ABSTRACT
Salicylic acid (SA) is used in agricultural practices because it induces stress tolerance. The goal of this study was to determine the SA concentration in leaves, the change in catalase (CAT) activity and the change in total antioxidant capacity (TAC) that result from the exogenous application of SA (10-4 M) to tomato leaves every 15 days in a greenhouse with and without NaCl application. Fruit yield was also verified. Treatments included SA + 100 mM NaCl, SA + 0 mM NaCl, water + 100 mM NaCl, and water + 0 mM NaCl. As a response to the foliar application of SA, the concentration of SA in leaf tissue exhibited a dynamic behavior that was similar to that reported when SA was induced by environmental stress. A similar phenomenon was observed for CAT and TAC activity after application of SA to leaves: CAT and TAC values increased at 24 h, reached their highest values at 48 h and began decreasing at 144 h, closely following the initial dynamics of SA concentration after each application. The spray application of SA in the absence of NaCl significantly increased the concentration of SA (up to 145 and 289% for the first and second application respectively) and CAT activity (up to 182% for the third application) in leaves, without affecting fruit yield. The application of SA in combination with 100 mM NaCl had the highest values of SA (up to 381 and 258% for the second and third application respectively) and CAT activity (up to 142 and 294% for the first and third application respectively), ​​without changing consistently the TAC of the leaves and without any effect on fruit production. The application of 100 mM NaCl did not affects the CAT activity, TAC or SA into the leaves, or fruit production.
Key words: Plant stress tolerance, elicitors, salicylates, antioxidants.
Salicylates are involved in the control of plant growth and development and also function as signals in environmental adjustment processes (Ashraf et al., 2010). Salicylic acid (SA) is a natural plant salicylate that regulates growth and tolerance to abiotic (Hussain et al., 2011) and biotic stresses. Specifically, SA aids in tolerance to biotic stress by regulating internal signals or activating genes that stimulate the production of various plant defense compounds, including those involved in responses to disease, pathogens and predators (Zhang et al., 2009). The application of exogenous salicylic acid (1) induces stress tolerance mechanisms, thereby improving the biological response of the plant to adverse growth factors (Poór et al., 2011) and (2) modifies a large number of metabolic and physiological responses, including the concentration of photosynthetic pigments, minerals, carbohydrates, proteins (Azooz and Yousef, 2010), metabolites of the phenylpropanoid pathway (Mustafa et al., 2009) and certain antioxidants (Azooz, 2009).
The utilization of SA in agricultural practices has yielded beneficial results in diverse crops (Noreen and Ashraf, 2009; El-Khallal et al., 2009; Azooz and Youssef, 2010), and it has become clear that the commercial use of these compounds decreases pesticide use (Esmailzadeth et al., 2008). Scientific literature and technical studies performed for the development and legal registration of products that contain such tolerance inductors as SA (with the objective of modifying tolerance to a certain environmental factor or increasing plant yield) describe the biochemical, physiological and morphological changes that occur in plants in response to the application or induction of SA (Azooz and Youssef, 2010). However, little is known about SA concentration dynamics when it is applied exogenously to plant tissues, and the effect of SA on certain biochemical traits, such as antioxidant capacity, is unclear. This information would aid in improving the agricultural use of this compound.
Our present study aimed to determine changes in (1) SA concentration, (2) catalase (CAT) activity and (3) total antioxidant capacity (TAC) after exogenous application of SA to tomato (Solanum lycopersicum L.) in a greenhouse under two salinity conditions (induced with NaCl). The effect of these factors on fruit production was also verified.
Location of the assay
The study was performed using the facilities of the Universidad Autónoma Agraria Antonio Narro, Buenavista, Saltillo, Coahuila, México.
Plant material
Tomato (Solanum lycopersicum L.) cv. Rio Grande (Petoseed) plants were used for all experiments. Seeds were planted in Berger BM2® commercial mix (thin peat:perlite:vermiculite 70:15:15 v/v) in polystyrene trays with 200 cavities. When the plants had produced three true leaves, the seedlings were transplanted to 20 L pots containing the same substrate. Plants were fertilized beginning on the fifth day after transplant using a Steiner solution (Steiner, 1961) diluted to 25%; when the first fruits appeared (35 days after transplant), full-strength (100%) Steiner solution was applied.
SA and NaCl treatment and application
Four treatments were utilized to examine the effects of two experimental factors: SA application to leaves and NaCl application to soil. SA was applied to leaves at a concentration of 10-4 M (13.8 mg L-1) using reactive grade SA (C7H6O3, CAS 67-72-7, CTR Scientific, Mexico), and NaCl was applied to soil at a concentration of 0 or 100 mM in culture medium. The four treatments were as follows: (1) Application of SA to leaves + 0 mM NaCl to soil; (2) Application of SA to leaves + 100 mM NaCl to soil; (3) application of water to leaves + 100 mM NaCl to soil, and (4) Application of water to leaves + 0 mM NaCl to soil (control). Each treatment was repeated 50 times.
Water and SA were applied to leaves at 15, 30 and 45 days after transplant during the early morning with a manual sprinkler using volumes that were large enough to achieve total coverage of the leaf area. A plastic barrier was set in between pots to prevent contamination between treatments.
NaCl (CTR Scientific, Mexico) was added to Steiner nutritional solution (Steiner, 1961) and applied beginning at 10 days after transplant. This solution was applied every two days until day 30 after transplant and daily thereafter until day 60. For all treatments, the substrate was washed every 10 days with water (1 L per pot) to avoid excessive accumulation of salts.
Collection of plant material for the determination of salicylic acid content, catalase activity and total antioxidant capacity
A single plant was one experimental unit, and the third completely developed leaf starting from the apex was used as a sample for evaluation. Plants that had already been sampled once were not used for subsequent evaluations. For each variable, treatment and sampling time, the leaves of two different plants (biological replicates) chosen at random were collected.
Leaf material used for the determination of salicylic acid and catalase activity was collected prior to application of treatments and at 12, 24, 48, 144 and 240 h after SA application. Leaf samples used for measuring total antioxidant capacity were collected at 48 and 144 h after SA application.
Prior to SA determination, cut leaves were wrapped in aluminum foil and were immediately submerged in liquid nitrogen for subsequent storage at -80°C. Samples used for verifying catalase activity and total antioxidant capacity were placed in a cooler at 4ºC and in liquid nitrogen, respectively, and were taken to the laboratory to be processed immediately.
Determination of SA
SA determination was performed using the modified method of Forcat et al. (2008), which involves (1) preparation of the sample for extraction and (2) determination of the SA concentration in the obtained extract. For (1), frozen plant material was ground in a mortar using liquid nitrogen. Ground material (0.05 g) was subsequently placed in a 1.5 ml microcentrifuge tube and 1 ml of extraction solution (10% methanol, 1% acetic acid, 89% distilled water) was added. The sample was subsequently vortexed for 20 s in a vortex mixer (Scientific products), degassed for 5 min in a Bramson gas stripper (model 1510) and centrifuged in a microcentrifuge (Labnet International, model 24D) for 10 min at 10,000 x g (10,400 rpm). After this procedure, the translucent supernatant was extracted with a manual pipette and placed into a fresh microcentrifuge tube. The sample was re-extracted thereafter with 0.5 ml of the extraction solution; thus, approximately 1.5 ml of solution containing the compound of interest was obtained. This solution was filtered through a 0.45-µm syringe-driven filter unit (Millipore), and the SA content was determined with an Agilent 1120 liquid chromatograph (LC) using the EZChrom Elite Compact software version 3.3.0B (Agilent 2005-2008). An aliquot (20 µl) was injected into the LC, and the separation was performed using a Phenomenex Luna C18 column (100 × 20 mm) at 35°C using two solvents: A (94.9% H2O: 5% CH3CN: 0.1% HCOOH) and B (5% H2O: 94.9% CH3CN: 0.1% CHOOH). A gradient from 0 to 100% B was run over 10 min. Each leaf sample was analyzed twice. The results obtained from the chromatograph (in mg L-1 of SA) were converted to µg of SA per g fresh weight.
Determination of CAT enzymatic activity
Determination of CAT activity was performed on the same day that leaf samples were collected using the technique described by Masia (1998). Using an AHUS Pioneer analytical balance, 0.5 g of leaf tissue was weighed and subsequently macerated in a mortar prior to the addition of 50 mg of polyvinylpyrrolidone (Sigma-Aldrich) and 5 ml of phosphate buffer solution (100 mM, pH 7.0). The sample was aliquoted into microcentrifuge tubes and centrifuged in a microcentrifuge at 11,000 x g (11,440 rpm) for 11 min. The supernatant was diluted 1:10 with distilled water. From the resulting solution, 1 ml was removed, mixed with 3 ml of 300 μM phosphate buffer and added to 1 ml of H2O2 (100 µM). The reaction between CAT and H2O2 was terminated after one minute by applying 10 ml of 2% sulfuric acid. This was then titrated with 0.01 N potassium permanganate. Using the same procedure, a blank was produced with distilled water. Catalase activity was determined with the following formula:
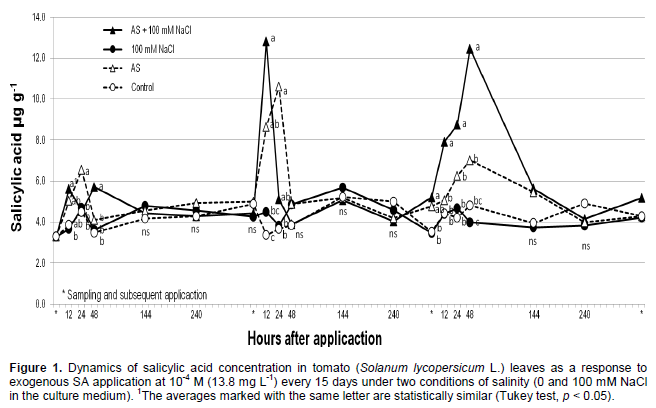
where TV = volume of KMnO4 consumed in the blank (ml); MV = volume of KMnO4 consumed in the sample (ml); KMnO4 concentration is given in moles; sample = weight of tissue used; NaKPi = sodium phosphate buffer (5 ml); FD sample = final sample dilution; final NaKPi = final amount of sodium phosphate buffer used (8 ml). One unit of catalase was defined as the amount of enzyme required to decompose 1 µM of H2O2 per min per g of tissue (Masia, 1998).
Determination of TAC
Determination of TAC was performed on the same day that leaf samples were collected using the technique described by Ramírez et al. (2006). The leaves were frozen in liquid nitrogen and ground in a mortar, and 4 g of the sample was added to 8 ml of 100 mM phosphate buffer (pH 7.0). Next, the sample was vortexed for 2 min using a vortex mixer (Scientific products) and centrifuged at 2880 x g (3000 rpm) for 10 min. The Calbiochem® Total Antioxidant Status Assay Kit (Miller et al., 1993) was used for determination of total antioxidant capacity. Briefly, an aliquot (20 µl) of the supernatant (extract) was combined with 1 ml of the chromogen (metmyoglobin + ABTSTM), and the absorbance of the resulting solution was determined at a wavelength of 600 nm using a spectrophotometer (Jenway, model 6320D). Absorbance values were also determined for a blank (distilled water) and a standard (Trolox: 6-hydroxy-2, 5, 7, 8-tetramethyl-chroman-2-carboxylic acid, 2.03 mM). After 3 min, 200 µl of the substrate (stabilized hydrogen peroxide) was added to the experimental, blank and standard samples, and the absorbance at 600 nm was read again to obtain differential (∆) measurements (∆ = initial absorbance – absorbance after 3 min). The total antioxidant capacity equivalent to Trolox (mM) was determined using the following formula:

Determination of fruit production
Fruit from ten plants exposed to each treatment were collected for five harvests, which determined the total amount of fruit produced between 73 and 105 days after transplant (in g plant-1). The fruit were weighed with a digital analytical balance (Explorer Pro).
Experimental design and statistical analysis
The experiment was performed using a randomized complete block design with two replicates. A single plant was the experimental unit, and two plants per assessment per treatment were considered. A total of 50 plants were included in each treatment.
A Wilcoxon matched-pairs test (Statistica for Windows, version 6.0) was used to ascertain differences between the concentrations over time of different compounds into the leaves. Similarly, a cross-correlation function test (Statistica for Windows, version 6.0) was applied to the concentration data to determine possible correlations between the levels of SA and CAT. The results from each sampling period were compared using an analysis of variance and a multiple comparison Tukey test to compare means (p ≤ 0.05) (Statistical Analysis System for Windows, version 9.0).
Change in SA concentration in leaves
The Wilcoxon test indicated a statistically significant difference (p ≤ 0.05) between the treatments. The exogenous application of SA to leaves resulted in the highest concentrations of this compound into the leaves (Figure 1) and the salinity condition (100 mM NaCl) did not alter the SA levels into the leaves. Treatments without SA resulted in the lowest SA concentrations into the leaves, and again the salinity condition (100 mM NaCl) did not have any effect. Similar results were reported by Szalai and Janda (2009) who applied 50 and 100 mM NaCl to corn seedlings (Zea mays L.) and observed no increase in the concentration of leaf SA. Additionally, Hussain et al. (2011) applied 50 mM NaCl to violets (Viola odorata L.) without observing an increase in SA concentration in leaves, and Iqbal and Ahsraf (2006) did not observe a significant change in SA concentration in wheat seeds (Triticum aestivum L.) that were germinated in a culture medium containing 150 mM NaCl (electrical conductivity of 15 dS m-1). It is possible that the detection of significant changes in SA concentration depends on (1) the timing of sampling and the NaCl concentration applied and (2) the specific stage of plant development and the species under study.
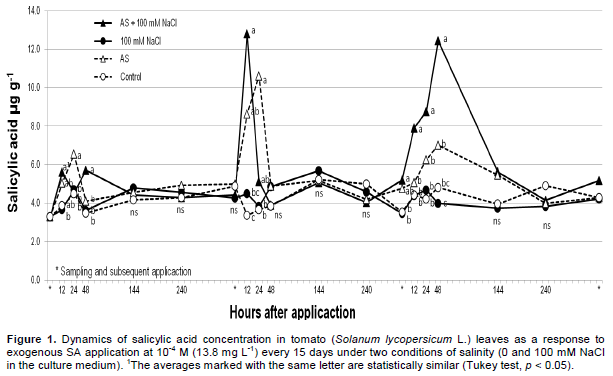
When comparing different sampling times (Figure 1), where was sprayed SA to plants without salinity conditions the SA concentration in leaf tissue was highest at 24 h after the first SA application, at 12 and 24 h after the second application and at 12, 24, and 48 h after the third application (Figure 1). The SA concentration subsequently decreased and was approximately equal to the control plants at 48 h after the first and second applications and 144 h after the third application. Although SA treatments resulted in similar increases in leaf SA levels in all sampling events regardless of the concentration of NaCl applied, we observed significant differences (p ≤ 0.05) between the treatments at 24 h after the first application and at 12, 24, and 48 h after the third application, with the highest SA values being observed in cases where NaCl was added (Figure 1). A similar response was described by Esmailzadeth et al. (2008), who applied SA (400 µM) to tomato leaves infected by Alternaria alternata and observed the lowest SA values when SA and the pathogen were applied separately. The significant increase in SA concentration in saline-stressed leaves upon foliar SA application (SA on the leaves + 100 mM NaCl in the substrate) could be attributed to the known function of SA as a stress signaling compound and to the induction of a resistance response (Iwai et al., 2007). Plants exposed to the control treatment did not show significant variation in leaf SA concentration throughout the study.
Similar to what was observed in our experiments, an increase and subsequent decrease in SA levels was reported by He et al. (2007) in bean leaves (Phaseolus vulgaris L.) when adding the mite Tetranichus urticae and by Ogawa et al. (2010) in Arabidopsis thaliana leaves when applying mechanical stimuli. These authors also observed peak SA concentrations 24 h after the stimulus was applied with a subsequent decrease in concentration. This decrease in detectable SA (after a concentration peak) after all applications may be due to the transformation of SA into conjugated forms (Klämbt, 1962), which would mainly include salicylic and benzoic glucosides (Mustafa et al., 2009; Owaga et al., 2010), methylated forms or forms activated with coenzyme A.
The concentrations of foliar SA determined in the present study are similar to those reported by He et al. (2007) in bean leaves 24 h after infestation with T. urticae mites, with a maximum SA value of 12 µg g-1 of fresh weight. Shulaev et al. (1995) observed maximum SA concentrations equivalent to 10 µg g-1 fresh weight in tobacco plants (Nicotiana tabacum L.) infected with tobacco mosaic virus (TMV) with an increase in SA concentration in tissue closest to the infection site 144 h after the infection began. Moreover, Iwai et al. (2007) determined an SA concentration of 7.3 µg g-1 fresh weight when applying probenazole to rice plants (Oryza sativa L.). Endogenous concentration of SA may depend on the plant species in question, the concentration of applied SA, and the conditions under which the trials are performed, although the apparent transformation of a fraction of the free SA into conjugated forms is consistent even if a high concentration of SA is applied and/or measurements are obtained as early as 30 min (Norman et al., 2004). Even at the lowest concentrations, peaks and declines are observed (Figure 1) which leads to the assumption that SA is incorporated into conjugated molecules, as observed by Yalpani et al. (1991), who reported SA concentrations of 0.35 µg g-1 dry weight in tobacco leaves (Nicotiana tabacum L.) 144 h after infecting plants with TMV.
CAT activity
The Wilcoxon test indicated a statistically significant difference (p ≤ 0.05) in CAT activity among the different treatments. The two treatments in which NaCl was applied showed the highest CAT activity, and this activity was not affected by SA application. The two treatments in which NaCl was omitted induced a lower level of CAT activity than the ones above and did not differ from each other, which indicates that compared with SA; NaCl has a greater effect on CAT. Furthermore, when comparing the different sampling times (Figure 1), the highest CAT activity was consistently observed following treatment with both NaCl and SA (Figure 2). This apparent synergistic effect of NaCl and SA on CAT activity agrees with studies using maize (El-Khallal et al., 2009), and Vicia faba (Azooz, 2009). It is possible that the effect of foliar SA on CAT activity is related to the interaction between SA and reactive oxygen species (ROS) formed during oxidative stress induced by NaCl (Miller et al., 2010). To verify this finding, a cross-correlation between two time series was performed, which yielded a significant positive correlation (R=0.571) between the dynamics of foliar SA concentration and CAT activity in the treatment with NaCl and SA. The correlation was not significant (p > 0.05) for any of the other treatments. This result may indicate that the interaction of SA and NaCl modifies the redox balance by stimulating the formation of ROS and H2O2, which, in turn, stimulates the accumulation of antioxidant enzymes, such as CAT (Miller et al., 2010).
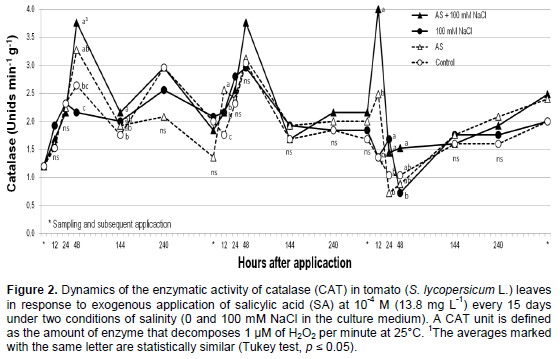
The changes in CAT activity following foliar SA applica-tion maybe were related to increases in the concentration of free radicals or compounds derived from them, such as H2O2 (Srivalli et al., 2003). Furthermore, Feieraband and Dehne (1996) indicated that the decreases following peaks of maximum CAT activity may result from the presence of a greater number of antioxidant molecules, such as peroxidase ascorbate, as was observed in corn by El-Khallal et al. (2009). Alternatively, the activity of proteases that degrade CAT may be induced by high levels of (or long periods of exposure to) a particular stress factor (Noreen and Ashraf, 2009).
Total antioxidant capacity (TAC)
The Wilcoxon test indicated statistically significant differences (p ≤ 0.05) between the treatments. Foliar application of SA induced the highest level of TAC in one sample at 144 h after application, while the application of NaCl did not have any effects. This response is attributed to the capacity of SA to modify the cellular redox balance by activating certain responses associated with oxidative stress, which, in turn, trigger the accumulation of antioxidant compounds (Alvarez, 2000).
The TAC of the foliar extract obtained at the different sampling times was higher at 144 h after first application in the SA application with or without salinity condition, and at 48 h after third application (Figure 3). We have no explanation about the increase of TAC in control treatment at 144 h after the second and third application, maybe has relation with an increase of midday maximum temperature into the greenhouse from 30 to 34°C, which could give rise to changes in the concentration of antioxidants (Sairam et al., 2000; Almeselmani et al., 2006).
Fruit production
Fruit production from the five cuts was not significantly different (p > 0.05) between plants exposed to the control treatment and those treated with foliar SA applications (Figure 4). Other authors reported increases in fruit yield (Yildirim and Dursan, 2008) after SA application. However, SA induces a wide range of metabolic responses that are generally directed toward adjusting the redox balance in the photosynthetic machinery under conditions of environmental stress. In the case of stress induced by salinity, adjustments are primarily made in the levels of antioxidant compounds that alleviate oxidative stress, and these adjustments do not always result in an increase in fruit yield (Joseph et al., 2010).
Foliar application of salicylic acid to tomato plants showed a residual effect in the concentration of this compound in leaves that lasted up to 48 h after application. The effect was evident in plants with and without NaCl treatment. According to the results of this assay, SA applications on tomato should be performed within a minimum interval of six to 10 days in order to maintain the SA concentration higher than the basal ones. The addition of 100 mM NaCl to the nutritive solution did not modify the catalase activity, the total antioxidant capacity, salicylic acid concentration in leaves or fruit production. This trend was modified by the application of salicylic acid, which in combination with NaCl application increased the salicylic acid concentration, catalase activity and the total antioxidant capacity on leaf tissue but did not modify fruit production. The application of salicylic acid over plants in absence of NaCl stress increased the catalase activity and the total antioxidant capacity of foliar tissue without modifying fruit production.
The authors declare that there is no conflict of interests regarding the publication of this paper.
Author Guzmán-Téllez thanks CONACYT for the financial support.
REFERENCES
Almeselmani M, Deshmukh P, Sairam R, Kushwaha S, Sing T (2006). Protective roll of antioxidant enzymes under high temperature stress. Plant Sci. 171:382-388.
Crossref |
|
Alvarez M (2000). Salicylic acid in the machinery of hypersensitive cell death and disease resistance. Plant Mol. Biol. 44:429-442.
Crossref |
|
Ashraf M, Akram NA, Arteca RN, Foolad MR (2010). The physiological, biochemical and molecular roles of brassinosteroids and salicylic acid in plant processes and salt tolerance. Crit. Rev. Plant Sci. 29:162-190.
Crossref |
|
Azooz M (2009). Salt stress mitigation by seed priming with salicylic acid in two faba bean genotypes differing in salt tolerance. Int. J. Agric. Biol. 11:343-350. |
|
Azooz M, Youseef M (2010). Evaluation of heat shock and salicylic acid treatments as inducers of drought stress tolerance in wheat. Am. J. Plant Physiol. 5:56-70.
Crossref |
|
El-Khallal S, Hathout T, Ahsour A, Kerrit A (2009). Brassinolide and salicylic acid induced antioxidant enzymes, hormonal balance and protein profile of maize plants grown under salt stress. Res. J. Agric. Biol. Sci. 5:391-402. |
|
Esmailzadeth M, Soleimani M, Rouhani H (2008). Exogenous application of salicylic acid for induced systemic acquired resistance against tomato steam canker disease. J. Biol. Sci. 8:1039-1044.
Crossref |
|
Feieraband J, Dehne S (1996). Fate of the prophyrin cofactors during the light-dependent turnover of catalase and the photosystem it reaction-center protein DI mature rye leaves. Planta 198:413-422.
Crossref |
|
Forcat S, Bennett N, Mansfield J, Grand M (2008). A rapid and robust method for simultaneously measuring change in the phytohormones ABA, JA y SA in plants following biotic and abiotic stress. Plant Methods 4:16.
Crossref |
|
He W, Li H, Li X, Li M, Chen Y (2007). Tetranynchus urticae Koch induced accumulation of salicylic acid in frijole leaves. Pesticide Biochem. Physiol. 88:78-81.
Crossref |
|
Hussain M, Nawaz K, Majeed A, Ilyas U, Lin F, Ali K, Nisar MF (2011). Role of exogenous salicylic acid applications for salt tolerance in violet. Sarhad J. Agric. 27:151-175. |
|
Iqbal M, Ahsraf M (2006). Wheat seed priming in relation to salt tolerance: growth, yield and levels of free salicylic acid and polyamides. Ann. Bot. Fennici 43:250-259. |
|
Iwai T, Seo S, Mitsuhara I, Ohashi K (2007). Probenazole induced accumulation of salicylic acid confers resistance to Magnaporte griseain adults plants rice. Plant Cell Physiol. 48:915-924.
Crossref |
|
Joseph B, Jini D, Sujatha S (2010). Insight into role of exogenous salicylic acid on plants growth under salt environment. Asian J. Crop Sci. 2:226-235.
Crossref |
|
Klämbt H (1962). Conversion in plants of benzoic acid to salicylic acid and its βd-glucoside. Nature 196:491.
Crossref |
|
Masia A (1998). Superoxide dismutase and catalase activities in apple fruit during ripening and postharvest and with special reference to ethylene. Physiol. Plant 104:668-672.
Crossref |
|
Miller N, Rice-Evans C, Davies M, Gopinathan V, Milner A (1993). A novel method for measuring antioxidant capacity and its application to monitory the antioxidant status in premature neonates. Clinical Sci. 84:407-412.
Crossref |
|
Miller G, Suzuki N, Ciftci-Yilmaz S, Mittler R (2010). Reactive oxygen species homeostasis and signaling during drought and salinity stress. Plant Cell Environ. 33:453-467.
Crossref |
|
Mustafa N, Kin H, Choi Y, Vepoorte R (2009). Metabolic changes in salicylic acid-elicited Catharanthus roseus cell suspension cultures monitored by NMR-based metabolic. Biotechnol. Lett. 31:1967-1974.
Crossref |
|
Noreen S, Ashraf M (2009). Assessment of variation in antioxidative defense system in salt treated pea (Pisum sativum) and its putative use as salinity tolerant markets. J. Plant Physiol. 166:1764-1774.
Crossref
|
|
Norman C, Howell K, Millar A, Whelan J, Day D (2004). Salicylic acid is an uncoupler and inhibitor of mitochondrial electron transport. Plant Physiol. 34:492-501.
Crossref |
|
Ogawa T, Ara T, Aoki K, Suzuki H, Shibata D (2010). Transient increase in salicylic acid and its glucose conjugates after wounding in Arabidopsis leaves. Plant Biotechnol. 27:205-209.
Crossref |
|
Poór P, Gemes K, Horváth F, Szepesi A, Simon ML, Tari I (2011). Salicylic acid treatment via the rooting medium interferes with stomatal response, CO2 fixation rate and carbohydrate metabolism in tomato, and decreases harmful effects of subsequent salt stress. Plant Biol. 13:105-114.
Crossref |
|
Ramírez H, Ranca-o-Arrioja JH, Benavides-Mendoza A, Mendoza-Villareal R, Padron-Corral E (2006). Influencia de promotores de oxidación controlada en hortalizas y su relación con antioxidantes. Revista Chapingo Serie Horticultura 12:189-195. |
|
Sairam R, Srivastava, G, Saxena D (2000). Increased antioxidant capacity under elevated temperatures: a mechanism of heat stress tolerance in wheat genotypes. Biol. Plant. 43:245-251. |
|
Shulaev V, León J, Raskin I (1995). Is salicylic acid a translocated signal of systemic acquired resistance in Tobacco? Plant Cell 7:1691-1701. |
|
Srivalli B, Sharma G, Khanna-Chopra R (2003). Antioxidative defense systems in an upland rice cultivar subjected to increasing intensity of water stress followed by recovery. Physiol. Plant. 119:503-512.
Crossref |
|
Steiner B (1961). A universal method for preparing nutrient solution of a certain desired composition. Plant Soil 16:134-154.
Crossref |
|
Szalai G, Janda T (2009). Effect of salt stress on the salicylic acid synthesis in young maize (Zea mays L.) plants. J. Agron. Crop Sci. 193:165-171.
Crossref |
|
Yalpani N, Silverman P, Wilson T, Kleier D, Raskin I (1991). Salicylic acid is a systemic signal of pathogenesis related protein in virus infected tobacco. Plant Cell 3:809-818. |
|
Yildirim E, Dursan E (2008). Effect of foliar salicylic acid applications on plant growth and yield of tomato under greenhouse condition. Acta Hortic. 807:395-400. |
|
Zhang Z, Li Q, Li Z, Staswick P, Wang M, Zhu Y, He Z (2009). Dual regulation role of GH3.5 in salicylic acid and auxin during Arabidopsis-Pseudomonas syringae interaction. Plant Physiol. 145:450-464.
Crossref |