ABSTRACT
Soil organic carbon is a fundamental soil resource base. However, there is limited information on soil organic carbon storage due to influence of tillage type and soil texture under smallholder production systems in Ethiopia. The objective of this study was therefore to quantify soil organic carbon in different soil textures and tillage types; and to the contribution of livestock in improving soil carbon, soil structure and soil fertility. Fifteen sample sites were selected for soil chemical analysis details on crops, soil and land management practices for each sample site was collected through household interviews, key informants discussion and literature review. The carbon storage per hectare for the four soil textures at 0 to 15 cm depth were 68.4, 63.7, 38.1 and 31.3 t/ha for sandy loam, silt loam, loam and clay loam; respectively. Sand and silt loams had nearly twice the organic carbon content than loam and clay loam soil. The soil organic carbon content for tillage type at 0 to 15 cm was 8.6, 10.6, 11.8 and 19.8 g kg-1for deep tillage, minimum tillage, shallow tillage, and zero tillage; respectively. Among tillage types soil organic carbon storage could be increased by using the minimum and shallow tillage. Carbon saved due to shallow cultivation as practiced by Ethiopian smallholders using oxen drawn plough contributed to carbon trade off of about 140 million ton per year. At current levels of carbon saving shallow tillage would generate $4.2 billion of revenue per year for Ethiopian smallholders.
Key words: Carbon budget, crop residue, manure, smallholder farming, soil texture, tillage.
Soil carbon storage is defined as “the process of transferring carbon dioxide from the atmosphere into the soil through crop residues and other organic solids, and in a form that is not immediately reemitted”. This transfer or “storage” of carbon helps to off-set emissions from fossil fuel combustion and other carbon-emitting activities, while enhancing soil quality and long term agronomic productivity (Sundermeier et al., 2005). Under mixed crop and livestock production systems in Ethiopia, crop production is the major cash income earner (IPMS, 2004), while livestock production plays an important role as a source of draught power for crop production, organic fertilizer, for crop production as well as serve as a living bank; and for household income generation and for human nutrition (Hadera, 2001).
Although livestock production was associated with environmental degradation and ecological devastation and irreversible desertification (FAO, 2006); there is an ample evidence to show that livestock production contributed positively to environmental stability in terms of carbon balance into the soil. Under smallholders management, the positive aspects of livestock production contribution to carbon storage is attained when farmers use animal power for farm operations such as ploughing, disking, ridging, weeding, threshing and transporting agricultural inputs and outputs; which is a carbon zero operation compared with mechanized agriculture (de Hann et al., 1998). The addition of animal manure and livestock waste into the soil is an alternative management option as carbon input for soil carbon storage (FAO, 2001; Lal, 2002, 2004).
Soil texture plays an important role in carbon storage in the soil and strongly influences nutrient availability and retention. Whendee et al. (2000) reported that sandy soils stored approximately 113 t/ha carbon to 100 cm depth versus 101 t/ha carbon in clay soils in a forest ecosystem in the Amazon. Fine-textured soils prevent organic matter against decomposition by stabilizing with minerals and clay surfaces; and physical protection of organic matter within aggregates (FAO, 2004).Carbon and nitrogen mineralization rates are often lower in clay soils than in coarse-textured soils because of the holding capacity of organic molecules onto surface minerals, which seems to be a major mechanism of soil organic-matter preservation (Christensen, 2001). Soils with less than the protective capacity are potentially capable of sequestering soil organic carbon in their clay and silt fractions (FAO, 2004).
Soil organic carbon content of cropping soils is well below the potential protective capacity, because they have been subjected to conventional tillage and burning practices, which cause substantial carbon losses (Lal, 2004). Therefore, tillage types have important impacts on soil organic carbon including controlling residue placement in soils (FAO, 2004). Deep tillage buries crop residue, but the residue is not mixed uniformly throughout the tillage depth (Lal, 2004). Moreover, tillage brings subsoil to the surface where it is exposed to atmospheric cycles (Lemus and Lal, 2005) and increases the decomposition of soil organic carbon. However, zero tillage provides minimum soil disturbance and promotes soil aggregation through enhanced binding of soil particles (FAO, 2004).
Depletion of soil organic carbon stocks has created a soil carbon deficit that represents an opportunity to store carbon in the soil through a variety of land management approaches (Franzluebbers et al., 2000). Increase in soil organic carbon can be achieved by increasing carbon inputs and decreasing the decomposition of soil organic matter or both (Rosenberg et al., 1999). Sundermeir et al. (2005) observed that the rate of organic carbon sequestration in the soil increase by each ton of residue applied was more for zero tillage than for plough-tilled cultivation. Therefore, it is important to study the impact of different land tillage practices under mixed crop and livestock production systems on carbon storage as well as the contribution of livestock to carbon storage through shallow tillage.
Previous research studies on carbon storage were related to natural resource management, forestry and soil perspective (Lal, 2004), whereas the current study was related to the contribution of livestock to improving soil organic carbon, soil structure and soil fertility; and the role of animal draught power in decreasing soil organic carbon decomposition due to tillage.
In this article, the relationships of soil organic carbon with both soil texture and tillage types was evaluated and discussed. The hypothesis that soil carbon content depends on the soil texture and tillage types was also tested. We also assessed the contribution of livestock and crop residues in improving soil structure, soil organic carbon and fertility. The investigation was based on soil samples collected in 2009 for chemical analysis as well as in the same year land; livestock and a crop management history of the study sites were captured through interviews with farmers and key informants who had a good knowledge of the study area.
Study area
The study was conducted in central Ethiopian highlands that represent 90%of the farming system in Ethiopia. In the highlands, the main agricultural activity is smallholder mixed farming systems dominated by crop production and livestock keeping (Constable, 1984), livestock play a key role in crop farming in ploughing the soil, threshing, transporting and adding manure to the soil.
The highland further subdivided into three zones based on the development potential and resource base at: the high potential cereal/livestock zones, the low potential cereal/livestock zone; and high potential perennial crop/livestock zone (Amare, 1980; FAO, 1988).
The current study area falls under the high potential cereal/livestock production zone (IPMS, 2004), which is located southeast of Addis Ababaat latitude 8°46’ 16.20’’ to 8°59’ 16.38’’ N and longitude 38°51’ 43.63’’ to 39°04’ 58.59’’ E, on the western margin of the Great East African Rift Valley. The altitude of the area ranges from 1500m to 2000m above sea level. Two major agro-climatic zones were identified in the study area: the mountain zone >2000meter above sea level, which covers 150km2or 9% of the area; and the highland zone at 1500 to 2000 m above sea level, which covers >1600 km2or 91% of the area (IPMS, 2004).
The agro-ecology of the study area is best suited for diverse agricultural production systems. The area is known for its excellent quality Teff grain, which is an important staple food grain in Ethiopia that is used for making bread (Enjera). Wheat is the second most abundant crop, while pulses especially chickpeas grow in the bottom lands and flood basins. Livestock production is an integral part of the whole agricultural production system.
Study methods
The study was designed to quantify soil organic carbon in different soil textures and tillage types and to determine the contribution of livestock in improving soil carbon, soil structure and soil fertility. The study combined socioeconomic surveys with laboratory chemical analyses. The laboratory data were complemented by land management histories and the current land management practices. Sample sites were selected purposely in consultation with farmers, experts and reviewing of secondary data. The selected sample sites had 15 characteristics, which were further subdivided into soil textures and tillage types to estimate carbon stock. Sample sites were described as shown in Table 1 based on world overview of conservation approaches and technology categorization system for conservation measures and land use management (WOCAT, 2013). The soil textures were subdivided into four classes based on the AOAC (2000) and Asnakew (1988) as loam, sandy loam, silt loam and clay loam.
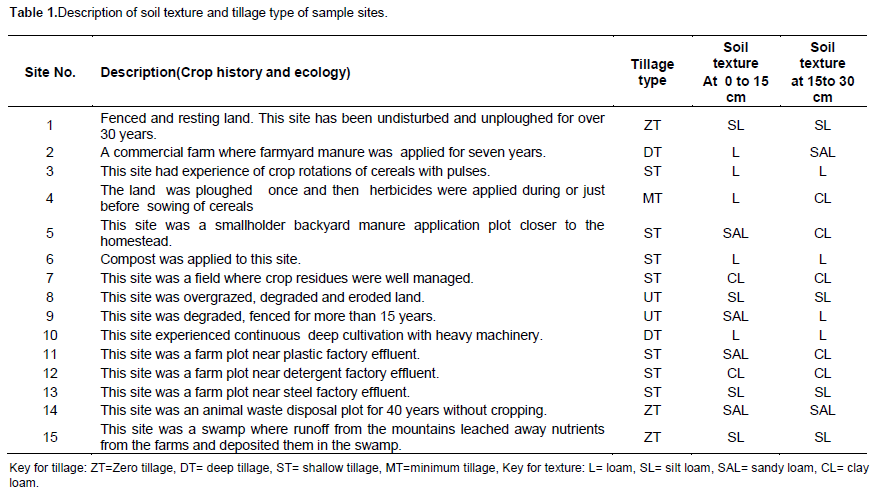
The tillage types were classified as described by Abiye and Firewe (1993) as follows: deep tillage uses mechanized tractors with disc ploughs at a soil depth 30 cm and above. Minimum tillage involves the removal of weeds first by using herbicides followed by ploughing only once by oxen at the depth of 8 to 10 cm before sowing. Shallow tillage uses a traditional ox-drawn plow at the depth of 15 to 20 cm. Zero tillage is where an area has never been ploughed for a long period of time.
Soil sampling and analysis
In June 2009, soil samples were taken from the above 15 sites samples were collected to determine the impacts of different land management practices and conservation measures such as slopes, degradation level, crop history, tillage type, level of fertilization, residue management and compost or manure application. Crops, soil and land management practices for each site were described based on detailed interviews with farmers, key informants and literature review. At each site, the auger was used to take samples from the top soil at 0 to15 cm and 15 to 30 cm soil depths at four points spaced within 30 to 50 m intervals through a transect walk. Soil extracted at these points was pooled to make up a single sample for analysis and mineralization assays. Prior to the soil sample collection, coarse organic matter (leaves, wood, and roots) was removed by hand. The samples were mixed and homogenized, and the pooled sample was stored in a plastic bag and transported to Debrezeit research centre soil laboratory within two hours of collection. Immediately after the arrival, soil samples were air dried ground with mortar and pestle, and sieved through a 2mm sieve. Soil organic carbon content was determined by using the procedure described by Walkely and Black (1934). Particle size analysis was carried out as described by Bouyoucos (1951).
Soil moisture analysis was done by oven drying of 15 samples for 24 hat 105°C. Samples were weighed and the amount of carbon per unit area was calculated using the formula described by Pearson et al. (2005) as: C (t/ha) = [(soil bulk density, (g/cm3) x soil depth (cm) x % c)] x 100. The carbon content was expressed as a decimal fraction that is, 2.2%C was expressed as 0.022. Soil bulk density was estimated by using the Adams equation (Adams, 1973) as shown below:
Where; BD = bulk density, OM = organic matter, MBD= mineral bulk density A typical value of 1.64 was used for MBD (Mann, 1986).
Statistical analysis
Detailed statistical analysis of the data was carried out by SPSS Version 17.0.1 (2008). A post hoc multiple comparison tests of means were done by univariate least significant difference.
Effect of soil texture on soil organic carbon content
Haque et al. (1993) noted that chemical properties of vertisol type of Ethiopia showed low organic matter and total nitrogen, while Ali (1992) observed that at 0 to 25 cm depth soil organic carbon content was 18.6 g kg-1in a similar site in the present study area. In the current study the average soil organic carbon content across all soil textures was 14.0 g kg-1in 0 to 15 cm and 10.0 g kg-1at 15 to 30 cm, which were well below the value reported by Ali (1992). The reason for these differences could be due to the effect of human anthropogenic factors such continuous ploughing, removal of crop residue, and change in traditional practices (such as use of manure for energy source than for soil fertilization) for the last 30 years. The soil textures in the study area were categorized into four groups, namely: clay loam (27%), loam (40%), sandy loam (20%) and silt loam (13%). At P-value<0.05, there was a significant difference in soil organic carbon content between these soil texture groups at 0 to 15 cm soil depth which is probably attributed due to organic inputs and land use history, but there was no significant difference at 15 to 30 cm depth (Table 2). Post and Kwon (2000) reported that the amount of decomposition rate and placement of carbon inputs above and below ground differed between ecosystems, soil texture and land uses.

Sandy Loam had the highest carbon content at both soil depths, estimated to be 28.1±9.5 6 g kg-1 for 0 to 15 cm soil depth and 15.5±5.6 g kg-1 for 15 to 30 cm soil depth. Mengistu and Fassil (2010) reported that in Northern Ethiopia the total organic carbon (TOC) ranged from 7.3 to 17.4 g kg-1. The highest 17.4 g kg-1 was found at the depth of 0 to 15cm in the forestland and the lowest 7.3 g kg-1 was observed in the farmland. In the current study the average carbon content ranged from 6.6 ± to 28.1±9.5 6 g kg-1 which was a wide range of variation because of differences in agriculture management practices.
From the mean results in Table 3, at the depth of 15 to 30 cm sandy loam texture had 15.1 ±5.6 g kg-1 carbon content and silt loam had 14.0±7.5 g kg-1. Both textures maintained better carbon contents than loam and clay loam which had soil organic carbon of 8.1±4.4 g kg-1 and 6.6±3.3 g kg-1; respectively. Organic carbon content was higher at 0 to 15 cm depth than at 15 to 30 cm depth for all soil texture types (Table 3). The result indicated that sandy loam and silt loam had twice more soil organic carbon content than loam and clay loam. Similarly, Tilahun and Asefa (2009) reported that in south east Ethiopia the soil dominated with clay in texture was negatively correlated with SOC stocks.
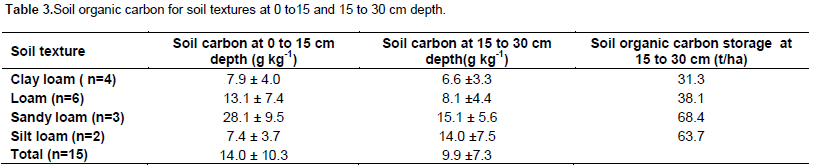
This implied that porosity and particle size had an effect on soil organic carbon accumulation (Whendee et al., 2000; Lal, 2004). However, Christensen (2001) and Milne and Heimsath (2012) suggested that soils with high clay content tend to have higher soil organic carbon than soils with low clay content under similar land use and climate conditions. In the current study the variation could be accredited mainly due to differences in land use type and crop history.
In the current study the carbon storage was calculated per hectare based on the average soil organic carbon percentage. The carbon storage for the four soil textures at 15 to 30 cm soil depth were 68.4, 63.7, 38.1 and 31.3 t/ha for sandy loam, silt loam, loam and clay loam, respectively, as shown in Table 3.
From the data of carbon storage in Table 3 the difference between sandy loam (68.4 t/ha) and that of clay loam (31.3 t/ha) was 36.7 t/ha. Using FAO(2001) plotting unit of carbon to wheat yield a difference of 36.7 t/ha for sandy loam would enable to produce 734 to 1468 kg more wheat per hectare than would have been produced by clay loam under the assumption of similar weather and management condition. In order to translate the impact of increase in crop yield because of carbon storage and subsequent repercussion on livestock it is useful to mention the use of crop residues as animal feed. To estimate the amount of crop residues produced from increased storage, the use of extrapolation of grain to straw ratio is important. In a study conducted in Ethiopian highlands wheat grain to straw ratio 1:1.8 and positively correlated (Kahsay, 2004). That meant the increase in grain productivity consequently results in an increase in straw production, which eventually add value to livestock feed.
The study area was dominated by Vertisols (IPMS, 2004; Kahsay, 2004), and this soil type is characterized with low organic carbon content and poor response to chemical fertilization (Haque et al., 1993; Srivastava et al., 1993). Thus under traditional soil management practices vertisols produce low crop yield. However, there was ample proof to show that this type of soils was capable of producing much more food and feed than they were producing, provided that they were adequately and properly managed (Mesfin and Jutzi, 1993).
Abate et al. (1993) reported that with proper drainage of vertisols using oxen drawn broad bed makers, cereal grain yield increased by 106% and that of straw increased by 78% compared with traditional hand used drainage systems. The effect of improved drainage on fertilizer use efficiency of cereal was investigated by Abate et al. (1993) who found that grain and straw yield were increased by 30%. They also noted that different crops and soil husbandry practices increased grain and straw yield; for example, sequential cropping increased by 60%; mixed cropping of legumes and cereals raised by 40%; row intercropping of forage legumes with cereals boosted by 30%; and forage grass and legume mixed cropping improved by 40%. All this resulted in high biomass production of both grain and straw. These results showed that through proper soil drainage and agronomic management practices it is possible to increase soil organic content and land productivity.
In smallholders farming livestock contributed to soil drainage by drawing broad bed markers for better aeration, nutrient uptake and improve storages. Therefore, livestock had a role in generating power for the improvement of soil structure and fertility under smallholder mixed agriculture, too.
It should also be noted that nitrogen is the most limiting nutrient in vertisols. The use of forage and grain legumes enhances nitrogen fixation which leads to increased productivity of grain and straw (Desta, 1988; Haque, 1992). Hence, animal feed production can be integrated with soil structure and fertility improvement. Animal manure and other animal wastes increase soil organic carbon content, total nitrogen and water retention capacity (Jutzi et al., 1987) which increase both grain and straw storage, and meet livestock nutrient demand for better milk and meat production.
The contribution of livestock in soil organic carbon storage in tilled land
The depth of ploughing has a significant impact on carbon storage in the soil, where organic matter and minerals are mechanically mixed with the top layer (Lal, 2009). Sundermeier et al. (2005) reported that sub-soiling at 35.6 cm depth caused the loss of more carbon than ploughing at 20.3 cm depth. They also reported that sub-soiling caused the loss of more carbon than strip-tillage and zero tillage. In a 40-yearexperimentation in Ohio State University, Sundermeier et al. (2005) found that continuous zero tillage nearly doubled the organic matter content in the top 5 cm, while ploughing reduced it by a third. In another study conducted in Brazil, Barreto et al. (2009) found that soils under natural vegetation and conservation tillage systems generally had higher aggregation indices and total organic carbon stocks in the surface layer than soils under conventional tillage. It showed that zero tillage had an effect on carbon stabilization between the natural ecosystem and conventional tillage.
Hernanz et al. (2009) studied different tillage practices such as conventional tillage, minimum tillage and zero tillage in an experimentation which combined the rotation of cereals with pulses for 20 years. They found that the average soil organic carbon content was 14% higher in zero tillage than in minimum tillage and conventional tillage. They also reported that in zero tillage, stocked soil organic carbon content increased in the top layer but declined systematically in the bottom layer.
In the current study tillage types were categorized into four groups: deep tillage, minimum tillage, shallow tillage and zero tillage. The analysis of variance showed that there was no significant difference in carbon content between tillage types at both 0 to 15cm and 15 to 30 cm depth as shown in Table 4.
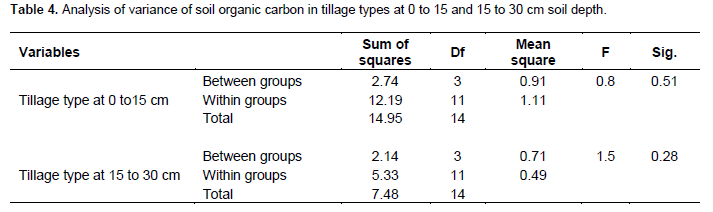
Soil organic carbon content of 0 to 15 cm depth was 8.6, 10.6, 11.8 and 19.8 g kg-1for deep tillage, minimum tillage, shallow tillage, and zero tillage; respectively. A similar trend was found at 15 to 30 cm depth for different tillage types as shown in Table 5. A land that had never been cultivated had soil carbon storage of 87.55 t/ha at 0 to 15 cm compared with 40.34 t/ha of deep tillage. That was twice the carbon storage capacity. Shallow tillage under traditional farming methods using oxen-drawn ploughs had carbon storage capacity of 54.28t/ha (62% of the zero tillage). Higher amount of soil organic carbon in zero tillage soils could have been occurred due to the protection of carbon from decomposition (Sundermeier et al., 2005). In contrast, conventional tillage causes oxidation of soil organic carbon because of the disruption of macro aggregates and exposure of soil organic carbon to microbial oxidation and decomposition (Hernanz et al., 2009).
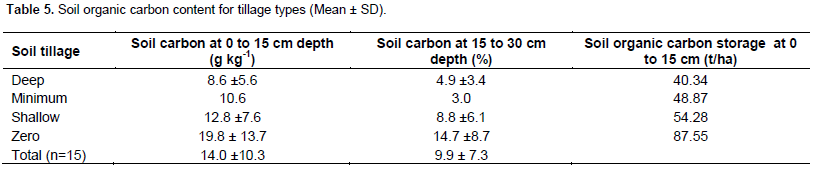
The results of the current findings were in agreement with the findings by Edwards et al (1992), who reported that after 10 years of experimentation comparing conventional mould board plough tillage with conservation tillage, the latter had increased surface carbon and nitrogen to 67% and the former had decreased by 66%. Jastrow (1996) also reported that the storage of soil organic carbon in shallow soil depth (<7.5 cm) was usually greater with zero tillage than with conventional tillage when sweep (<10 cm), chisel (15 cm) or mould board Plough (15 to 30 cm depth) were the primary tillage tools. Assuming a linear increase in soil organic carbon with time, Jastrow (1996) found that the rate of soil organic carbon storage at 0 to 50 cm depth in zero tillage was 84.44 g/m3C per year. He also reported that the carbon stock of continuously cropped and conventionally tilled soils was also 25% lower than the carbon stock in conservation tillage.
In the current study the amount of carbon saved by using shallow tillage with ox-driven plough instead of deep ploughing with mechanized tractors was estimated at 140 million tons of carbon per year, calculated based on the assumption that the difference of 54.28 for shallow tillage to 40.34 t/ha for deep tillage equals to 14 t/ha carbon saved due to tillage type and multiplied by 10 million hectares land annually ploughed by smallholder farmers using animal draught power in Ethiopia (MoFED, 2009). In addition to the carbon saving, shallow tillage fostered carbon-free non-fuel consuming draught power farm operations. For example, a wheat farm required about 5 tractor hours per hectare to complete all farm operations equivalent of 160 kg of carbon per hectare (FAO, 2004). When this figure was multiplied by 10 million hectares of land annually ploughed by smallholder farmers traditionally using draught power in Ethiopia, the total amount of carbon saved in Ethiopia due to tillage type estimated to be to 1.6 million tons of carbon per year.
One of the anticipated benefits for smallholder farming in carbon credit schemes was financial gain that could be achieved from carbon trading. Carbon credits create a market for reducing greenhouse emissions by giving a monetary value to the cost of polluting the air. Nordhaus (2008) has suggested that an optimal price of carbon is around $30/ton based on the social cost of carbon emissions and will need to increase with inflation. In general carbon saved due to shallow tillage contributed to carbon trade off of 140 million tons per year. At the current level of carbon saving and a tax of $30/t of carbon, shallow tillage could generate $4.2 billion revenue per year.
In addition to more carbon release into the atmosphere from the disturbed soil, mechanization breaks soil structure and contributes to wind and water erosion. Mechanization also releases carbon monoxide, carbon dioxide and other greenhouse gases from fuel combustion. Hence, shallow ploughing with draught power is a positive farm operation from an environmental point of view because it uses an energy source which is renewable and reasonably non-polluting. In addition, draught power (oxen, donkeys, horses, camels and mules) serves as a means of transport which is also relatively pollution free, unlike gasoline that releases too much carbon emissions. This indicated that livestock plays a key role in reducing carbon emissions to the atmosphere and global warming. However, it is not realistic to continue with traditional farming given the current increase in human population and the need for increased food production.
Loss of soil organic carbon can lead to a reduction in soil fertility, and an increase in land degradation and desertification when activities such as tillage type and land management practices were not properly applied. The natural soil texture characteristics in terms of carbon storage will would possibly changes as result of change in land use and crop management.
Livestock had a significant role in the improvement of soil structure and increased fertility through the provision of manure and draught power in smallholder mixed agriculture. It was noted that nitrogen was the most limiting nutrient in vertisols, while the use of forage and grain legumes enhanced nitrogen fixation which led to increased carbon absorption. Hence, animal feed production could be integrated to land care for improved soil structure and fertility. Animal manure and other animal wastes increased soil organic carbon. Livestock reduced carbon emissions when draught power was used for ploughing and transportation. As there are several evidence on the negative contribution of livestock towards global warming, there are also ample information where livestock can contribute positively towards environmental management and climate change through carbon storage reduction of carbon emission.
The authors have not declared any conflict of interests.
We gratefully acknowledge (ILRI) International Livestock Research Institute for the research fund support and use ILRI facilities.
REFERENCES
Abate T, Mohamed-Saleem MA, Tekalign M, Tirkessa D (1993). Grain, fodder and residue management. In: Takalign M, Abiye A, Srivastya KL, Asgelil D (eds). Improved management of vertisols for sustainable crop-livestock production in the Ethiopian highlands: Synthesis report 1986-92. Technical committee of the joint vertisol project, Addis Ababa, Ethiopia. pp. 103-137.
|
|
Abiye A, Ferew K (1993). Modifying the traditional plough (marseha) for better management of vertisols. In: Takalign M, Abiye A, Srivastya KL, Asgelil D (eds). Improved management of vertisols for sustainable crop-livestock production in the Ethiopian highlands: Synthesis report 1986-92. Technical committee of the joint vertisol project, Addis Ababa, Ethiopia. pp. 85-101.
|
|
|
Adams WA (1973). The effect of organic matter on the bulk and true densities of some uncultivated podzolic soils. J. Soil Sci. 24:10-17.
Crossref
|
|
|
Ali Y (1992). Nitrogen transformation in some Ethiopian highland Vertisols. PhD thesis, University College of Wales, Aberystwth, UK. P 174.
|
|
|
Amare G (1980). Agro-climates and agricultural systems in Ethiopia, Agric. Syst. 5:39-50.
Crossref
|
|
|
Association of Official Agricultural Chemists (AOAC) (2000). Official methods of analysis, 17th ed. Gaithersburg, Maryland, USA.
|
|
|
Asnakew W (1988). Physical properties of Ethiopian Vertisols. In: Jutzi, SC, Haque I, McIntire J and Stares JES (eds). Management of Vertisols in sub-Saharan Africa. Proceedings of a conference held at ILCA, Addis Ababa Ethiopia 31 August - 4 September 1987. pp. 111-123.
|
|
|
Barreto R, Madari BE, Maddock JEL, Machado PLOA, Torres E, Franchini J, Costa AR (2009). The impact of soil management on aggregation, carbon stabilization and carbon loss as CO2 in the surface layer of a Rhodic Ferralsol in southern Brazil. Agric. Ecosyst. Environ. 132(3-4):243-251.
Crossref
|
|
|
Bouyoucos GJ (1951). Arecalibration of the hydrometer method for the analysis of soils. Agron. J. 43(9):434-437.
Crossref
|
|
|
Christensen BT (2001). Physical fractionation of soil and structural and functional complexity in organic matter turnover. Eur. J. Soil Sci. 52:345-353.
Crossref
|
|
|
Constable M (1984). Resources of rural development in Ethiopia: Ethiopian Highlands. Reclamation Study. Working paper 17, FAO and Ministry of Agriculture, Addis Ababa. Ethiopia.
|
|
|
de Hann CH, Steinfeld H, Blackburn H (1998). Livestock-environment interaction: issues and options. Report of a study. WRENmedia, Fressingfield, EYE, Suffolk, IP21 5SA United Kingdom.
|
|
|
Desta B (1988). Soil fertility research on some Ethiopian Vertisols. In: Jutzi SC, Haque I, McIntire J and Stares JE S (eds), Management of Vertisols in sub-Saharan Africa Proceedings of a conference held at ILCA, Addis Ababa, Ethiopia, 31 August – 4 September 1987. pp. 223-231.
|
|
|
Edwards JH, Wood CW, Thurlow DL, Ruf ME (1992). Tillage and crop rotation effects on fertility status of a Hapludult soil. Soil Sci. Soc. Am. J. 56:1577-1582.
Crossref
|
|
|
Food and Agriculture Organization (FAO) (1988). A summary of the agricultural ecology of Ethiopia. FAO, Rome.
|
|
|
Food and Agricultural Organization (2001). Soil carbon sequestration for improved land management. Food and Agricultural Organization, World Soil Resources Report No. 96. Rome. Viewed on 8 March 2013 View
|
|
|
Food and Agricultural Organization (2004). Carbon Sequestration in Dry lands. Food and Agricultural Organization, Rome.
|
|
|
FAO (2006). Livestock's Long Shadow: environmental issues and options, LEAD website.
|
|
|
Franzluebbers AJ, Stuedemann JA, Schomberg HH, Wilkinson SR (2000). Soil organic C and N pools under long-term pasture management in the Southern Piedmont USA. Soil Biol. Biochem. 32:469-478.
Crossref
|
|
|
Hadera G (2001). Role of livestock in food security and food self sufficiency in the Highland production system: Livestock in Food Security – Roles and Contributions Proceedings of the 9th annual conference of the Ethiopian Society of Animal Production (ESAP) held in Addis Ababa, Ethiopia, August 30-31, 2001.
|
|
|
Haque I (1992). Use of legume biological nitrogen fixation in crop and livestock production systems. In: Mulongoy K, Gueye M and Spencer, DSC (eds). Biological nitrogen fixation and sustainability of tropical agriculture. A Wiley Saye Co-publication. pp. 423-437.
|
|
|
Haque I, Mesfin A, Tekalign M, Asgelil D (1993). Nutrient Management. In: Takalign M., Abiye A, Srivastya KL, Asgelil D (eds). Improved management of vertisols for sustainable crop-livestock production in the Ethiopian highlands: Synthesis report 1986-92. Technical committee of the joint vertisol project, Addis Ababa, Ethiopia. pp. 51-73.
|
|
|
Hernanz JL, Sanchez-Giron V, Navarrete L (2009). Soil carbon sequestration and stratification in a cereal/leguminous crop rotation with three tillage systems in semiarid condition, Agric. Ecosyst. Environ. 133(1-2):114-122.
Crossref
|
|
|
Improving Productivity Market Success (IPMS) (2004). Diagnosis Survey of Ad Liben Woreda, ILRI, Addis Ababa, Ethiopia.
|
|
|
Jastrow JD (1996). Soil aggregate formation and the accrual of particulate and mineral-associated organic matter. Soil Biol. Biochem. 28:665-676.
Crossref
|
|
|
Jutzi SC, Anderson FM, Abiye A (1987). Low cost modifications of the traditional Ethiopian tine plow for land shaping and surface drainage of heavy clay soils: Preliminary results from on-farm verification. ILCA Bull. 27:28-31. ILCA, Addis Ababa, Ethiopia.
|
|
|
Kahsay B (2004). Land use and land cover changes in the central highlands of Ethiopia: The case of Yere Mountains and its surroundings, M.Sc. Thesis, Addis Ababa University.
|
|
|
Lal R (2002). Carbon sequestration in dryland ecosystems of west Asia and north Africa. Land Degradation Dev. 13:45-59.
Crossref
|
|
|
Lal R (2004). Soil carbon sequestration impacts on global climate change and food security. Science 304(5677):1623-1627. www.sciencemag.org Viewed on 8 March 2013
Crossref
|
|
|
Lal R (2009). Sequestering atmospheric carbon dioxide. Crit. Rev. Plant Sci. 28:90-96.
Crossref
|
|
|
Lemus R, Lal R (2005). Bioenergy Crops and carbon sequestration, Crit. Rev. Plant Sci. 24:1-21.
Crossref
|
|
|
Mann LK (1986). Changes in soil carbon after cultivation. Soil Sci.142:279-288.
Crossref
|
|
|
Mengistu G, Fassil K (2010). Land Use Change Effect on Soil Carbon Stock, Above Ground Biomass, Aggregate Stability and Soil Crust: A Case from Tahtay Adyabo, North Western Tigray, Northern Ethiopia. J. Drylands 3(2):220-225.
|
|
|
Mesfin A, Jutzi S (1993). The joint project on vertisols management. Retrospect and prospect. In: Takalign M, Abiye A, Srivastya KL, Asgelil D (eds). Improved management of vertisols for sustainable crop-livestock production in the Ethiopian highlands: Synthesis report 1986-92. Technical committee of the joint vertisol project, Addis Ababa, Ethiopia pp. 147-157.
|
|
|
Milne E, Heimsath A (2012). Soil organic carbon, In: Cutler J. Encyclopedia of Earth. (Ed). Environmental Information Coalition, National Council for Science and the Environment, Cleveland Washington, D.C. Retrieved March on 13, 2013
View
|
|
|
Ministry of Finance and Economic Development (MoFED) (2009). GTP annual progress report 2010/11. www.mofed.gov.et. Viewed on 17 March 2013.
|
|
|
Nordhaus W (2008). A Question of Balance. Yale, CT: Yale University Press. P 209.
|
|
|
Pearson T, Walker S, Brown S (2005). Sourcebook for land use, land-use change andforestry projects. Winrock International and the Biocarbon Fund of the World Bank. P 57.
|
|
|
Post WM, Kwon KC (2000). Soil Carbon sequestration and land-use change, Processes and potential, Glob. Chang Biol. 6:317-328.
Crossref
|
|
|
Rosenberg NJ, Izaurralde RC, Malone EL (1999). Carbon sequestration in soils: science, monitoring and beyond. In Proceedings of St. Michaels Workshop, Columbus, OH, December 1998.
|
|
|
Statistical Product and Service Solutions (SPSS) (2008). SPSS version 17.0.1, SPSS Inc., Chicago Ill
|
|
|
Srivastava KL, Abiye A, Tekalign M, Hailu R, Selamyihun K (1993). Land, soil and water management In: Takalign M, Abiye A, Srivastya KL, Asgelil D (eds). Improved management of vertisols for sustainable crop-livestock production in the Ethiopian highlands: Synthesis report 1986-1992. Technical committee of the joint vertisol project, Addis Ababa, Ethiopia. pp. 75-84.
|
|
|
Sundermeier A, Reeder R, Lal R (2005). .Soil Carbon Sequestration—Fundamentals, Extension Fact Sheet AEX–510–05, The Ohio State University Extension, Columbus, OH.
|
|
|
Tilahun Chibsa, Asefa Ta (2009). Asessment of Soil Organic Matter Under Four Land Use Systems in the Major Soils of Bale Highlands, South East Ethiopia. B. Factors affecting Soil Organic Matter Distribution. World Appl. Sci. J. 6(11):1506-1512.
|
|
|
Walkley A, Black IA (1934). An examination of Degtjareffmethod for determining soil organic matter and a proposed modification of the chromic acid titration method. Soil Sci. 37:29-37.
Crossref
|
|
|
Whendee L, Neff SJ, McGroddy M, Veldkamp Ed, Keller M (2000). Effects of Soil Texture on Belowground Carbon and Nutrient Storage in a Lowland Amazonian Forest Ecosystem. J. Ecosyst. 3(2):193-209.
Crossref
|
|
|
World Overview of Conservation Approaches and Technology Categorization System (WOCAT) (2013). n.d..
|
|