ABSTRACT
Diffusion coefficients of biopolymers extracted from Grewia spp. barks in water were studied under various physicochemical conditions. Extractions were carried out on barks of 3 cm in length at different pH (5-9), temperature (25-50°C) and ionic strength (10-3-10-2M), using CaCl2 and NaCl as background electrolytes. Factorial experimental plan design was used and diffusion coefficients were determined using second law of Fick equation, with linear and logarithmic approximation. Obtained results show that diffusion coefficients of Grewia spp. biopolymers are in the range of 9.78458E-06±1.6462E-08 cm2/min, and are not significantly influenced¶not soignificantly influence by the pH, the ionic strength and the temperature. Maximum diffusion was obtained with CaCl2 at high ionic strength. Both linear and logarithmic approximations were found to be suitable for diffusion coefficients and were dependent on the background electrolyte used.
Key words: Biopolymers, diffusion coefficient, Grewia.
Different plants extracts have been used or tested as biopolymers for coagulation; Moringa oleifera, Strychnos potatorum, Jatropha curcas sabdariffa, Curcuma angustifolia (Pritchard et al., 2010), Phaseolus vulgaris and Grewia. Grewia spp. is a flowering plant from the family of tiliacées that grows in the shade of nearby rocks and reeds, native to warm tropical regions of Africa, Asia and Australia (Emeje et al., 2008). It is used as a thickener, stabilizer in food process and in some cases it may act as a coagulant /floculant (Ndi et al., 2013).
In water clarification processes, studies on efficient biopolymers for coagulation purposes are widespread due to the environmental impact of those coagulants (Chun-Yang, 2010). Biopolymers can be extracted from different parts of the plant including barks, leaves and roots but, when powders are used, fine particles are released, increasing the suspended material and the turbidity of the suspension, and may act as a support for bacterial growth (Kebreab et al., 2005). Therefore, purification of extracted biopolymers is often required. Unfortunately, these methods include a combination of lyophilisation, chromatography and dialysis, which are complex and expensive for implementation in rural areas of developing countries (Kebreab et al., 2005). Thus, looking for an optimum in the extraction of biopolymers with fewer amounts of fines released is of great importance for coagulation purposes. Using barks instead of powders as coagulant/flocculant may increase the settling velocity of flocs, reducing considerably the amount of fines released in the suspension, and further accelerate the clarification step.
During flocculation, the required amount of coagulant or flocculant for good clarification purpose is directly linked to the initial water quality. Thus, knowledge of biopolymer concentration in the suspension is of great importance for coagulation process. When barks are soaked in water, biopolymers are released from the bark to the sus-pension. This extraction process can be improved by agitation of the suspension, but in a house hold scale it is generally done without any agitation, as it is easily carried out, leading to natural diffusion of biopolymers from the bark to the suspension. As natural diffusion is a mass transfer process, induced by gradient concentration, there’s need to evaluate the amount of biopolymers released within the contact period time of the bark in the liquid. This can be done knowing diffusion coefficients.
Diffusion coefficient is a key parameter of mass transfer process and describes how fast the amount of released biopolymers in the suspension is. Diffusion coefficient although generally assumed as constant depends on many parameters like ionic strength, pH, temperature and bark concentration (Giovana et al., 2011).
Diffusion coefficients are well determined by direct methods including technique of fluorescence recovery after photo bleaching (FRAP) which has been refined through the use of a laser scanning confocal microscope (LSCM) or by indirect methods like spectroscopy, chromatography and mass balance in the system (Matthew et al., 2000). When indirect methods are used, plotting the amount of biopolymer released as a function of the concentration as described by the second law of Fick equation gives the diffusion coefficient. Approximation of the Fick equation commonly used is linear and logarithmic ones which were found to be suitable for biopolymers diffusion in water system. The linear model corresponds to the earlier part of the slope of the diffusion process while logarithmic is mainly used when the required time for extraction is high (Poirot, 2007).
The aim of this study is therefore to evaluate diffusion coefficients of Grewia spp. biopolymers and to find suitable models describing it under different physicochemical conditions.
Biological material
Grewia spp. was collected from Mokolo, a town located in the far Northern region of Cameroon. Grewia spp. barks of 3 cm length were used as biological material for biopolymer extraction. They were dried at 45°C ¶ausing a forced convection dryer (CKA2000AUF) for 24 h, allowed to cool in a desiccator, and kept in sealed plastic bags prior to their use. The thickness (L) of Grewia spp. bark used was obtained by measuring the average size of 100 barks selected randomly.
Characterization
FTIR analyses were carried out before and after biopolymer extraction.
Polymer extraction
Polymer extraction was carried out in batch mode without any mechanical stirring of the solution, with distilled water of pH (5-9) adjusted using 1 M hydrochloric acid (Sherman Chemicals limited) or 1 M NaOH solutions (Prolabo), and ionic strength (0-0.01 M) adjusted using NaCl (Jeulin) and CaCl2 (Jeulin) salts. Conductivities were monitored using conductimeter (HI 8733, HANNA Instrument) and biopolymer mass was determined after each extraction by drying in an oven (Memmert) at 105°C and weighing the resulting extract on a mass balance of 10-3 precision (Gibertini).
Extraction kinetics were done with a ratio of barks mass (5, 10 and 20 g) over extraction solvent volume of 250 mL.
Experimental design
Complete factorial design was used. Selected factors are presented in Table 1. The experimental matrix is described in Table 2, and the diffusion coefficient was defined as the response.
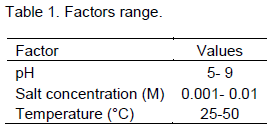
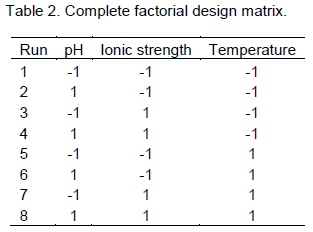
Diffusion coefficients determination
Diffusion coefficients were derived from the slopes of the following equations (Aghfir et al., 2008; Claudia et al., 2009). Taking into consideration the hypothesis that:
1. The particles have the shape of a plate;
2. Uniform initial distribution of moisture;
3. Simplification of the movement of moisture by diffusion;
4. Negligible contracting;
5. Constant coefficient of diffusion.
The diffusion coefficient can be calculated by using the following equations for the two models:
Where Mt is the mass of polymers at the moment t, M∞ is the mass of polymers at infinite time, L is the thickness of the barks, t the time and the multidirectional diffusion coefficient. The obtained quadratic equation was expressed as:
Diffusion coefficient = CST + CA*A + CB*B + CC*C+ CAB *A*B + CAC*A*C - CBC *B*C
Where, CX denotes the coefficient factor related to the factor X studied.
Characterization of Grewia spp.
The FT-IR spectroscopic spectra of the powders of Grewia unextracted and extracted are presented in the Figures 1 and 2, respectively. The main difference between these two spectra is at the level of the intensities of the peaks.
The spectra exhibit the typical bands and peak characteristic of polysaccharides. The broad band occurring at 3272.60 cm-1, results from the presence of hydroxyl (-OH) group. The peak obtained at 2927.68 cm-1 results from stretching modes of the C-H bonds of methyl group (-CH3). Natural gum usually contains fractions of sugar acid units which would usually impart a weakly anionic character to the gum macromolecule (Wang et al., 2003).
Absorption bands around 1618 and 1430 cm-1 are typical of carboxylate group of the galacturonic acid residues (Figueiro et al., 2004). The peak obtained at 1519.07 cm-1 is typical of carboxylic group. The peak obtained at 1074.97cm-1 result from the presence C-O-C group. The peaks obtained at 1720.23 and 1607.16 cm-1 results from the presence of C=O group. The wave numbers between 800 and 1200 cm-1 represents the finger print region for carbohydrates (Fillipove, 1992).
Physicochemical effects on released Grewia spp. biopolymers
Figure 3 presents the effect of bark mass/water solution ratio (5/250, 10/250 and 20/250) on biopolymer extraction over time on the ratio of biopolymer mass (EP) recovered over the initial mass of the bark introduced (BM). As shown, less than 4%
of the bark mass is released in the suspension during batch operations whatever the ratio. From 0 to 60 min, rapid transfer of biopolymer from the bark to the suspension is observed. Further, the biopolymer is still recovered, but at a low rate. When 20 g of bark is introduced in 250 mL of suspension, the amount of polymer recovered slightly decreases. Polymer extraction in dilute solutions is coherent with other works related to polymer transfer. Reduction of the amount of polymer when bark ratio increases is due to the well-known resistance of transport due to the gradient of concentration in the solution which becomes low and then reduces the transfer of the polymer inside the suspension.
Modeling diffusion coefficients of Grewia spp.
Using complete factorial design followed with linear and logarithmic approximation, coefficients of factors involved in the extraction process and quadratic interactions are presented in Table 3 for diffusion with NaCl salt and in Table 4 for diffusion with CaCl2 salt.
The obtained quadratic equation with NaCl was expressed as:
Linear model
Diffusion coefficient = 1.02E-5- 2.93E-8*A+1.19E-6*B+3.99E-6*C+ 2.98E-8*A*B + 1.65E-7*A*C + 4.44E-7*B*C
Logarithmic model
Diffusion coefficient = 1.36E-4+6.61E-7*A+1.26E-5*B+5.09E-5*C-3.31E-6*A*B+3.31E-6*A*C+1.98E-6*B*C
The obtained quadratic equation with CaCl2 was expressed as:
Linear model
Coefficient diffusion = 1.07E-05+3.62E-7*A+2.27E-6*B+3.52E-6*C+1.65E-7*A*B+1.96E-7*A*C- 8.78E-8*B*C
Logarithmic model
Coefficient de diffusion = 1.43E-4+3.31E-6*A+9.92E-6*B+4.69E-5*C+4.63E-6*A*B-3.31E-6*A*C+ 6.61E-7*B*C
Tables 3 and 4 present the coefficients of parameters involved in the quadratic equation resulting from the factorial design plan. The observed probability of all the factors was found to be greater than 0.05, showing that at a probability of 95%, these factors did not have a great influence on the diffusion coefficient.
Table 5 presents the values of statistic parameter used to validate the model. Since the values obtained R2, AADM, Bf is near the values expected, the mathematical model obtained is considered as valid.
But by taking into account the parameters of validation of the model, one notes that the model logarithmic model
presents itself as an explanation of the interaction between the various factors of the extraction of polymers of Grewia in the presence of CaCl2, whereas the linear model presents itself rather better than the extraction in the presence of NaCl.
Figure 4 presents the pH effect on extraction ratio on polymer removal and extraction time on polymer mass recovered over the mass of the bark as shown for a ratio bark/volume equal to 10/250. At the beginning of the diffusion of polymers into the solution (from 0 to 60 min), less influence of pH on polymer transfer is observed. Net pH influence is found after 120 min of extraction, and the lowest extraction ratio is obtained at pH 7, while the highest one is obtained at pH 6.
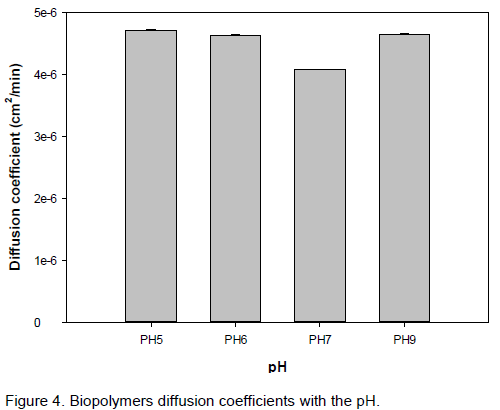
Plants polymers are mainly polysaccharides. They possess a high tendency to associate, caused by the abundance in hydroxyl or amino groups present in the macromolecules that easily undergo hydrogen bonding, and are very sensitive to pH variations. In acidic solutions, protonation of carboxylic and amino groups will increase electrostatic repulsion and solubilize the polymer, increasing its diffusivity (Nandhini and Abhilash, 2010; Lawrence et al., 2006). This behavior is also observed at high pH (9), but in this case, deprotonation is involved. At neutral pH 7, hydrogen bonds existing inside the polymers will increase the size of polymer which will result in a decrease of polymer diffusivity.
Figure 5 presents the effect of salts and salt concentration on polymer. Without any salt, the released amount of polymer is low (2.5 %) and increases with the type of salt and the concentration. As ionic strength increases, the solubility of the polymers increases as predicted by the salting in theory (Djamen, 2011). The solubilisation will induce a rapid diffusion of the polymer in the medium. In this particular case, traction is more pronounced with Ca than Na salts. These differences
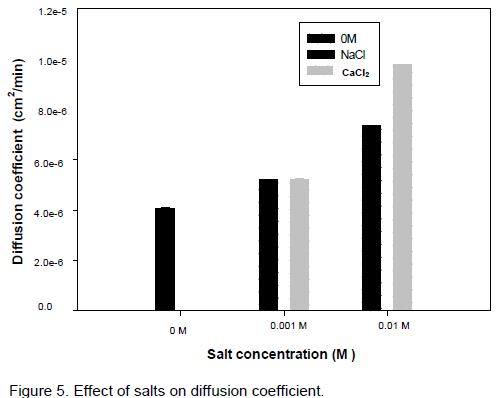
could be explained by the ionic conformation of the different salts used; Ca ions of greater ionic radius than Na ones release their solvation water more quickly, thus increasing the solubility of polymers and in fine the diffusion coefficient (Djamen, 2011).
From the above results it is obvious that physicochemical properties of the solution greatly affect the yield of polymer extraction.
The principal objective of this work was to determine the diffusion coefficient of polymers of Grewia spp. under various operating conditions. The results show that the polymers of Grewia spp. diffuse more quickly in the salt solutions and is effective at high temperatures than in distilled water; with diffusion coefficients of 9,78458e-06±1,646e-08, 7,3456e-06±1,746e-08 and 4,07001e-06±1,55077e-08 cm2/min, respectively with CaCl2, NaCl and distilled water. These results also show that the diffusion coefficients of Grewia spp. polymers in the presence of CaCl2 are higher than those obtained in the presence of NaCl, and that it is not preferable to extract polymers from Grewia spp. with neutral pH. ¶According to the type of salt, one can make use of either the linear model or the model logarithmic curve for the expression of the interaction between the parameters of extraction.
The authors have not declared any conflict of interests.
REFERENCES
Aghfir A, Akkad S, Rhazi M, Kane CSE (2008). Détermination du coefficient de diffusion et de l'énergie d'activation de la menthe lors d'un séchage conductif en régime continu. Revue des énergies renouvelables 11:3385-394.
|
|
Chun-Yang Y (2010). Emerging usage of plant-based coagulants for water and wastewater treatment. Process Biochem. 45:1437-1444.
Crossref
|
|
|
Claudia M, Rascon-Chu A, Jorge AE, Ana Luisa M (2009). Maize arabinoxylan gels as proteindelivery matrices. Molecules 14:1475-1482.
Crossref
|
|
|
Djamen MCN (2011). Etude structurale et rhéologique des systèmes mixtes caséinates/carraghénanes. Thèse de doctoratUniversité du Maine.
|
|
|
Emeje M, Isimi C, Kunle O (2008). Effect of Grewiagum on the mechanical properties of Paracetamol tablet formulations. Afr. J. Pharm. Pharmacol. 2(1):1-6.
|
|
|
Figueiro SD, Moreira RA, Sombra ASB (2004).On the physicochemical and dielectric properties of glutaraldehyde cross-linkelgalactomannan-collagen films. Carbohydr. Polym. 56:313-320.
Crossref
|
|
|
Fillipove MP (1992). Practical infrared spectroscopy of pectin substance.Food Hydrocoll. 6:115-142.
Crossref
|
|
|
Giovana D, Ferreira M, TessaroI C, Caciano PZ (2011). Evaluation of water, sucrose and NaCl effective diffusivities during osmotic dehydration of banana (Musa sapientum, shum.). LWT Food Sci. Technol. 44:82-91.
Crossref
|
|
|
Kebreab A, Ghebremichaela Gunaratnab K, Hongbin H, Harry B, Gunnel D (2005). A simple purification and activity assay of the coagulant protein from MoringaOleifera seed. ISRN KTH/LWR/PHD 1013-SE.
|
|
|
Lawrence AA, Mohammednur Desta S, Shiferaw M (2006). Effect of ionic strength and/or pH on Extractability and physico-functional characterization of broad bean (Vicia faba L.) protein concentrate. Food Hydrocoll. 20:1124-1134.
Crossref
|
|
|
Matthew D, Burke JO, Mohan S, Saad A (2000). Diffusion of Macromolecules in Polymer Solutions and Gels: A LaserScanning Confocal Microscopy Study.Macromolécules 33:7500-7507.
Crossref
|
|
|
Nandhini V, Abhilash M (2010). Study of hydration kinetics and rheological behaviour of guar gum. Int. J. Pharma Sci. Res. 1(1):28-39.
|
|
|
Ndi KS, Ngnie NP, Kofa GP, Amba EV, Kayem GJ (2013). Optimization of polymer extraction from Grewia spp. Plant. SFGP2013 - XIVe congrès de la Société Française de Génie des Procédés.
|
|
|
Poirot R (2007). Méthodologie pour le passage en continu d'extraction de soluté à partir de matière végétale. National Polytechnique de Toulouse école doctorale: mécanique, énergétique, génie civil, procédés spécialité: génie des procédés et environnement.
|
|
|
Pritchard A, Craven T, Mkandawire B, Edmondson A (2010). A study of the parameters affecting the effectiveness of Moringa Oleifera in drinking water purification. Phys. Chem. Earth 35: 791–797.
Crossref
|
|
|
Wang O, Ellis PR, Ross–Murphy SB (2003). Dissolution kinetics of guar gum powders: effect of concentration and molecular weight. Caborhydr. Polym. 53:75-83.
Crossref
|
|