ABSTRACT
Efficiency of biosorbent from chitosan coated dead fungal biomass of Aspergillus niger for dye removal with its impact on metals and physicochemical parameters of textile industry wastewater were assessed. Dye and metal concentrations of wastewater were determined using UV and Atomic Absorption Spectrophotometers respectively, before and after column biosorption using simulation optimized parameters with biosorbent. Physicochemical parameters were determined using standard methods. Simulated and industrial wastewaters data were analysed statistically with one way ANOVA and T-test. Biosorption of dye was classified with Langmuir and Freundlich isotherms. Results showed that at dye concentration of 100 mg/L, 6.90 mg/g of indigo dye adsorbed per mass (qe); in 1.87 mg/L of wastewater, 0.07 mg/g of dye adsorbed per unit mass (qe). Isotherm model fitted well to Freundlich with constant 0.04 and 8.45 mg/g in textile and simulated wastewater respectively. Metals in wastewater ranged from 0.071 to 1.102 mg/L. Acidity, COD Zn, Pb, Fe, Ni, Mn, Cu, Cd, Cr, Co, Mg ions were reduced by 32.94, 39.02, 84.95, 34.57, 44.19, 42.19, 49.09, 25.13, 43.66 and 32.03% respectively after treatment while TDS, conductivity, alkalinity, turbidity, pH increased. Chitosan coated A. niger could be effective in removing dyes and heavy metals from textile wastewater.
Key words: Aspergillus niger, biosorbent, physico-chemical, heavy metals, textile wastewater.
The progress made in the development of Industries and technology has resulted in extra load of burden on the environment. This burden is linked to the large quantities of hazardous waste, heavy metals (cadmium, chromium, and lead) and metalloids such as arsenic and antimony, and organic contaminant having deleterious effect on the ecosystem (Ayangbenro et al., 2017). Heavy metal toxicity has proven to be a major threat and there are several health risks associated with it. The toxic effects of these metals interfere with the metabolic processes and sometimes get accumulated in the body and food chain and exhibit chronic nature (Jaishankar et al., 2014; Khan et al., 2015; Carolin et al., 2017). The textile industry being the largest dye stuff, water user and wastewater producer is not left out in inflicting this serious damage especially with the large volume of wastewater being discharged to the available water resources. The first modern textile industry in Nigeria, the Kaduna Textile Mill, started production in 1956. By the 70s and 80s, the Nigerian textile industry had grown to become the third largest in Africa. A report by the United Nations University (UNU) accounted for 37 textile firms in the country as of 1987, with operating 716,000 spindles and 17,541 looms. This was the golden period of Nigeria's textile industry. Environmental problems with used dyes baths are associated to the wide variety of different components added to the dye bath, often in relatively high concentrations. The dye itself is the greatest problem (Fersi et al., 2005). Textile wastewater is usually treated in an activated sludge plant not for reusing purposes but to meet regulatory standards for discharge, hence it is not surprising that an appreciable amount of physico-chemical contaminants such as Suspended Solids (SS), Chemical Oxygen Demand (COD), Biochemical Oxygen Demand (BOD), high pH and strong colour still remain in biologically treated textile effluents. Colour removal by conventional treatment methods (e.g. ozonation, bleaching, hydrogen peroxide/UV, electrochemical techniques) was found to be unsatisfactory (Marmagne and Coste, 1996; Ciardelli et al., 2001; Baig and Liechti, 2001) because most textile dyes have complex aromatic molecular structures that resist degradation and are stable to light, oxidizing agents and aerobic digestion.
Metals, on the other hand, are used in a variety of processing techniques in the textile industry, including oxidizers for vat and sulphur dyes, after treatment of direct dyes, metal catalyst used for curing resins, finishes (that is, flame retardant, soil release, and water repellent), dye stripping agents, and various classes of dyes. Majority of the common dye classes, acid, basic, direct, disperse, fiber reactive and vat all contain toxic metals including Cr, As, Cd, Hg, Cu, Pb and Zn (Leonas and Leonas, 1994; Ahalya et al., 2005). Generally, heavy metals are present in low concentration in wastewaters and are difficult to remove from water. There are many methods being used to remove and recover the metals from metal bearing industrial effluents and our environment and many physico-chemical methods have been presented for their removal from wastewater (Iqbal et al., 2002). These methods differ in their effectiveness and cost. Some of the methods such as chemical precipitation, reverse osmosis, ultrafiltration, electrochemical deposition etc become inefficient when contaminants are present in trace concentration (Muhammad et al., 1998). They are not environment friendly and do not seem to be economically feasible for such industries because of their relative high costs (Quek et al., 1998). During the 1970s, the increasing awareness and concern about the environment motivated research for new efficient and cost effective technologies that would be capable of removing metals and other organic contaminants such as dyes from polluted waste waters (Rao and Prabhakar, 2011). It was only in the 1990s that a new scientific area developed and helped to recover heavy metals and some other contaminants. The early reports described how abundant biological materials could be used to remove, at very low cost, even small amounts of toxic heavy metals from industrial effluents (Joshi, 2018). The main advantages of biological technologies for the removal of pollutants are that they can be carried out in situ at the contaminated site, usually environmentally benign (no secondary pollution) and they are cost effective. Biosorption is a simple technique that relies on the ability of living and nonliving biomass to remove metals or other organic contaminants from the matrix of interest (Ojima et al., 2019). Biosorption has been demonstrated to possess good potential to replace conventional methods for the removal of organic and inorganic contaminants (Malik, 2004; Di Piazza et al., 2017; Okoya et al., 2020a). This search brought biosorption/adsorption to the foreground of scientific interest as a potential basis for the design of novel wastewater treatment processes (Guendouz et al., 2016). Several adsorbents are currently used which are by-products from agriculture and industries, which include seaweeds, moulds, yeast., bacteria, fungi, crab shells, agricultural products such as wool, rice husk, cocoa husk, maize husks, straw, coconut husks, peat moss, exhausted coffee waste tea leaves, walnut skin, coconut fibre, Moringa oleifera husk etc. (Amuda et al., 2007; Okoya et al., 2014; Okoya et al., 2015; Okoya et al., 2020b). Therefore, the search for efficient, eco- friendly and low cost effective biosorbent for dye and heavy metals removal from wastewater is a continuous one. Hence this study seeks to assess the efficiency of the synergy of Aspergillus niger and chitosan from snail shell as biosorbent for the removal of heavy metals and indigo dye from textile industry wastewater.
Collection and preparation of materials
The A. niger isolate used for this research was collected from the Department of Microbiology, Obafemi Awolowo University, Ile- Ife while the snail shells used for the extraction of chitosan were collected from households in Ile-Ife, Osun State. The snail shells samples were first washed, sun dried and ground into a powdery form after which it was sieved with 150 µm size mesh. The less than 150µm sized powdered snail shell was later stored and used for the extraction of chitosan according to the procedure (Okoya et al., 2014). Analytically graded reagents were used for the extraction of chitosan, preparation of A. niger fungal biomass and all other experiments in this study.
Preparation of the powdered fungal biomass of A. niger
The A. niger isolate collected was inoculated into sterile petri-dishes of Potato Dextrose Agar (PDA) and incubated at 25°C for 5 days. After incubation, A. niger spores that had grown on the petri-dishes were harvested with distilled water in a 250 ml conical flask. Yeast extracts medium solution containing salts of KH2PO4; (NH4)2 SO4.7H2O; ZnSO4.7H2O and MgSO4.7H2O was later prepared and autoclaved at 121°C for 30 min. After allowing the autoclaved yeast medium salt solution to cool, the harvested A. niger spore solution was then inoculated into the yeast extract medium salt solution in different 250 ml conical flasks and mechanically agitated for 5 days to allow for maximum contact of the yeast medium and its content with the spores (del Rocío Urbina-Salazar et al., 2019). A. niger spores were later harvested by autoclaving the resultant contents of the conical flasks and filtering out the dead A. niger spores as the filtrate. The dead A. niger was pre-treated by washing with distilled water until the pH of the wash solution is close to neutral and then oven dried at about 70°C for 36 h. The dry biomass was ground to powder using a pestle and mortal. The powdered biomass was later sieved with a sieve opening of 150 µm and used as biosorbent.
Extraction of chitosan from snail shells
Chitosan was extracted from snail shell according to the method of Okoya et al. (2014) and Okoya, (2015). Snail shells samples were first washed, sun dried and ground into a powdery form after which it was sieved with 150 µm size mesh. The less than150 µm sized powdered snail shell was later deproteinised with 4% (w/v) KOH, the product demineralized with 3% (v/v) 1 M HCl, decolourised by refluxing in acetone for 3 h at 60°C to get chitin which was then deacetylated in 50% (w/v) NaOH solution on a magnetic stirrer at 30°C for 4 h to obtain the chitosan (2-acetamido-2-deoxy-β-D-glucose- (Nacetylglucosamine) shown in the following chemical reaction.
Characterization of chitosan
The chitosan powder was characterized using Fourier Transform Infra-red Spectroscopy (Perkin Elmer Spectrum BXl'l) to verify the structural modification upon extraction of chitosan from the snail shell (chitin).
Preparation of chitosan gel
About 5 g of chitosan was slowly added to 100 ml of 10% (w/ v) oxalic acid with constant stirring. The mixture was also heated to 40-50°C to facilitate mixing. A chitosan-oxalic acid mixture was formed with a whitish viscous gel.
Modification of biosorbent
The chitosan gel (100 ml) was diluted with water (~500 ml) and heated to 40 - 50°C. Ten grammes (10 g) of the sample of the adsorbent was slowly added to the diluted gel in separate container, and mechanically agitated using a shaker at 200 osc/min for 24 h. The gel coated adsorbents were then washed with distilled water and dried. It was then extensively rinsed with distilled water and dried in an oven at 102°C for 2 h, cooled at room temperature and stored in a desiccator.
Column biosorption experiment
Column biosorption study of the dye was carried out using the modified biosorbent in a column of 1.27 cm diameter and height of 40 cm, with simulated dye solutions of different initial concentrations (5, 25, 50 and 100 mg /L). Other parameters optimized include biosorbent dosage (0.1, 0.2, 0.3, 0.4 and 0.5 g) and contact time (10, 20, 30, 40, 50 and 60 min). For each of the investigations, the column was first loaded with the chitosan coated fungal biomass (biosorbent) and gravel in the ratio of 1 to 5 to create pressure for a better biosorption process. The simulated dye solution was later poured into the column setup in a down-flow direction. The effluents from the columns were then collected and residual dye concentrations determined with a UV/Visible spectrophotometer. The optimized conditions obtained from the simulated biosorption experiment were used to run the column biosorption of the textile industry waste water whose physicochemical properties have been determined using standard methods (Ademoroti, 1996; APHA, 2012) and heavy metal concentrations determined with Atomic Absorption Spectrophotometer ( APHA, 2012).
Adsorption isotherms
Dye was sorbed from various initial concentrations (5 to 100 mg/L) of simulated dye solution. Langmuir and Freundlich models were applied to the adsorption isotherm and relevant constants were generated (Langmuir, 1916; Freundlich, 1906).
Statistical analysis
The various data obtained before and after treatment with chitosan coated fungal biomass of A. niger were analyzed using one way ANOVA to test if there are significant differences between the initial dye concentration before treatment and after treatment with the adsorbent in both simulated dye solution and industrial textile wastewater. Student T-test was used to check for significant differences between the initial concentration before treatment and final concentration after treatment of the physico-chemical parameters in the textile wastewater.
Sample characterization
The FTIR spectrum of the chitosan powder (Figure 1) showed a broad band at 3383 cm-1due to O-H (hydroxyl) and N-H (amine) stretching. The peak at 1630 cm-1 was assigned to C=O stretch. In addition to the peak at 1382 cm-1 assigned to amide Ι, there are also peaks at 1476 and 1353 cm-1characteristic of chitin and chitosan which have been reported as amide I and III bands, respectively (Dragan et al., 2010).
Effect of initial concentration on the amount of dye adsorbed
Table 1 shows the effect of initial dye concentrations on the amount of dye adsorbed when the biosorbent was used for the treatment of both simulated dye solution and textile industry wastewater. As the initial concentration of the dye solution increased, the amount of dye uptake also increased in the simulated dye solution. This increase in the amount of dye adsorbed per unit mass of the adsorbent (Qe) with increase in initial dye concentration of the simulated wastewater is due to an increased driving force between the aqueous and solid phases thereby increasing the number of collisions between dye molecules and the adsorbent (Hubbe et al., 2011). In the textile wastewater, more dye uptake was observed than in the simulated dye solution. This is in agreement with Mohammed et al. (2009) who reported that in the application of adsorption for purification of wastewaters the solution will normally be a mixture of many compounds rather than a single one. The interactions of these compounds may mutually enhance adsorption.
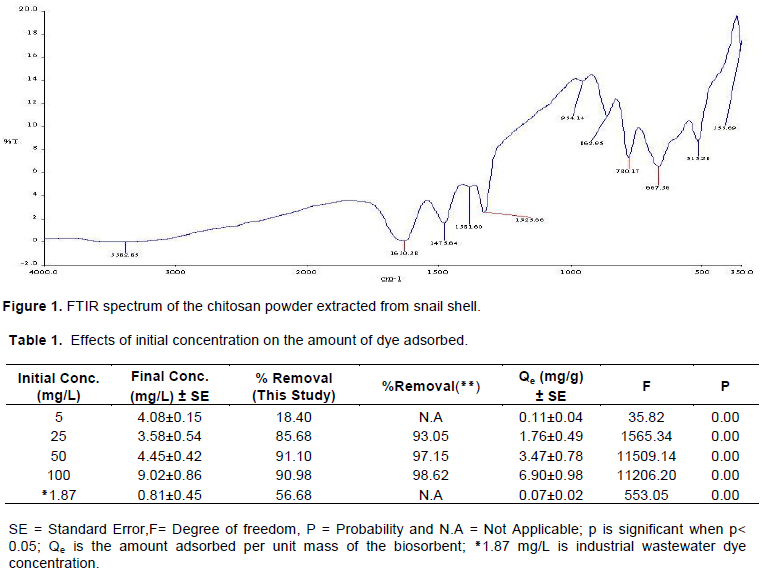
Effect of biosorbent dosage on the amount of dye adsorbed
Effect of biosorbent dosage on biosorption of dye was studied by varying biosorbent dosage from 0.1 to 0.5 g when other parameters like initial dye concentration were kept constant. Tables 2 and 3 show increase in dye uptake by the biosorbent. This is because of the availability of more binding sites in the surface of the biosorbent as the dosage increased (Asubiojo et al., 2009; Choi et al., 2009; Okoya et al., 2020a) until the surface was saturated leading to a decrease in the dye uptake by the biosorbent. Even though there were increases in the amounts of dye adsorbed with increase in biosorbent in the simulated dye solution and industrial wastewater, statistically there were no significant differences in the increases observed. This might be due to the fact that there is no commensurate increase in the adsorption resulting from lower adsorption of indigo dye per unit mass of adsorbent as reported by Etim et al. (2016).
Effect of contact time on biosorption of dye
Tables 4 and 5 show the uptake of dye as a function of contact time from both simulated dye solution and textile wastewater. The trend observed may be due to a very high continuous transfer rate of the dye to the surface of the biosorbent due to the availability of a large number of vacant sites initially for adsorption; later the adsorption capacity tailed off due to the saturation of vacant sites.
Adsorption isotherm
The applicability of sorption processes as a unit operation can be evaluated using isotherm models. The equilibrium sorption data obtained were analysed in terms of the Langmuir and Freundlich equations. The coefficients of these isotherms for different systems are usually determined by experimental analysis (Enemose et al., 2014). Table 6 shows the co-efficient of the isotherm for both the simulated dye solution and textile industrial wastewaters. The coefficient of correlation (R2) for both model falls within 0< R2< 1. This indicates that both models adequately describe the experimental data of the adsorption of dye studied. Freundlich isotherm had a better fitting for both simulated dye solution and the textile industrial wastewater.
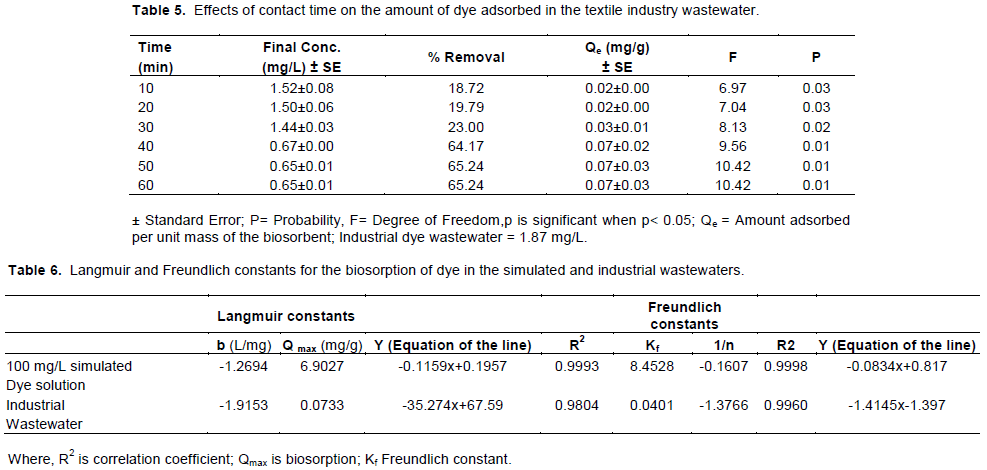
In this study, Langmuir adsorption constant was both negative in the simulated dye solution and textile industrial wastewater; while the
Freundlich isotherm model was positive in the simulated dye and industrial textile wastewater. This indicates that the amount of dye adsorbed when saturation is attained is higher with a strong electrostatic force of attraction when qmax is greater than unity (Alkan et al., 2008); but the reverse was the case in the industrial wastewater with a low Freundlich constant of 0.040 mg/g. The value of 1/n from Freundlich isotherm model indicates a constant related to intensity of adsorption. The 1/n from Table 6 shows a value that is less than a unit (1/n < 1) in both simulated and industrial wastewaters. A smaller value of 1/n indicates better adsorption mechanism and formation of a relatively stronger bond between adsorbate and adsorbent (Tellan et al., 2007).
Effect of biosorbent treatment on selected physico-chemical parameters and heavy metals in textile wastewater
The levels of heavy metals in the textile wastewater were quite higher than the tolerable limits set by Standard Organization of Nigeria (SON, 2007) which could have been possible through the various processes involved in textile producing factories as reported by Ohioma et al. (2009). Huang et al. (1988) reported that the uptake of metals with biological materials may be possible due to physical sorption or the nature of the biosorbent by being heterogeneous as with this adsorbent. Chromium ion was 0.131±0.000 mg/L before treatment as against 0.050 mg/L permissible limit set by Standard Organisation of Nigeria (SON, 2007) but it was reduced to 0.070±0.012 mg/L after treatment with the biosorbent. The 46% removal of Cr metal ion recorded in this work from the textile industrial wastewater supports the finding of Chojnacka (2005), which investigated the sorption of Cr (III) ions with hen eggshells from aqueous solutions and postulated the remediation potential of wastewaters containing heavy metals with agricultural by-products as biosorbent. Odoemelam et al. (2009) removed about 90% of Pb (II) ions from aqueous solution with oyster and giant snail shells reported the possibility of using these materials to remediate wastewaters contaminated with heavy metals. This work supports his findings with a removal of about 40% Pb metal ion from textile industry wastewater. Cadmium metal ion in the textile wastewater was reduced by 49% which agrees with the report of Mapolelo and Torto (2004) that proved the biosorption of Cd (II), Cr (III), Cr (VI), Cu (II), Pb (II) and Zn (II) with Saccharomyces cerevisiaeis optimum when the pH value is above 5. The textile wastewater contained 0.255±0.000 mg/L of zinc metal ion before treatment with the biosorbent which was reduced to 0.171±0.021 mg/L after treatment. This is about 33 % removal of Zn metal ion from the textile wastewater which is still in accordance with the report of Mapolelo and Torto (2004)) as sited previously. Cobalt metal ion, copper (Cu) and iron (Fe) were reduced by about 44, 42and 20% respectively. Magnesium (Mg) was 0.281±0.000 mg/L before treatment and 0.191±0.004 mg/L after treatment with the adsorbent while Manganese (Mn) was 0.043±0.000 mg/L before treatment and 0.024±0.004 mg/L after treatment with the adsorbent. Nickel (Ni) was 0.081±0.000 mg/L before treatment and 0.053±0.011mg/L after treatment with the adsorbent.
The conductivity of the wastewater increased from 342±1.000 µS/cm to 503.000±1.000 µS/cm after treatment with the biosorbent which could be as a result of the presence of salts which were used to cultivate A. niger. Total dissolved solids (TDS) was increased after treatment of the wastewater with the biosorbent, as against reduced TDS reported by Ramamurthy et al. (2011) in which live cultured isolate of A. niger was used. The increase in TDS observed in this work may be due to the use of dead A. niger fungal biomass. Alkalinity increased while acidity reduced from 100.000±3.50 to 38.500±1.600 mg/L, which is about 61.50% reduction. This may also be due to the alkaline nature as a result of the hydroxyl group present in the biosorbent used as evidenced in the FTIR spectrum of the chitosan used. Turbidity of the wastewater increased, which may be due to the dissolution of soluble organic compounds and other materials from the biosorbent into the wastewater as presented in Table 7.
The authors have not declared any conflict of interests.
The authors appreciate SwWECh laboratory, Institute of Ecology and Environmental Studies and Microbiology Department, Obafemi Awolowo University for granting access to the laboratory and assisting in the collection of Aspergillus niger isolates for the research.
REFERENCES
Ademoroti CMA (1996). Standard methods for water and effluents analysis. Nigeria: Foludex Press Limited 3:29-118.
|
|
Ahalya N, Kanamadi R, Ramachandra T (2005). Biosorption of chromium (VI) from aqueous solutions by the husk of bengal gram (cicer arientinum). Electronic Journal of Biotechnology 8(3):10-15.
Crossref
|
|
|
Alkan M, DoÄŸan M, Turhan Y, DemirbaÅŸ Ö, Turan P (2008). Adsorption kinetics and mechanism of maxilon blue 5G dye on sepiolite from aqueous solutions. Chemical Engineering Journal 139(2):213-223.
Crossref
|
|
|
American Public Health Association (APHA) (2012). Standard methods for examination of water and wastewater, method 9221 B.
|
|
|
Amuda OS, Giwa A, Bello IA (2007). Removal of heavy metal from industrial wastewater using modified activated coconut shell carbon. Biochemical Engineering Journal 36(2):174-181.
Crossref
|
|
|
Asubiojo OI, Ajelabi OB. (2009). Removal of heavy metals from industrial wastewaters using natural adsorbents. Toxicological & Environmental Chemistry 91(5):883-890.
Crossref
|
|
|
Ayangbenro AS, Babalola OO (2017). A new strategy for heavy metal polluted environments: a review of microbial biosorbents. International journal of environmental research and public health, 14(1):94.
Crossref
|
|
|
Baig S, Liechti PA (2001). Ozone treatment for bio-refractory COD removal. Water Science and Technology 43(2):197-204.
Crossref
|
|
|
Carolin CF, Kumar PS, Saravanan A, Joshiba GA, Naushad M (2017). Efficient techniques for the removal of toxic heavy metals from aquatic environment: A review. Journal of Environmental Chemical Engineering 5(3):2782-2799.
Crossref
|
|
|
Choi HD, Cho JM, Baek K, Yang JS, Lee JY (2009). Influence of cationic surfactant on adsorption of Cr (VI) onto activated carbon. Journal of hazardous materials 161(2-3):1565-1568.
Crossref
|
|
|
Chojnacka K (2005). Biosorption of Cr (III) ions by eggshells. Journal of Hazardous Materials 121(1-3):167-173.
Crossref
|
|
|
Ciardelli G, Capannelli G, Bottino A (2001). Ozone treatment of textile wastewaters for reuse. Water Science and technology 44(5):61-67.
Crossref
|
|
|
del Rocío Urbina-Salazar A, Inca-Torres, AR, Falcón-García G, Carbonero-Aguilar P, Rodríguez-Morgado B, del Campo JA, Bautista J (2019). Chitinase Production by Trichoderma harzianum Grown on a Chitin-Rich Mushroom Byproduct Formulated Medium. Waste and Biomass Valorization 10(10):2915-2923.
Crossref
|
|
|
Di Piazza SG, Cecchi AM, Cardinale C, Carbone MG, Mariotti MG Zotti M (2017). Penicillium expansum link strain for a biometallurgical method to recover rees from weee. Waste Management 60(2):596-600.
Crossref
|
|
|
Dragan ES, Dinu MV, Timpu D (2010). Preparation and characterization of novel composites based on chitosan and clinoptilolite with enhanced adsorption properties for Cu2+. Bioresource technology 101(2):812-817.
Crossref
|
|
|
Enemose EA, Osakwe SA (2014). Studies on the effect of pH on the sorption of Al 3 and Cr 6 Ions from aqueous solutions by Almond Tree (Terminalia catappa L.) Biomass. Research Journal of Chemical Sciences.
|
|
|
Etim UJ, AUmoren S, Eduok UM (2016). Coconut coir dust as a low cost adsorbent for the removal of cationic dye from aqueous solution, Journal of Saudi Chemical Society.
Crossref
|
|
|
Fersi C, Gzara L, Dhahbi M (2005). Treatment of textile effluents by membrane technologies. Desalination, 185(1-3): 399-409.
Crossref
|
|
|
Freundlich HMF (1906). Over the adsorption in solution. Journal of Physical Chemistry. 57(385471):1100-1107.
|
|
|
Guendouz S, Khellaf N, Djelal H, Ouchefoun M (2016) Simultaneous biosorption of the two synthetic dyes direct red 89 and reactive green 12 using nonliving macrophyte, L. gibba L. Desalination and Water Treatment Processes 57:4624-4632.
|
|
|
Huang CP, Westman D, Quirk K, Huang JP (1988). The removal of cadmium (II) from dilute aqueous solutions by fungal adsorbent. Water Science and Technology 20(11-12):369-376.
Crossref
|
|
|
Hubbe MA, Hasan SH, Ducoste JJ (2011). Cellulosic substrates for removal of pollutants from aqueous systems: A review. 1. Metals. Bio Resources 6(2):2161-2287.
Crossref
|
|
|
Iqbal MAS, Akhtar N (2002). Petiolar felt-sheath of palm: A new biosorbent for the removal of heavy metals from contaminated water. Bioresource Technology 81(2):151-153.
Crossref
|
|
|
Joshi NC (2018). Biosorption: Agreen approach for heavy metals removal from water and waste waters. Research Journal of Life Science Bioinformatics, Pharmaceutical and Chemical Sciences 4(1):1-59.
|
|
|
Jaishankar M, Tseten T, Anbalagan N, Mathew BB, Beeregowda KN (2014). Toxicity, Mechanism and Health effects of some Heavy Metals. Interdisciplinary Toxicology 7(2): 60-72.
Crossref
|
|
|
Khan N, Ryu KY, Choi JY, Nho EY, Habte G, Choi H , Kim MH, Park KS, Kim KS (2015). Determination of toxic heavy metals and speciation of arsenic in seaweeds from South Korea. Journal of food Chemistry. 169:464-470.
Crossref
|
|
|
Langmuir I (1916). The constitution and fundamental properties of solids and liquids. Part I. Solids. Journal of the American chemical society 38(11):2221-2295.
Crossref
|
|
|
Leonas KK, Leonas ML (1994). Textile process wastewater permits: an update and strategies. American Dyestuff Reporter 83(3):26-35.
|
|
|
Malik A (2004). Metal bioremediation through growing cells. Environment international 30(2):261-278.
Crossref
|
|
|
Mapolelo M, Torto N (2004). Trace enrichment of metal ions in aquatic environments by Saccharomyces cerevisiae. Talanta 64(1):39-47.
Crossref
|
|
|
Marmagne O, Coste C (1996). Colour removal from textile plant effluents. American Dyestuff Reporter 85(4):15-21.
|
|
|
Mohammed WT, Farhood, HF, Al-Mas' udi, AHB. (2009). Removal of Dyes from Wastewater of Textile Industries Using Activated Carbon and Activated Alumina. Iraqi Journal of Chemical and Petroleum Engineering 10(1):43-52.
|
|
|
Muhammad N, Jeremy P, Michael P, Smith D, Wheatley A (1998). Sanitation and water for all, Paper presented at the 24th WEDC Conference, Islamabad, Pakistan, Islamabad, Pakistan.
|
|
|
Odoemelam SA, Eddy NO (2009). Studies on the Use of Oyster, Snail and Periwinkle Shells as Adsorbents for the Removal of Pb2+ aqueous solution. Journal of Chemistry 6(1):213-222.
Crossref
|
|
|
Ohioma AI, Luke NO, Amraibure O (2009). Studies on the pollution potential of wastewater from textile processing factories in Kaduna, Nigeria. Journal of Toxicology/Environmental Health Science 1(2):034-037.
|
|
|
Ojima Y, Kosako S, Kihara M, Miyoshi N, Igarashi K, Azuma M (2019). Recovering metals from aqueous solutions by biosorption onto phosphorylated dry baker's yeast. Scientific reports 9(1):1-9.
Crossref
|
|
|
Okoya AA, Akinyele AB, Ofoezie IE, Amuda OS, Alayande OS, Makinde, OW (2014). Adsorption of heavy metal ions onto chitosan grafted cocoa husk char. African Journal of Pure and Applied Chemistry 8(10):147-161.
Crossref
|
|
|
Okoya AA, Akinyele AB, Amuda OS, Ofoezie IE (2015). Chitosan grafted modified maize cob for removal of lead and chromium from wastewater. Ethiopian Journal of Environmental Studies and Management 8(2):881-892.
Crossref
|
|
|
Okoya AA, Adegbaju OS, Akinola OE, Akinyele AB, Amuda OS (2020a). Comparative assessment of the efficiency of rice husk biochar and conventional water treatment method to remove chlorpyrifos from pesticide polluted water. Current Journal of Applied Science and Technology pp. 1-11.
Crossref
|
|
|
Okoya AA., Olaiya OO, Akinyele AB, Ochor NO (2020b). Efficacy of Moringa oleifera Seed Husk as Adsorptive Agent for Trihalomethanes from a Water Treatment Plant in Southwestern, Nigeria. Journal of Chemistry 2020:1-11.
Crossref
|
|
|
Quek S, Wase D, Forster C (1998). The use of sago waste for the sorption of lead and copper. Water SA 24(3):251-256. B15 2TT.
|
|
|
Ramamurthy N, Balasaraswathy S, Sivasakthivelan P (2011). Biodegradation and physico-chemical changes of textile effluent by various fungal species. Romanian Journal of Biophysical 21(2):113-123.
|
|
|
Rao L, Prabhakar G (2011). Removal of heavy metals by biosorption-an overall review. Journal of England Research Studies.
|
|
|
SON (2007). Nigerian standards for drinking water quality (NSDWQ), Industrial standards. SON, 554, 1-14,2007.
|
|
|
Tellan AC, Owalude SO (2007). Some Langmuir and Freundlich parameters of adsorption studies of chlorpheniramine maleate. Research Journal of Applied Sciences 2(8):875-878. ISSN: 1815-932X.
|
|