ABSTRACT
Spawn quality is an important factor that affects the final yield in mushroom production. One of the most common risks is the contamination of substrates and spawn by mitosporic fungi. Some species do not easily grow on grain substrate as traditional species do and these find it real difficult to produce spawn. A method was evaluated to produce spawn for mushroom production based on aseptically encapsulated mycelium using alginate beads. Strains of four selected edible mushrooms were cultivated in liquid medium, mycelium was harvested by filtration. Subsequently, it was blended with 100 mL of 2% (w/v) sodium alginate solution or with the alginate solution with the addition of 2% (w/v) yeast extract solution or with the addition of 2% (w/v) meat extract solution. An electronic mixer was used to disaggregate the mycelium and generate a homogeneous suspension. To obtain the artificial spawn, the cells suspensions were dripped in 200 mL of 500 mM CaCl2 solution using a cut micro-tip. Beads obtained were inoculated in potato dextrose agar Petri dishes and in substrate (wheat straw or Poplar sawdust) to evaluate the growth. Tests carried out using this method, demonstrated that the incorporation of a suitable nutrient increases the ability of selected mushroom to emerge from the beads. Mycelia colonization tests were carried out on sterilized substrate, one using alginate beads and another one using the traditional spawn as inoculum. No significant differences according to t-test (p<0.05) were shown. However, spawning production based on alginate beads can be performed in only a few days, whereas the traditional method requires more time. The method proposed here represents an antecedent to improve spawn generation for future industrial applications, for further studies and for other uses as mycelium inoculant.
Key words: Alginate beads, inoculum, mushroom cultivation, spawn production.
The cultivation of edible mushrooms is a worldwide important commercial activity (Chang, 2000). Mushrooms are attractive functional food and a source for the development of drugs and nutraceuticals (Lakhanpal and Rana, 2005; Albertó et al., 2010). These characteristics, together with the use of agro-industrial wastes for its production, have contributed to the development of this activity around the world (Lechner and Albertó, 2007, 2011; Philippoussis et al., 2000, 2001; Royse, 2013). The spawn quality is an important factor that affects the final yield in the mushroom production. At present, a variety of different substrates such as: manure, tobacco stems and wheat, rye or millet grains have been successfully used in the spawn preparation (Salmones et al., 1999; San Antonio, 2011).
The method worldwide employed at present was devised by Sinden (1935). This method is based on the use of cereal grains as substrate giving not only a physical matrix that is used as carrier, but a source of carbon (C) and nitrogen (N).
This is an eight-step method: 1) boiling the grains in water; 2) draining the water; 3) addition of 1% w/w CaCO3; 4) sterilizing at 120°C; 5) cooling at 25°C, 6) inoculating agar plugs of selected strains; 7) incubating at 25°C and 8) periodical shaking during 20-30 days (Jaramillo and Albertó, 2013).
One of the most important problems during mushroom cultivation is the contamination of the substrate or spawn by mitosporic fungi such us Trichoderma spp., an environmental recurrent fungus of fast growth which is frequently the cause of high loses. This infection in edible basidiomycetes has been known for a long time. The contamination of mushroom substrate and spawn by this fungus is known as green mold disease (Hatvani et al., 2007). Trichoderma sp. is often observed in the early stages of the process, especially during spawning run period, but also during cropping period and causes huge losses in mushrooms crops (Jandaik and Guleria, 1999). An Agaricus green mold disease started in Northern Ireland in 1985 and rapidly spread over farms across Europe and was quickly succeeded by subsequent outbreaks all along Ireland in 1986, in England and Scotland in 1987, in the Netherlands in 1994, in France in 1997 and in Spain in 1998. This disease also occurred in the United States and Canada causing important economic losses (Colavolpe et al., 2014).
In the spawn generation, the contaminations occur because of wrong handling during spawn production or by intrinsic grain microbial contamination. The microbial populations change among grains batches and the mitosporic fungi present in cereals constitute a potential problem for spawn production (Laca et al., 2006; Sreenivasa et al., 2010). This heterogeneity between batches constitutes a problem for spawn generation. Although, there were many improvements in the mushroom industry on the method worldwide employed, the problem of contamination is only partially controlled with adapted sterilization; the topic is still cause of economic loses (Hatvani et al., 2002) and it is not totally solved. The variability of nutrient composition, water content, osmolality and pH of grains constitute another disadvantage of spawn generation using the traditional method (Romaine, 1987). Some species do not easily grow on grain substrate (Agaricus spp. or Gymnopilus pampeanus) as traditional species do (Pleurotus spp, Lentinula edodes, etc) and this is really difficulty to produce spawn.
Alginate is an anionic polymer typically obtained from brown seaweed; this polymer has a number of properties such as: biocompatibility, low toxicity, low cost and mild gelation by addition of divalent cations such as Ca2+. Due to these properties, Alginate has wide uses in different biotechnological applications including drugs and protein delivery, enzyme immobilization, cell culture, microbial encapsulation, artificial seed generation among others (Smidsrød and Skjåk-Bræk, 1990; Romaine and Schlagnhaufer, 1992; Díaz et al., 2009; Lee and Mooney, 2012; Peart et al., 2012; Sakhanokho et al., 2013; Zhao et al., 2016). In particular, the microbial encapsulation improved the viability of microorganisms under storage or in adverse environmental conditions. In the process of encapsulation, the microorganisms are confined to a micro-environment, this micro-environment can be rationally designed including specific nutrients to favor the maintenance or microorganism development (Khosravi et al., 2014). Immobilization based on encapsulation offers a high level of protection to the inocula from environmental stress (Friel and McLoughlin, 1999).
Taking into account the difficulties mentioned in the spawn generation using natural substrates such as grains, culturing nontraditional species of mushrooms and the advantages that the use of alginate fungal encapsulation mentioned above could provide, the aim of this work is to generate an artificial spawn for mushroom production based on alginate encapsulation of mycelium.
Mushrooms used
The strains used in this work were: Pleurotus ostreatus: ICFC 153/99, commercial strain, 19-XI-1999, leg. E. Albertó. Gymnopilus pampeanus: ICFC 748/12, Chascomús, Buenos Aires, Argentina; growing on Eucalyptus, 04-X-2011, leg. M. B. Colavolpe. L. edodes: ICFC 293/99, United States, from Northwest Mycological, Consultants, 27/IV/00, leg. E. Albertó. Agaricus bisporus: ICFC 745/12, Xalapa, Veracruz, Mexico, 25/VIII/11 leg. M. Sierra Marina. ICFC strains are conserved in the IIB-INTECH Collection of Fungal Cultures (ICFC), reference in the WDCM database: WDCM 826. All the strains were periodically propagated and maintained in potato dextrose agar (PDA; Britania®) at 4°C.
Spawn production
Spawn was prepared following Lechner and Albertó (2011). Briefly, wheat grains were prepared by soaking and boiling in 1% (w/v) of CaCO3. The grains were sterilized in autoclave for 2 h at 1.2 psi and 120°C and inoculated with peripheral mycelia PDA plug (1 cm diameter) cut from the advancing margin of a 5-days-old colony. The cultures were maintained at 25 ±2°C, with periodical shaking for 30 days.
Artificial spawn: Alginate beads preparation
The strains were grown in darkness at 25±2°C and 150 rpm, using 250 mL Erlenmeyer flasks containing 50 mL of 2% (w/v) yeast extract medium inoculated with 9 plugs (5 mm diameter) of a peripheral mycelium of a 5-days-old colony grown in PDA. Sodium alginate used here was obtained from brown seaweeds of the phylum Phaeophyceae. After 6 days of incubation, the mycelia were harvested by filtration through Whatman #1 paper and washed with sterile water. Subsequently, the fungal mycelia were blended with i) 100 mL of 2% (w/v) sodium alginate solution (AG) or ii) 2% (w/v) sodium alginate plus 2% (w/v) yeast extract solution (AGY) or iii) 2% (w/v) of sodium alginate plus 2% (w/v) meat extract (2%) solution (AGM). After this step, a mixer was used to disaggregate the mycelium and generate homogeneous cells suspensions. To obtain the artificial spawn, the cells suspensions were dripped in 200 mL of 500 mM CaCl2 solution at room temperature using a cut 1000 µL micro-tip at 3 mm from the top. Finally, the artificial spawn was harvested by filtration using a sterile Whatman #1 paper and left on an absorbent filter paper for 10 min in a laminar flow chamber. Under these conditions, the resulting artificial spawn was 5±0.5mm of diameter. All solutions were sterilized in autoclave for 20 min at 1.2 psi and 120°C.
Radial growth assays
The artificial spawn was inoculated in PDA Petri dishes. Plates were incubated at 25±2°C in darkness. Mycelia growth was evaluated by measuring colony diameter using a caliper; three replicates were performed per assay. The experimental tests were performed in triplicate.
Substrate assays
Polypropylene bags (10 x 25 cm) containing 100 g of substrates poplar sawdust or wheat straw, were moistened to 70% (v/w) with water and sterilized in autoclave at 121°C for 2 h. The substrates were inoculated with artificial spawn (encapsulated mycelia in alginate beads) or conventional spawn (mycelia on sterilized grains) using different strains. The percentage of inoculation for P. ostreatus and G. pampeanus was 5%. Meanwhile, the percentages for A. bisporus and L. edodes were 0.4 and 7%, respectively. In all cases, the percentages used for inoculation are those frequently used by mushroom industry. Information on the number of beads and grains in 100 g of substrate is given in Table 1. The inoculated bags were maintained for 7 days at 25±2°C and, at the end of this period, the percentage of mycelia growth was evaluated as described below. The total substrate area of bags was divided into eight quadrants (four quadrants per side of the bag with an area of 12.25% per quadrant); and the superficial mycelia colonization was visually estimated (Figure 1). To obtain the total percentage of colonization, the coverage area was referred to the total bag area (Colavolpe et al., 2014). Additionally, the total number of inoculum points (number of grains or beads) was determined. All assays were performed by triplicate and analyzed by non-parametric test, using the statistics software GraphPad® Prims trial version 5.0.
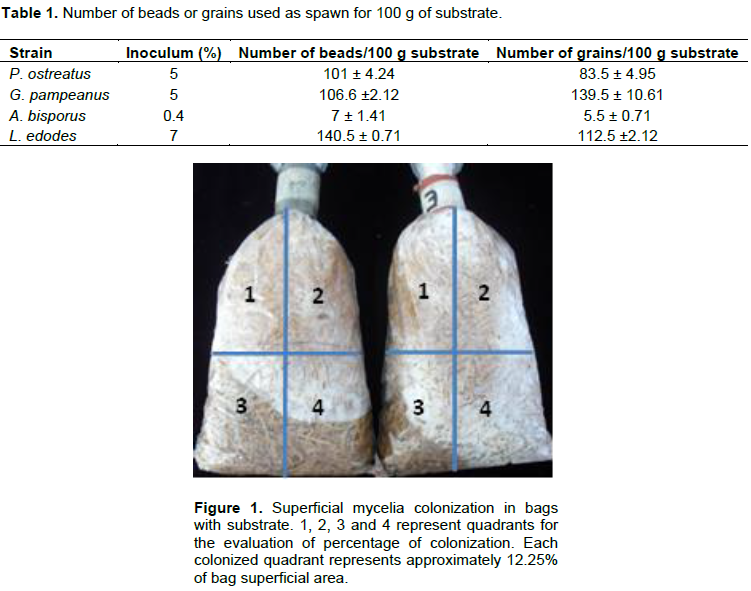
Short and long term preservation
The artificial spawns were placed in screw cap vials containing 10 mL of sterilized water or glycerol solution 20% (v/v). The vials were stored for two weeks in refrigerator at 4°C in the first case and 6 months at -20°C. After this period, PDA Petri dishes were inoculated with artificial spawns and incubated at 25±2°C. Three replicates were performed for each treatment. Cryo-preserved spawn was also inoculated on bags containing sterilized substrate which were prepared as explained above.
Effect of nutrient incorporation on artificial spawn
To evaluate the effect of nutrient incorporation in artificial spawn generation, the ability of the mycelium to emerge from the artificial spawn and colonize the substrate was evaluated by diameter of colony measurement. Figure 2 shows that incorporation of yeast or meat extract during the encapsulation process generated an increase in the capacity of the mycelia to emerge from the beads. However, the effect of the nutrient source added in the process of encapsulation affected the emerging capacity and this effect resulted particular from each species; this fact could be clearly observed for P. ostreatus and A. bisporus. For the former species, the addition of meat extract enhanced growth and Petri dishes were covered in 4 days; in the case of the L. edodes, the addition of yeast extract reduced the time needed to colonize the Petri dishes. On the other hand, no significant difference with additional nutrient source could be observed in L. edodes and G. pampeanus indicating that additional nutritional source in order to improve mycelia growth and enhance the emerging capacity from the bead should be evaluated for each species of commercial mushroom in particular. Based on the results obtained here, nutrient formulation in beads varies with the different fungal species. Additionally, if we compare the growth of G. pampeanus at 25±2°C obtained using beads with the growth obtained by Colavolpe and Albertó (2014) using plugs of agar for strain ICFC 748/12, we can observe that it is very similar, which means that the encapsulated mycelium has not negatively influenced the mycelium growth capacity.
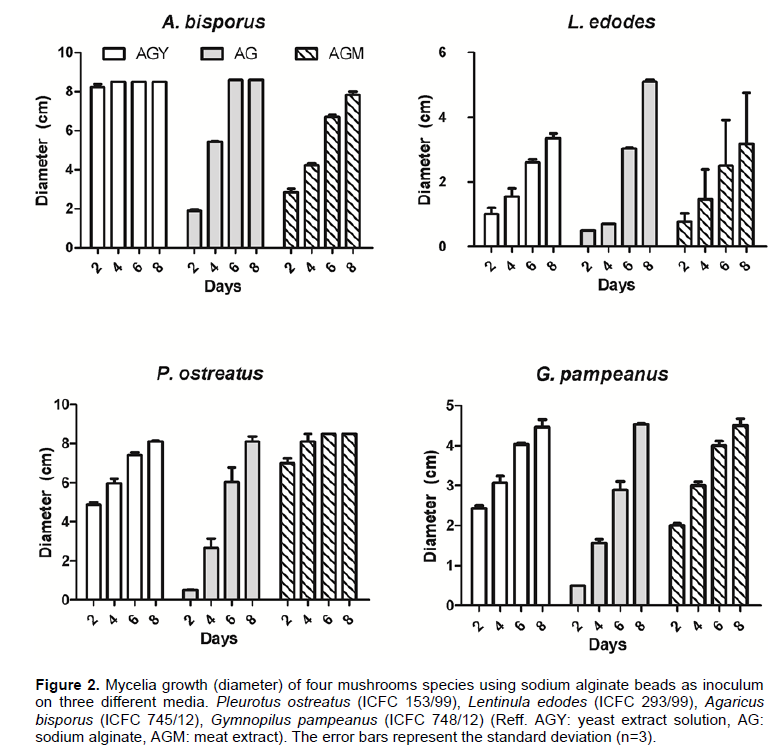
Substrate colonization
The superficial mycelium colonization on the sides of sterilized substrate bags were compared after 7 days incubation using alginate beads or traditional spawn as inoculum. In this experiment, the percentage of substrate colonization and the inoculum points were evaluated as response, in the four strains. Significant differences were not detected between alginate beads or traditional spawn in any treatment when responses were analyzed using t-test for nonparametric statistical analysis (Figure 3). In these assays, sterilized substrates were used to compare all treatments. Some mushrooms species such as A. bisporus for example, are cultivated on pasteurized substrates. Friel and McLoughlin (1999) compared the colonisation of pasteurised compost by entrapped and co-entrapped mycelial beads and conventional grain spawn proving that the beads could colonize pasteurised compost. So, encapsulate mycelia could be used both on sterilized and pasteurized substrate.
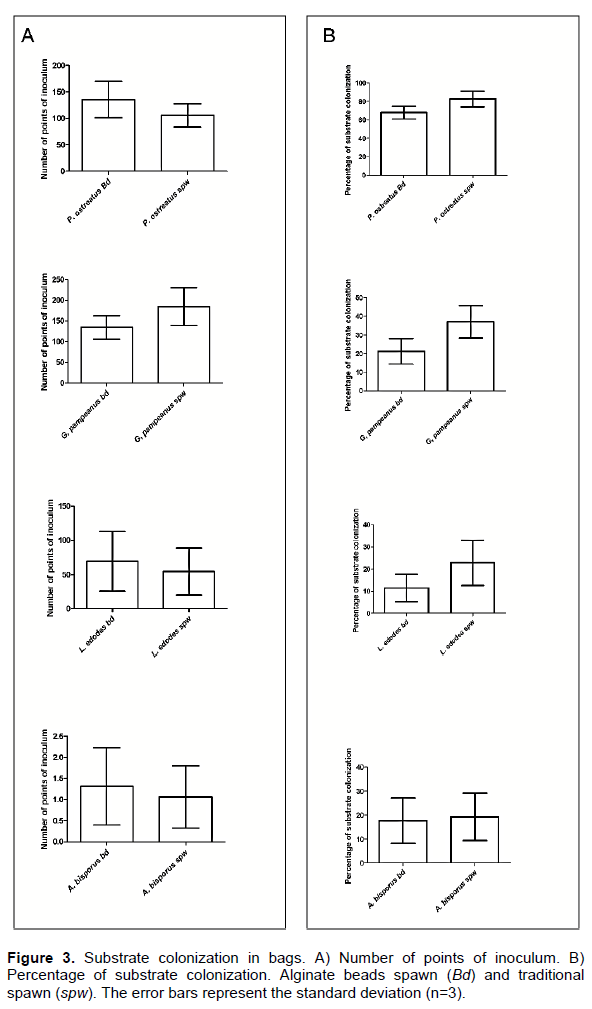
Alginate beads did not increase the mycelia coverage in relation to spawn but managed a very good colonization of the substrates without any contamination. It was considered that this work represents a first step for testing the efficiency of beads methodology at laboratory scale.
Grains of cereals used for spawn production usually carry many fungi (Fusarium spp., Trichoderma spp., Aspergillus spp., Rhizopus spp., etc) which frequently produce contaminations on the spawn. This is a serious problem because it reduces the profitability of mushroom production and consequently, it causes economic losses (Lee at al., 1986). The alginate beads avoid contaminations since they are produced under sterilized conditions. The number of points of inoculum depends on the grain used to produce the spawn. A higher number of points of inoculum help in a better colonization of the substrate. Actually, if the grain is small, the farmer will have a higher number of points of inoculum by weight of spawn and as a result, a higher number of colonies and a faster colonization of the substrate (Salmones et al., 1999). On the other hand, with the use of beads, although the number of points of inoculum is higher than with the use of grains, we do not observe a higher effect on the percentage of colonization. Anyway, an optimized range of bead size could be designed and a number of beads per area surface can be determined for a faster colonization of the substrate. Some nontraditional species such as Gymnopilus pampeanus, which have serious problems to grow on grain, also manage to properly grow.
Long and short term preservation of beads
In order to examine the viability of mycelia under preservation conditions, after two weeks or six months at -20°C, beads were placed on PDA plates and growth was evaluated. All the strains grew at 20°C, except for L. edodes. In this case, it could probably have been due to the strain inability to grow after a storage period. As it usually occurs with fungi, there is no unique method for mycelia preservation that works with the same efficiency for all fugal species (García-García et al., 2014; Hu et al., 2014). Additionally, the alginate beads were also viable for substrate colonization after thawing (data not shown). Artificial spawn thus, has an extra advantage over traditional spawn. It is usually recommended to use spawn before one month of storing at 4°C, the artificial spawn can be kept frozen for six months at least in three of the four species assayed.
In the present work, a method to produce spawn based on encapsulated fungal mycelia using alginate beads for spawning was used. The tests carried out using this method demonstrated that the incorporation of a suitable nutrient increases the ability of mycelia to emerge from the beads at least in P. ostreatus and A. bisporus. No significant differences were found between inoculations and traditional spawn or alginate beads when the mycelium colonization test was done on sterilized substrate. However, production of spawn based on alginate beads can be carried out in very few days as compared to the traditional method that requires 20 to 30 days of incubation. This work represents a previous step for a future industrial application. Additionally, there are some practical advantages since beads can be maintained frozen for six months and the mycelia remain viable in most of the species assayed. The methodology presented here to produce alginate beads was developed at laboratory scale, but it could be possible to design an automatic machine to produce the spawn in a quick, safe and efficient way in order to be applied in mushroom industry. At present, there are peristaltic pumps that can produce alginates beads drop by drop improving the speed of the process (Takka and Gürel, 2010; Swioklo et al., 2016). Based on the comments made above and time being a valuable resource, it can be considered that the method proposed here is an adequate alternative for improving spawning generation not only for mushroom production but also for any other bioprocess that requires any fungal inoculum.
The authors have not declared any conflict of interests.
REFERENCES
Albertó EN, Curvetto J, Deschamps RM, Lechner B (2010). Hongos Silvestres y de Cultivo en Argentina: Historia, regiones y sistemas de producción, hongos silvestres de valor económico, consumo, mercado interno y externo, legislación, oferta tecnológica e investigación y desarrollo. In: Martínez-Carrera, En., Curvetto, D., Sobal, N., Morales, M., Mora, P., V.M. (Eds.), Hacia un Desarrollo Sostenible del Sistema de Producción-Consum de los Hongos Comestibles y Medicinales en Latinoamérica: Avances y Perspectivas en el Siglo XXI. Red Latinoamericana de Hongos Comestibles y Medicinales, 19. COLPOS-UNSCONACYT-AMC-UAEM-UPAEP-IMINAP, Puebla, pp. 333-358.
|
|
Chang ST (2000). Global impact of edible and medicinal mushrooms on human welfare in the 21st century: no green revolution. Int. J. Med. Mushrooms 1:1-7.
Crossref
|
|
|
Colavolpe MB, Albertó E (2014). Cultivation requirements and substrate degradation of the edible mushroom Gymnopilus pampeanus-A novel species for mushroom cultivation. Sci. Hortic. 180:161-166.
Crossref
|
|
|
Colavolpe MB, Jaramillo Mejía S, Albertó E (2014). Efficiency of treatments for controlling Trichoderma sp during spawning in mushroom cultivation. Braz. J. Microb. 45:1263-1270.
Crossref
|
|
|
Díaz G, Carrillo C, Honrubia M (2009). Production of Pinus halepensis seedlings inoculated with the edible fungus Lactarius deliciosus under nursery conditions. New For. 38(2):215-227.
Crossref
|
|
|
Friel MT, McLoughlin AJ (1999). Immobilisation as a strategy to increase the ecological competence of liquid cultures of Agaricus bisporus in pasteurised compost. FEMS Microbiol. Ecol. 30(1):39-46.
Crossref
|
|
|
García-García M, Rocha-Zavaleta L, Valdez-Cruz NA and Trujillo- Roldán MA (2014). Conservation of the mycelia of the medicinal mushroom Humphreya coffeata (Berk.) Stey. in sterile distilled water. MethodsX 1:19-22.
Crossref
|
|
|
Hatvani N, Kredics L, Antal Z, Mécs I (2002). Changes in activity of extracellular enzymes in dual cultures of Lentinula edodes and mycoparasitic Trichoderma strains. J. Appl. Microbiol. 92(3):415-423.
Crossref
|
|
|
Hatvani L, Antal Z, Manczinger L, Szekeres A, Druzhinina IS, Kubicek CP and Kredics L (2007). Green mold diseases of Agaricus and Pleurotus spp. are caused by related but phylogenetically different Trichoderma species. Phytopathology, 97(4):532-537.
Crossref
|
|
|
Jandaik S, Guleria DS (1999). Yield loss in Agaricus bisporus due to Trichoderma sp infection. Mushroom Res. 8:43-46.
|
|
|
Jaramillo S, Albertó E (2013). Heat treatment of wheat straw by immersion in hot water decreases mushroom yield in Pleurotus ostreatus. Rev. Iberoam. Micol. 30(2):125-129.
Crossref
|
|
|
Hu X, Webster G, Xie L, Yu C, Li Y and Liao X (2014). A new method for the preservation of axenic fungal cultures. J. Microbiol. Methods 99:81-83.
Crossref
|
|
|
Khosravi Zanjani MA, Tarzi, BG, Sharifan A, Mohammadi N (2014). Microencapsulation of probiotics by calcium alginate-gelatinized starch with chitosan coating and evaluation of survival in simulated human gastro-intestinal condition. Iran J. Pharm. Res. 13:843-852.
|
|
|
Laca A, Mousia Z, Díaz M, Webb C, Pandiella SS (2006). Distribution of microbial contamination within cereal grains. J. Food Eng. 72:332-338.
Crossref
|
|
|
Lakhanpal TN, Rana M (2005). Medicinal and nutraceutical genetic resources of mushrooms. Plant Genet. Resour. Charact. Util. 3:288-303.
Crossref
|
|
|
Lechner B, Albertó E (2007). Optimal conditions for the fruitbody production of naturally occurring strains of Lentinus tigrinus. Bioresour. Technol. 98:1866-1869.
Crossref
|
|
|
Lechner BE, Albertó E (2011). Search for new naturally occurring strains of Pleurotus to improve yields. P. albidus as a novel proposed species for mushroom production. Rev. Iberoam. Micol. 28:148-154.
Crossref
|
|
|
Lee KY, Mooney DJ (2012). Alginate: Properties and biomedical applications. Prog. Polym. Sci. 37:106-126.
Crossref
|
|
|
Lee US, Jang HS, Tanaka T, Toyasaki N, Sugiura Y, Oh YJ, Cho CM, Ueno Y (1986). Mycological survey of Korean cereals and production of mycotoxins by Fusarium isolates. Appl. Environ. Microbiol. 52:1258-1260.
|
|
|
Peart PC, Chen ARM, Reynolds WF, Reese PB (2012). Entrapment of mycelial fragments in calcium alginate: A general technique for the use of immobilized filamentous fungi in biocatalysis. Steroids 77:85-90.
Crossref
|
|
|
Philippoussis A, Diamantopoulou P, Zervakis G, Ioannidou S (2000). Potential for the cultivation of exotic mushroom species by exploitation of Mediterranean agricultural wastes. Mushroom Sci. 15:523-530.
|
|
|
Philippoussis A, Zervakis G, Diamantopoulou P (2001). Bioconversion of lignocellulosic wastes through the cultivation of the edible mushrooms Agrocybe aegerita, Volvariella volvacea and Pleurotus spp. World J. Microbiol. Biotechnol. 17:191-200.
Crossref
|
|
|
Romaine P, Nelsen C, Davis E (1987). Synthetic substrate for filamentous fungi. Patent US4803800 A.
|
|
|
Romaine CP, Schlagnhaufer B (1992). Characteristics of a hydrated, alginate-based delivery system for cultivation of the button mushroom. Appl. Environ. Microbiol. 58(9):3060-3066.
|
|
|
Royse DJ (2013). Trends in mushroom production worldwide. Proceedings of the 7th International Symposium on Mushrooms in Brazil, Manaus, Brazil. pp. 38-47.
|
|
|
Sakhanokho HF, Pounders CT, Blythe EK (2013). Alginate encapsulation of Begonia microshoots for short-term storage and distribution. Sci. World J. 7p.
|
|
|
Salmones D, Mata G, Ramos LM, Waliszewski KN (1999). Cultivation of shiitake mushroom, Lentinula edodes, in several lignocellulosic materials originating from the subtropics. Agronomy 19:13-19.
Crossref
|
|
|
San Antonio JP (2011). Origin and Improvement of Spawn of the Cultivated Mushroom Agaricus brunnescens Peck, in: Horticultural Reviews. John Wiley & Sons, Inc., Hoboken, NJ, USA pp. 85-118.
Crossref
|
|
|
Sinden JW (1935). Mushroom spawn and method of making it. Patent US2044861 A.
View
|
|
|
Smidsrød O, Skjåk-Bræk G (1990). Alginate as immobilization matrix for cells. Trends Biotechnol. 8:71-78.
Crossref
|
|
|
Sreenivasa Y, Dass RS, Janardhana GR (2010). Survey of postharvest fungi associated with sorghum grains produced in karnataka (India). J. Plant Protect. Res. 50:335-339.
|
|
|
Swioklo S, Ding P, Pacek AW, Connon CJ (2016). Process parameters for the high-scale production of alginate-encapsulated stem cells for storage and distribution throughout the cell therapy supply chain. Process Biochemistry.
Crossref
|
|
|
Takka S, Gürel A (2010). Evaluation of Chitosan/Alginate Beads Using Experimental Design: Formulation and In Vitro Characterization. AAPS Pharm. Sci. Technol. 11(1):460-466.
Crossref
|
|
|
Zhao D, Li JS, Suen W, Chang MW, Huang J (2016). Preparation and characterization of Ganoderma lucidum spores-loaded alginate microspheres by electrospraying. Mater. Sci. Eng. C 62:835-842.
Crossref
|
|