ABSTRACT
Sugarcane production can be affected by extreme environmental conditions, such as drought stress. This study focused on establishing a screening method to evaluate and select drought tolerant germplasm of Saccharum species. A new drought screening methodology is presented in this paper. A randomized block design experiment was conducted under greenhouse conditions with 2 months old seedlings of five genotypes of Saccharum spontaneum (IRAN 28, JW43, TUS12-72, TUS12-23, X08-0299) and one genotype of a commercial cultivar (Saccharum spp. CP72-1210), under well-irrigated and water stress conditions. Three treatments were established with irrigation intervals at 3, 6, and 9 days, with four replicates per each genotype in each treatment. Evapotranspiration, stomatal conductance, total biomass, leaf death rate, rate of new tillers and shoot growth were evaluated during the experiment. JW43 and CP72-1210 presented the highest values of stomatal conductance with non-significant differences. Accumulated water used by all the genotypes was significantly different among the three treatments. Also, significant differences were found in the total dry biomass among all the treatments. Results showed that TUS12-23 genotype can be classified as very tolerant to drought stress and JW43 is the less tolerant, whereas the other genotypes could be susceptible and may survive under mild drought conditions. Results also indicated that the methodology used, and the parameters measured are effective in identifying sugarcane germplasm with extreme reaction to drought stress.
Key words: Drought stress, Saccharum species, biomass, stomatal conductance, agronomic parameters.
Sugarcane (Saccharum species) is a crop of economic importance worldwide for producing sugar and considered as an essential renewable source of biofuel (Prabu et al., 2011). It’s a tall growing monocotyledonous crop that belongs to the genus Saccharum L., of the tribe Andropogoneae in the grass family (Poaceae) which is cultivated in the tropical and subtropical regions of the world. The Saccharum complex comprises of Saccharum, Erianthus section Ripidium, Miscanthus section Diandra, Narenga and Sclerostachya (Daniels and Roach, 1987). Modern sugarcane varieties that are cultivated for sugar production are founded on interspecific hybrids between Saccharum spontaneum and Saccharum officinarum (Saccharum spp.). This specie can be adapted to different environmental conditions; however, drought stress can limit the amount of sugarcane establishment and growth in many world regions because sugarcane requires substantial amounts of water during early stages (Wiedenfeld, 2008).
The limitation of drought stress on sugarcane productivity has been the subject of several studies (Inman-Bamber and Smith, 2005; Smit and Singels, 2006; Silva et al., 2008; Singels et al., 2010). Generally, water shortage results in a negative impact on establishment of the crop, especially if the drought duration exceeds the capacity of drought tolerance of the plant species (Smit and Singels, 2006). Under soil water-deficit conditions, plants show adaptive mechanisms and/or responses that allow them to survive. One alternative to mitigate water deficit in sugarcane is irrigation (Inman-Bamber, 2004); however, water is limited in some regions, and equipment costs make this strategy expensive (Silva et al., 2007). Therefore, studies aimed at selecting drought-tolerant cultivars are a viable alternative to increase productivity and a necessity for the future (Prabu et al., 2011).
Water-deficit stress at early stage alters a variety of growth and physiological processes in sugarcane, which cause decreased yields (Zhang et al., 2001; Silva et al., 2007). Water deficit during establishment can trigger a negative impact upon growth and development of the crop, compromising plant productivity (Inman-Bamber, 2004). Moderate water deficit causes significant morphological and physiological changes in sugarcane establishment (Creelman et al., 1990) while severe deficit may lead to plant death (Cheng et al., 1993). Despite the existence of a significant amount of research on these physiological variables such as stomatal conductance, and others (Silva et al., 2014), little have focused on their interaction with agronomic parameters such as crop productivity and growth rate. Such traits can be measured as an alternative method of characterizing tolerant and susceptible genotypes in sugarcane breeding programs. Silva et al. (2008) found differences in productivity and yield components of 80 sugarcane genotypes grown under two water regimes, with and without water deficit stress. Under stress, the authors found that some varieties, considered drought-tolerant, showed a higher productivity, greater stem mass than sensitive varieties, showing a positive correlation between these morphological variables and total biomass production.
Generally, the drought tolerance is assessed by the combination of physiological and agronomic parameters response to water stress (Ferreira et al., 2017). Additionally, assessing the biomass production per water unit could give an idea about the plant survival under water deficit. The development of screening methodologies for tolerance of
S. spontaneum for abiotic stresses, such as drought is very important for breeding of bioenergy crops (Da Silva, 2017). Sugarcane biomass yields per hectare vary widely (25 to 35 dry t·ha
-1). Newly-developed energy canes and wide hybrids involving
Saccharum spp. have shown even higher biomass yield potentials, 30 to 45 dry t·ha
-1 (Da Silva, 2017) and higher water productivities under the long growing season conditions in parts of the Southeast U.S. The objective of this study was to develop an agronomic screening approach to evaluate and select drought tolerant genotypes of
Saccharum spp.
A greenhouse experiment was conducted at Weslaco, Texas, USA (26°12′N, 97°57′W), from August to October 2016 to evaluate one commercial sugarcane cultivar (Saccharum interspecific hybrid, CP72-1210) and five genotypes of energy cane S. spontaneum (IRAN 28, TUS12-72, TUS12-23, X08-0299, and JW43) under drought conditions. The plant materials selected for the experiments were 2 months old seedlings with uniform height and crop development. A randomized block design experiment was conducted with three treatments and four replications per each genotype. The treatments denoted as A, B, and C consisted in applying irrigation to field capacity at intervals of 3, 6, and 9 days, respectively. The treatments represented a well irrigated treatment (treatment A) and water stress conditions (treatments B and C).
The water used by the crop (crop evapotranspiration) was calculated by the difference in pot weight before and after each irrigation event. To intensify the drought stress in the plants, at the sixth weeks of the experiment, treatments A, B, and C were adjusted to replace 50, 25 and 10% of the amount of water by the plants which was calculated from the average of the evapotranspiration data recorded during the first experimental six weeks, by which 250, 150, and 100 mL of water were added every 3 days to the treatments A, B, and C, respectively. Accumulated crop evapotranspiration (the total water used for each treatment), was estimated at the end of the season by summing the water used during all the irrigation intervals. Several methods and measurements were used to screen the plants for drought stress. Other agronomic measurements were rate of tillers and plant height that used to estimate the growth rate per week. A measure stick was used to measure plant height from the base until the top of the main stalk.
Total fresh and dry biomass was calculated at the end of the experiment from the difference in the fresh and dry weight of the shoots and roots. At the end of the experimental period, the numbers of dead leaves were counted to calculate the percent of dead leaves in each plant. Moreover, the plants were collected and separated by stems, leaves, and roots at the end of the experimental period and oven-dried for three days at 110°C to measure dry matter. The physiological parameter of stomatal conductance (gs) was measured once per week during the experimental period, in one young adult leaf per pot. The leaf measurements were taken with a leaf porometer (SC-1, Decagon Devices Inc., Pullman, WA) between 9:00 and 11:00 h to avoid the high temperatures and low relative humidity of the afternoon that can cause a high leaf-to-air vapor pressure deficit that close stomata (Hu et al., 2009). All the biomass data were analyzed using a two-way variance (Analysis of Variance, ANOVA), StatPlus statistical packages, StatPlus, and AnalystSoft Inc.-Statistical analysis program, Version 6 (http://www.analystsoft.com/en/) were analyzed using Tukey’s test for mean separations (P≤0.05) when a significant F-test was observed.
Total water used by the different genotypes
The evapotranspiration rate recorded in the studied genotypes during the first experimental six weeks is shown in
(Figure 1). Among all genotypes, TUS12-23 consistently presented the lowest evapotranspiration rate under well-watered (treatment A, irrigation every 3 days) and drought stress conditions (treatment B and C, irrigation every 5 and 9 days, respectively). In contrast, JW43 presented the highest evapotranspiration rate under well-watered conditions, whereas no significant differences were found under drought conditions. Cumulative amounts of water use per each genotype during the whole experimental period (twelve weeks) are shown in (Figure 2).
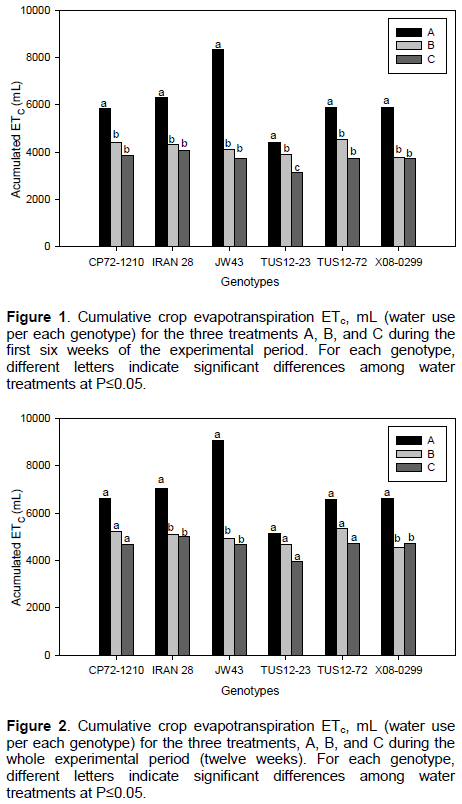
Total amounts of water use were not significantly different among the three irrigation treatments A, B, and C for genotypes TUS 12-23, CP72-1210, and TUS 12-72. No statistical differences among treatments B and C were observed for genotypes IRAN 28, JW43, and X08-0299. Considering the whole experimental period of twelve weeks (Figure 2), the genotype JW43 showed the highest amount of water use in treatment A with approximately 9043 mL, followed by IRAN28, CP72-1210, TUS12-72, X08-0299, and TUS12-23, respectively, whereas the lowest amount of water use for the same treatment A was observed for TUS12-23 with approximately 5124 mL (Figure 2). Non-significant differences on total water use for each genotype were observed for treatments B and C. There was a slight numerically decrease in water use between treatment B and C among all genotypes except in genotype X08-299.
The range of stomatal conductance values observed for all genotypes during the experiment varied from 3 to 90 mm H2O m-1. Overall the experimental period, there was not any observable trend (Figure 3) that reflects variation changes in stomatal conductance between genotypes and treatments. However, analyzing the measurements of each individual date showed significantly higher values of stomatal conductance (92 and 88 mm H2O m-1) in CP72-1210 and JW43 in some days, compared with the other genotypes that gave values less than 68 mm H2O m-1. These same varieties (CP72-1210 and JW43) were among the varieties that resulted in the highest cumulative evapotranspiration. Lower numerically stomatal conductance with non-statistical differences was observed for all the genotypes under irrigation treatments B and C.
Growth rate and yield production
Shoots dry weight (SDW) values of CP72-1210 were not significantly affected by drought stress (
Table 1), the reduction of SDW from the control treatment to drought treatments was very low, similar results observed in IRAN 28, TUS12-23 and TUS12-72 genotypes. The greatest of SDW reduction from treatment A to treatment B were observed for the JW43 and X08-0299 genotypes (35.14 and 42.78%, respectively). Statistical differences were found in roots dry weight (RDW) in CP72-1210, IRAN 28, and JW43. The reduction of RDW from treatment A to B was 31.9 and 41.1% for the IRAN 28 and X08-229 genotypes.

The RDW increase of genotype CP71-1210 from treatment A to B was probably caused by the variability of the genotypes characteristics. Small numerical reductions were observed in the RDW of TUS12-72. Statistically significantly reduction of total dry weight (TDW) was observed for the IRAN 28 (16.91%), JW43 (37.02%), and X08-299 (42.44%) genotypes from the well-water treatment (3 days interval) to the drought treatment (6 days interval). TDW values showed that the IRAN 28 was less affected by drought conditions than the other genotypes, and the most affected genotypes for TDW drought tolerance was X08-299. The percent of dead leaves at the end of the experiment showed that the CP72-1210 was the least affected treatment under the well-watered conditions. However, it statistically increased under drought conditions. It is important to mention that all of the genotypes, except CP72-1210 under treatment A, presented a
high percent of dead leaves.
The highest numerical percent value of dead leaves was observed for the drought treatments of genotypes JW43 and X08-299 for treatment C (irrigation interval of 9 days). The rate of new tillers decreased significantly under drought conditions for the JW43 genotype. For the other genotypes, the rate of tillers of the well-water treatments (A) was compared with the drought treatments (B and C), and no statistically differences were observed. However, numerically higher rate of tillers was observed for genotypes IRAN28 and X08-0299 under the drought treatment (treatment C). No statistical differences on growth rate were observed among genotypes of the three treatments.
The aim of this study was focused on establishing a screening method to evaluate and select drought tolerant genotypes of Saccharum spp. during the early growth phase. The data showed that there were statistical differences in the amount of water use among the studied genotypes. TUS12-23 consistently showed the lowest water consumption under well-watered and drought conditions, and there was not significant reduction in biomass, new tillers produced, and percent of dead leaves. On the other hand, JW43 genotype was the highest consumer of water for the well irrigated treatment A followed by IRAN 28, X08-0299, CP72-1210, TUS12-72, and TUS12-23, respectively. This same genotype resulted in the highest total biomass for the irrigation treatment A, but when it was submitted to drought conditions led to high reduction of the total dry biomass which was approximately 51%. Water consumption for IRAN 28, X08-0299, CP72-1210, and TUS12-72 resulted in intermediate values between the two extreme values of the TUS12-23 and JW43 genotypes. For the IRAN 28 genotype, the reduction of total dry biomass from the well-water treatment A to the drought treatment C was approximately 40%. There was a significant difference of the amount of water used between well-watered and drought conditions in the X08-0299 genotype, and the reduction of yield was reduced 53.3% of the total produced biomass. Whereas, the reduction of water use under drought treatments in TUS12-72, CP72-1210, and TUS12-23 genotypes resulted in non-significant differences in the total dry biomass; however there were slightly numerical differences.
Results showed that JW43 and X08-0299 were the lowest total dry biomass and the highest percent of dead leaves which indicate that these genotypes cannot be adapted to drought conditions either moderate or severe water stress conditions as in treatments B and C, respectively. The rate of new tillers decreased significantly under drought conditions for the JW43 genotype. For the other genotypes, the rate of tillers of the well-water treatments (A) was compared with the drought treatments (B and C), and no statistical differences were observed. However, numerically higher rate of tillers was observed for genotypes IRAN28 and X08-0299 under the drought treatment (treatment C). The JW43 and IRAN 28 varieties that produced more biomass under well-water conditions, they were also the ones that suffered the greatest reductions of biomass, and the greatest reductions of the number of tillers. The reduction in the number of tillers due to water stress was reported by Robertson et al. (1999); Silva and Costa (2004); Singh and Reddy (1980) and Soares et al. (2004). Ramesh and Mahadevaswamy (2000) reported that the formation of tillers in sugarcane is important because of the contribution they make to the crop yield by acting as a storage sink. Joshi et al. (1996) also reported that the tillering ability and subsequent growth efficiency largely determine the yield of a given cultivar.
The obtained results give an indication that JW43 and X08-0299 are intolerant drought genotypes and very sensitive to water shortage. Therefore, submitting these genotypes to severe water stress may lead to plant death, band could cause high reduction in the yield production. Sonia et al. (2012) reported that drought events can significantly decrease sugarcane productivity during the establishment stage. Several more studies have highlighted the effect of water stress on sugarcane (Jones and Ritchie, 1990). Ramesh and Mahadevaswamy (2000) also reported the reduction of biomass of these genotypes can be attributed to sucrose accumulation caused by water stress. On the other hand, TUS12-23 and CP72-1210 genotypes demonstrated high tolerance to drought stress, where the total dry biomass was slightly numerically reduced (less than 15.6 and 24.4%, respectively), as compared to well-watered conditions and non-significant differences were found in reduction rate of new tillers between drought and well-watered conditions. Whereas IRAN 28 genotype showed that it could be susceptible for drought stress, the reduction of total biomass under drought conditions was 40%, however the rate of new tillers was not affected by drought stress; similar results found in the percent of dead leaves which indicated that this genotype could tolerate moderate drought stress and sustain a rational production for prolonged periods.
According to Inman-Bamber and Smith (2005), plants may avoid or delay water stress by limiting transpiration through stomatal closure or by reducing exposed leaf area through leaf rolling. Statistical differences were found in stomatal conductance values among the well-watered and drought stress treatments in CP72-1210 and JW43 genotypes. However, no statistical differences were found in the other genotypes, which indicate that the studied genotypes may need prolonged stress periods to show differences in stomatal conductance and other physiological parameters. Therefore, as a preliminary study that bases on an agronomic approach for a rapid screening of drought tolerance, TUS12-23 genotype can be classified as very tolerant to drought stress and JW43 as the less tolerant genotype, whereas the other genotypes could be susceptible and may survive under mild drought conditions. The results of this study showed that S. spontaneum genotypes presented extreme reactions to drought stress, which is consistent with the great phenotypic variability presented by these species, which is found in environments with different and extreme conditions, including flood basins and deserts.
The physiological parameters such as stomatal conductance may provide reliable information on the impact of water stress on sugar and energy cane genotypes. However, it requires prolonged periods to obtain significant differences, which might not be a good option for some genotypes that cannot be submitted to prolonged stress conditions. Therefore, an agronomical approach could be a useful tool for rapid screening of sugar and energy cane genotypes for drought stress. The study showed significant differences were found in the response of sugar and energy cane genotypes to different drought stress levels under the evaluation of the total amounts of water use, total dry biomass, the rate of new tillers and percent of dead leaves.
The authors have not declared any conflict of interests.
REFERENCES
Cheng Y, Weng J, Joshi CP, Nguyen HT (1993). Dehydration stress-induced changes in translatable RNAs in sorghum. Journal of Crop Science, 33:1397-1400.
Crossref
|
|
Creelman RA., Mason HS, Bensen RJ, Boyer JS, Mullet JE (1990). Water Deficit and Abscisic Acid Cause Differential Inhibition of Shoot versus Root Growth in Soybean Seedlings: Analysis of Growth, Sugar Accumulation, and Gene Expression. Plant Physiology, 92:205-214.
Crossref
|
|
|
Da Silva JA (2017). The Importance of the Wild Cane Saccharum spontaneum for Bioenergy Genetic Breeding. A Review. Sugar Technology.
Crossref
|
|
|
Daniels J, Roach BT (1987). Taxonomy and evolution. Chapter 2. In: DJ Heinz, ed. Sugarcane improvement through breeding, Volume 11. Elsevier, Amsterdam, Netherlands, pp. 7-84.
Crossref
|
|
|
Ferreira TH, Tsunada MS, Bassi D, Araújo P, Mattiello L, Guidelli GV, Menossi M (2017). Sugarcane water stress tolerance mechanisms and its implications on developing biotechnology solutions. Frontiers in Plant Science, 8:1077.
Crossref
|
|
|
Hu MJ, Guo Y-P, Shen Y-G, Guo D-P, Li D-Y (2009). Midday depression of photosynthesis and effects of mist spray in citrus. Annals of Applied Biology, 154:143-155.
Crossref
|
|
|
Inman-Bamber NG (2004). Sugarcane water stress criteria for irrigation and drying off. Field Crops Research, 89:107-122.
Crossref
|
|
|
Inman-Bamber NG, Smith DM (2005). Water relations in sugarcane and response to water deficits. Field Crop Research, 92:185-202.
Crossref
|
|
|
Jones JW, Ritchie JT (1990). Crop growth models In: (Hoffman G.J., Howell T.A., Solomon K.H. eds). Management of farm irrigation systems ASAS Monograph No 9 Michigan USA, pp. 80-86.
|
|
|
Joshi HC, Pathak H, Choudhary A, Kalra N (1996). Distillery effluents as a source of plant nutrient: Problems and prospects. Fert. News, 41(11):41-47.
|
|
|
Prabu G, Kawar PG, Pagariya MC, Prasad DT (2011). Identification of water deficit stress upregulated genes in sugarcane. Plant Molecular Biology Reporter, 29:291-304.
Crossref
|
|
|
Ramesh P, Mahadevaswamy M (2000). Effect of formative phase drought on different classes of shoots, shoot mortality, cane attributes, yield and quality of four sugarcane cultivars. Journal of Agronomy Crop Sciences, 185:249-258.
Crossref
|
|
|
Robertson MJ, Muchow RC, Donaldson RA., Inman-Bamber NG, Wood AW (1999). Estimating the risk associated with drying-off strategies for irrigated sugarcane before harvest. Australia Journal of Agricultural Research, 50:65-77.
Crossref
|
|
|
Silva ALC, Costa WAJM (2004). Varietal variation in growth, physiology and yield of sugarcane under two contrasting water regimes. Tropical Agricultural Research, Peradeniya, 16:1-12.
|
|
|
Silva M de A, Jifon JL, Da Silva JAG, Dos Santos CM, Sharma V (2014). Relationships between physiological traits and productivity of sugarcane in response to water deficit. Journal of Agricultural Science, 152:104-118.
Crossref
|
|
|
Silva MA, Silva JAG, Enciso J, Sharma V, Jifon J (2008). Yield components as indicators of drought tolerance of sugarcane. Science Agricola, 65:620-627.
Crossref
|
|
|
Silva MDA, Jifon JL, Silva JAG, Sharma V (2007). Use of physiological parameters as fast tools to screen for drought tolerance in sugarcane. Brazilian Journal Plant Physiology, 19:193-201.
Crossref
|
|
|
Singels A, Van Den Berg M, Smit MA, Jones MR, Van Antwerpen R (2010) Modeling water uptake, growth and sucrose accumulation of sugarcane subjected to water stress. Field Crop Research, 117:59-69.
Crossref
|
|
|
Singh S, Reddy MS (1980). Growth, yield and juice quality performance of sugarcane varieties under different soil moisture regimes in relation to drought resistance. In: International Society of Sugar Cane Technologists Congress, 17, Manila. Proceedings... Manila: ISSCT, 1:541-555.
|
|
|
Smit MA, Singels S (2006). The response of sugarcane canopy development to water stresses. Field Crop Research, 98:91-97.
Crossref
|
|
|
Soares RAB, Oliveira PFM, Cardoso HR, Vasconcelos ACM, Landell MGA, Rosenfeld U (2004) Efeito da irrigação sobre o desenvolvimento e a produtividade de duas variedades de cana-de-acucar colhidas em inicio de safra. STAB – Açúcar Álcool e Subprodutos, Piracicaba, 22(4):38-41.
|
|
|
Sonia MZ, Fabiana AR, Jose PG, Livia MP, Mirian VL (2012). Sugarcane Responses at Water Deficit Conditions, Water Stress, Brazil, pp. 256-275.
|
|
|
Wiedenfeld B (2008). Effects of irrigation water salinity and electrostatic water treatment for sugarcane production. Agricultural Water Management, 95:85-88.
Crossref
|
|
|
Zhang MQ, Li GJ, Chen RK (2001). Photosynthesis characteristics in eleven cultivars of sugarcane and their responses to water stress during the elongation stage. Proceedings - International Society of Sugar Cane Technologists, 24:642-643.
|
|