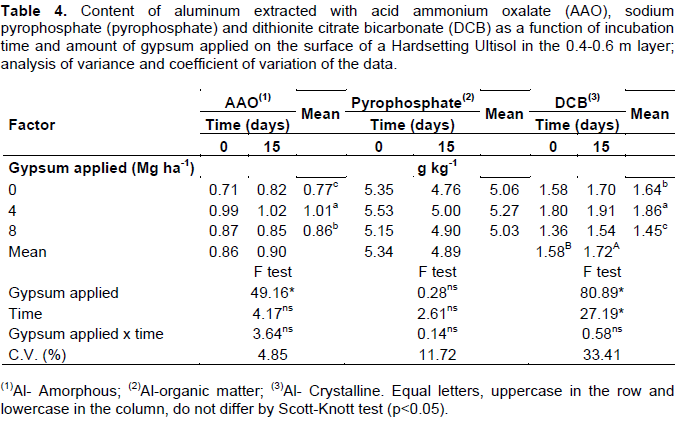
Al-crystalline forms became responsible for the buffering of exchangeable Al as depth increased (Tables 2, 3 and 4). Al extracted using dithionite citrate bicarbonate (DCB) decreased significantly in the layer of 0.2-0.4 m deep as a function gypsum incubation time (Table 3) and in the layer 0.4-0.6 m deep as a function amount gypsum applied (Table 4). Al-crystalline forms in deeper layers controlled the buffering of exchangeable Al, not allowing gypsum to act on the reduction of this Al form in the soil (Figure 7).
Gypsum incubation time increased Al-crystalline content in the layer, 0.4-0.6 m deep (Table 4). However, in higher gypsum application rate, Al-crystalline was reduced. This Al compartment buffered soil exchangeable Al in high gypsum applied rate, but over time others Al-crystalline forms may have been reconstructed.
At the lowest gypsum applied dose, Al-crystalline and Al-amorphous content increased, and at higher gypsum doses they were reduced. The gypsum appropriate dose to correct the exchangeable Al was about 4 Mg ha-1. So, all the exchangeable Al can have be neutralized and Al-crystalline and Al-amorphous increased. Excess exchangeable Ca provided by the high amount of gypsum applied can have moved others form of Al such as low crystallinity Al, for example. Buffering of this layer appears to have been performed by Al-crystalline and Al-amorphous, because the Al-organic matter in the deepest soil layer does not act in buffering.
Interaction between applied gypsum and incubation time was not statistically significant at any Al-compartment and soil layer. The main effects were independent. For example, treatment without gypsum application showed the same behavior as the treatments with application gypsum. Therefore, there were no other factors that may have interfered with the Al-compartments content in this study.
The capacity of gypsum to modify soil chemical characteristics can be evaluated through the increase in the exchangeable Ca and S-SO42- contents in cationic and anionic adsorption sites, respectively (Caires et al., 2011; Santos et al., 2013b), as observed in the present study (Figures 3 and 4), and also through its movement and action in deeper soil layers, promoting alterations in exchange complex in subsurface.
The slower displacement of S-SO42- and its later saturation can be attributed to some reasons: S-SO42- displaces, through mass action, the OH- ions adsorbed onto the positively charged surface of oxides and clay minerals, occupying their spaces; S-SO42- in solution complexes exchangeable Al, forming insoluble Al2(SO4)3; S-SO42- can also form ionic pairs with exchangeable Al, such as AlSO4+; there is also a preferential adsorption of S-SO42- by the exchangeable Al, in relation to the exchangeable Ca (Farina et al., 2000).
Reduction in soil pH (water) is commonly observed in study with gypsum application and incubation (Figure 5), however, this effect is due the action of soluble salts and increase of the electrolyte concentration that displace exchangeable Al to the soil solution. Al is hydrolyzed producing H+ and lowering pH (water); therefore gypsum has no direct action on the soil pH (water) reduction (Ernani et al., 2001). According to Carvalho and Raij (1997), the pH measurement using solutions with defined concentrations provide the same ionic force in the pH measurement solution, such as CaCl2 0.01 mol L-1 (Figure 6).
Reduction in exchangeable Al contents in subsurface is an expected effect when gypsum is applied (Li et al., 2010). This decrease is attributed to S-SO42-, which, after forming ionic pair with exchangeable Al, removes it from the root zone, whether through in-solubilization [Al2(SO4)3] or leaching (AlSO4+) (Saldanha et al., 2007).
Evaluating the effectiveness of gypsum application in soil focusing only on exchangeable Al determination may compromise the evaluation of the gypsum action and lead to misunderstandings in the interpretation of the results (Figure 7). Al dynamics is complex and its adsorption/desorption is related to the activity of different soil Al compartments (Coelho et al., 2010).
The main Al compartments responsible for the buffering of exchangeable Al are associated with the organic matter, cation exchange capacity and soil amorphous minerals (Coelho et al., 2010) and are susceptible to modifications caused by chemical alterations in the soil, such as gypsum application (Tables 2, 3 and 4).
These reactions probably occurred in this soil, both in surface and subsurface. However, the Al-organic matter and Al-crystalline prevented gypsum from reducing the exchangeable Al content (Tables 2, 3 and 4; Figure 7). According to Pedrotti et al. (2003), there are forms of reactive Al weakly associated with organic matter, reactive Al strongly associated with the organic fractions and non-reactive Al strongly complexed with the organic matter.
The increasing amounts of gypsum applied in this study caused increase in Al-amorphous compartment in subsurface (Tables 3 and 4). According to Takahashi et al. (2006), gypsum application reduces the solubility of exchangeable Al through the formation of Al-hydroxides and Al-sulfate minerals of low crystallinity. Álvarez et al. (2012) in experiment using limestone as soil conditioner, and Takahashi et al. (2006) using phosphogypsum, also observed increase in soil Al-amorphous contents.
Childs et al. (1983) reported that the increase in Al-amorphous in subsurface can be indicative of Al translocated from upper layers. Al leaching is one of the forms through which gypsum acts to remove high amounts of exchangeable Al from the plant root zone.
Gypsum application increased exchangeable calcium and sulfur contents in subsurface and did not reduce the exchangeable aluminum content. Soil pH (water) decreased with gypsum application time in all evaluated layers; however, there was no difference in soil pH (CaCl2). Al-organic matter in the surface layer decreased along incubation time and gypsum application. Al-amorphous in subsurface layers increased with the increased amounts of gypsum. Al-crystalline decreased as soil depth increased. The buffering of exchangeable aluminum in surface layers was performed by Al-organic matter and, in subsurface, by Al-crystalline.
The authors declare that there is no conflict of interest.
REFERENCES
Álvarez E, Fernández-Sanjurjo MJ, Nú-ez A, Seco N, Corti G (2012). Aluminium fractionation and speciation in bulk and rhizosphere of a grass soil amended with mussel shells or lime. Geoderma 173-174:322-329.
Crossref
|
|
Alvarez V VH, Dias LE, Ribeiro Junior ES, Souza RB, Fonseca CA (2001). Methods of sulfur analysis in soils and plants. Federal University of Viçosa, Viçosa, MG, Brazil.
|
|
|
Caires EF, Joris HAW, Churka S (2011). Long-term effects of lime and gypsum additions on no-till corn and soybean yield and soil chemical properties in southern Brazil. Soil Use Manage. 27:45-53.
Crossref
|
|
|
Carvalho MCS, Raij BV (1997). Calcium sulphate, phosphogypsum and calcium carbonate in the amelioration of acid subsoils for root growth. Plant Soil 192:37-48.
Crossref
|
|
|
Childs CW, Parfitt R L, Lee R (1983). Movement of aluminium as an inorganic complex in some podzolised soils, New Zealand. Geoderma 29:139-155.
Crossref
|
|
|
Coelho MR, Vidal-Torrado P, Perez XLO, Martins VM, Vázquez FM (2010). Aluminum fractionation by techniques of selective dissolution in Spodosols the coastal plain of São Paulo state. Rev. Bras. Cienc. Solo 34:1081-1092.
Crossref
|
|
|
Donagema GK, Campos DVB, Calderano SB, Teixeira WG, Viana JHM (2011). Soil analysis methods manual. Embrapa, Rio de Janeiro, RJ, Brazil.
|
|
|
Ernani PR, Ribeiro MS, Bayer C (2001). Chemical modifications in acid soils caused by addition of gypsum or limestone. Sci. Agric. 58:825-831.
Crossref
|
|
|
Farina MPW, Channon P, Thibaud GRA (2000). Comparison of Strategies for Ameliorating Subsoil Acidity: II. Long-Term Soil Effects. Soil Sci. Soc. Am. J. 64:652-658.
Crossref
|
|
|
Li JY, Wang N, Xu RK, Tiwari D (2010). Potential of industrial byproducts in ameliorating acidity and aluminum toxicity of soils under tea plantation. Pedosphere 20:645-654.
Crossref
|
|
|
McKeague JA (1967). An evaluation of 0.1 M pyrophosphate and pyrophosphate-dithionite in comparison with oxalate as extractants of the accumulation products in Podzols and some other soils. Can J. Soil Sci. 47:95-99.
Crossref
|
|
|
Mehra OP, Jackson ML (1960). Iron oxide removal from soils and clays by dithionite-citrate system buffered with sodium bicarbonate. Clays Clay Min. 7:317-327.
Crossref
|
|
|
Pedrotti A, Ferreira MM, Curi N, Silva MLN, Lima JM, Carvalho R (2003). Relationship between physical attributes, clay fraction mineralogy and aluminum forms in soil. Rev. Bras. Cienc. Solo 27:1-9.
Crossref
|
|
|
Ramos LA, Nolla A, Korndörfer GH, Pereira HS, Camargo MS (2006). Reactivity of soil acidity correctives and conditioners in lysimeters. Rev. Bras. Cienc. Solo 30:849-857.
|
|
|
Reyes-Díaz M, Meri-o-Gergichevich C, Alarcón E, Alberdi M, Horst W (2011). Calcium sulfate ameliorates the effect of aluminum toxicity differentially in genotypes of highbush blueberry (Vaccinium corymbosum L.). J. Soil Sci. Plant Nutr. 11:59-78.
Crossref
|
|
|
Rocha AT, Oliveira AC, Rodrigues AN, Lira Junior MA, Freire FJ (2008). Araripe gypsum use in improving the environment root of sugarcane. Rev. Bras. Ciênc. Agrár. 3:307-312.
Crossref
|
|
|
Saldanha ECM, Rocha AT, Oliveira ECA, Nascimento CWA, Freire FJ (2007). Use of the mineral gypsum on Latosol cultivated with sugar cane. Caatinga 20:36-42.
|
|
|
Santos HG, Jacomine PKT, Anjos LHC, Oliveira VA, Lumbreras JF, Coelho MR, Almeida JA, Cunha TJF, Oliveira JB (2013a). Brazilian system of soil classification. Embrapa, Brasília, DF, Brazil 353 p.
|
|
|
Santos RL, Freire FJ, Azevedo VM, Rocha AT, Tavares JA (2013b). Yield of elephant grass and movement of cations as a function of mined gypsum. Rev. Bras. Eng. Agric. Ambient. 17:1030-1037.
Crossref
|
|
|
Schwertmann U (1964). Differenzierung der eisen oxide des bodens durch extraktion unit saurer ammoniumoxalat-losung. Zeitung Pflanzenernährung 105:194-202.
|
|
|
Soil Survey Staff (1998). Keys to soil taxonomy. USDA-NRCS, Washington, DC, EUA.
|
|
|
Takahashi T, Ikeda Y, Nakamura H, Nanzio M (2006). Efficiency of gypsum application to acid Andosols estimated using aluminum release rates and plant root growth. Soil Sci. Plant Nutr. 52:584-592.
Crossref
|
|