The aim of the present study was to assess the antipyretic activity of the macrolide antibiotic, tilmicosin, at dose levels of 20 and 40 mg/kg of body weight, subcutaneously, in Brewer's yeast-induced fever model in mice. Pyrexia was induced by subcutaneous injection of 20 mL/kg of 20% (w/v) Brewer’s yeast suspension into the animal’s scruff region. Eighteen hours later, feverish animals were treated with either tilmicosin or acetylsalicylic acid (200 mg/kg injectable solution, subcutaneously) or vehicle; and rectal temperatures were evaluated at 1, 2, 3, 4 and 5 h post-treatment using digital thermometers. Tilmicosin showed dose-dependent significant decrease in the elevated body temperature of mice that remained sustained throughout the tested time points from 1 to 5 h in the used model. Both small and large dose levels showed a significant inhibition of elevated body temperature when compared with the corresponding febrile controls (37.65 ± 0.04 vs. 38.41 ± 0.08°C and 37.44 ± 0.04 vs. 38.44 ± 0.04, after 1 h; 37.19 ± 0.04 vs. 38.41 ± 0.08°C and 36.80 ± 0.03 vs. 38.44 ± 0.04°C after 5 h, respectively). These activities were standardized as 38.0 and 51.59% and 47.9 and 66.43% after 5 h, respectively, compared to that of the standard antipyretic and acetylsalicylic acid (200 mg/kg of body weight, subcutaneously). These results may indicate that tilmicosin, in addition to its well established antibacterial activity, possesses significant antipyretic activity that may be beneficial in symptomatic relief when it is used in therapy of infectious disease conditions and inflammatory disorders.
Fever is a well-known finding in almost all of infectious disease conditions and inflammatory disorders. Although uncomfortable, or even risky if 4 degrees over normal because of dehydration, strained heart and impaired respiration; however, it gives an alarm of warning of infection or a risk to the body. Mastering of fever besides treating the specific infectious agent that causes the disease is considered as a critical and important issue in therapy for safety and welfare of animal and human patient subjects. Classically, the genesis of fever or pyrexia; upon exposure to infectious agents as bacteria, viruses, fungi and some parasites or to mechanical injuries; is induced by inflammatory mediators (that is, prostaglandins and pro-inflammatory cytokines) that are released by affected tissue and activated immune cells (
Ahrens, 1996;
Roth, 2006). Within the brain, prostaglandin E2 (PGE2), produced by cyclooxygenase (COX)-2, is regarded as the principal mediator of fever (
Aronoff and Neilson, 2001)acting on thermosensitive or thermointegrative hypothalamic neurons. Brewer’s yeast-induced pyrexia is the most common model for investigating the antipyretic potentials of unknown substances. It is an eukaryote and belongs to the fungus
Saccharomyces cerevisiae that is single cellular spherical or ellipsoidal in shape. The yeast-induced fever (pathogenic fever) in mouse model was employed in the present study, to investigate the antipyretic activity of tilmicosin. An effective antipyretic is a drug or agent that can reduce fever or elevated body temperature. The word comes from the Ancient Greek (
anti, against) and (
pyreticus, pertaining to fever) (
Merriam-Webster, 2014).
Antipyretics may interrupt pyrexogenesis at any step from periphery to heat regulating centres. Many well-known and approved antipyretics are established including aspirin, paracetamol, ibuprofen, diclofenac, etc., are prescribed together with specific drugs in fevers associated with inflammatory conditions caused by infectious and/or traumatic agents. In addition to the standard and established common antipyretic agents, some other drugs may have antipyretic potentials besides their categorized main pharmacological actions and purposes. This finding may give the benefit of synergism when these potentially active drugs can be combined together with the standard ones; synergism may render, sometimes, therapy safer and more agreeable. Tilmicosin is a macrolide antibiotic with the chemical name of 20-deoxo-20-(3,5-dimethyl piperidin-1-yl) desmycosin. Macrolide class of antibiotics contain a macrocyclic lactone ring in their molecular structure; tilmicosin contains a 16-member one. The kinetic behaviour and properties of macrolide antimicrobials, that is, characterized by a distribution volume (Vd) allow to reach a high concentration in the target tissue (Biophase) even after administration of a small dose. Tilmicosin, among macrolide antimicrobials, is a bacteriostatic and works by penetrating the cell membrane of sensitive microbes and binding to the 50s ribosomal subunit, suppressing protein synthesis.
More precisely, the translocation of immature peptide chains between the 50 and 30s ribosomal subunits is interfered, this leads to early detachment and incomplete peptide chains are being synthesized (
Seiple et al., 2016). Tilmicosin is an effective remedy for various Gram-positive organisms such as
Corynebacterium and
Listeria species, some Gram-negative bacteria, such as
Pasteurella and
Haemophilus species, as well as atypical bacteria as
Mycoplasma species that infection by all of which is associated with fever. Tilmicosin is related to tylosin in its chemical structure, with the chemical formula of C
46H
80N
2O
13 and molecular weight of 869.15. From the physical point of view, it is freely soluble in organic solvents like hexane, acetone, acetonitrile, chloroform, dichloromethane, ethyl acetate, methanol, and tetrahydrofuran with solubility rate of 1500 mg/L or greater. Water solubility depends on temperature and pH; the solubility rate is about 566 mg/mL at pH 7 and 25°C. In a previous study, the antinociceptive potential of tilmicosin in mice have been proven against chemical but not thermal stimulai (
El-Mahmoudy and Gheith, 2016). The objective targeted in the current study was the assessment of the antipyretic activity of tilmicosin on Brewer’s yeast induced fever in mice.
Fever is a well-known finding in almost all of infectious disease conditions and inflammatory disorders. Although uncomfortable, or even risky if 4 degrees over normal because of dehydration, strained heart and impaired respiration; however, it gives an alarm of warning of infection or a risk to the body. Mastering of fever besides treating the specific infectious agent that causes the disease is considered as a critical and important issue in therapy for safety and welfare of animal and human patient subjects. Classically, the genesis of fever or pyrexia; upon exposure to infectious agents as bacteria, viruses, fungi and some parasites or to mechanical injuries; is induced by inflammatory mediators (that is, prostaglandins and pro-inflammatory cytokines) that are released by affected tissue and activated immune cells (
Ahrens, 1996;
Roth, 2006). Within the brain, prostaglandin E2 (PGE2), produced by cyclooxygenase (COX)-2, is regarded as the principal mediator of fever (
Aronoff and Neilson, 2001)acting on thermosensitive or thermointegrative hypothalamic neurons. Brewer’s yeast-induced pyrexia is the most common model for investigating the antipyretic potentials of unknown substances. It is an eukaryote and belongs to the fungus
Saccharomyces cerevisiae that is single cellular spherical or ellipsoidal in shape. The yeast-induced fever (pathogenic fever) in mouse model was employed in the present study, to investigate the antipyretic activity of tilmicosin. An effective antipyretic is a drug or agent that can reduce fever or elevated body temperature. The word comes from the Ancient Greek (
anti, against) and (
pyreticus, pertaining to fever) (
Merriam-Webster, 2014).
Antipyretics may interrupt pyrexogenesis at any step from periphery to heat regulating centres. Many well-known and approved antipyretics are established including aspirin, paracetamol, ibuprofen, diclofenac, etc., are prescribed together with specific drugs in fevers associated with inflammatory conditions caused by infectious and/or traumatic agents. In addition to the standard and established common antipyretic agents, some other drugs may have antipyretic potentials besides their categorized main pharmacological actions and purposes. This finding may give the benefit of synergism when these potentially active drugs can be combined together with the standard ones; synergism may render, sometimes, therapy safer and more agreeable. Tilmicosin is a macrolide antibiotic with the chemical name of 20-deoxo-20-(3,5-dimethyl piperidin-1-yl) desmycosin. Macrolide class of antibiotics contain a macrocyclic lactone ring in their molecular structure; tilmicosin contains a 16-member one. The kinetic behaviour and properties of macrolide antimicrobials, that is, characterized by a distribution volume (Vd) allow to reach a high concentration in the target tissue (Biophase) even after administration of a small dose. Tilmicosin, among macrolide antimicrobials, is a bacteriostatic and works by penetrating the cell membrane of sensitive microbes and binding to the 50s ribosomal subunit, suppressing protein synthesis.
More precisely, the translocation of immature peptide chains between the 50 and 30s ribosomal subunits is interfered, this leads to early detachment and incomplete peptide chains are being synthesized (
Seiple et al., 2016). Tilmicosin is an effective remedy for various Gram-positive organisms such as
Corynebacterium and
Listeria species, some Gram-negative bacteria, such as
Pasteurella and
Haemophilus species, as well as atypical bacteria as
Mycoplasma species that infection by all of which is associated with fever. Tilmicosin is related to tylosin in its chemical structure, with the chemical formula of C
46H
80N
2O
13 and molecular weight of 869.15. From the physical point of view, it is freely soluble in organic solvents like hexane, acetone, acetonitrile, chloroform, dichloromethane, ethyl acetate, methanol, and tetrahydrofuran with solubility rate of 1500 mg/L or greater. Water solubility depends on temperature and pH; the solubility rate is about 566 mg/mL at pH 7 and 25°C. In a previous study, the antinociceptive potential of tilmicosin in mice have been proven against chemical but not thermal stimulai (
El-Mahmoudy and Gheith, 2016). The objective targeted in the current study was the assessment of the antipyretic activity of tilmicosin on Brewer’s yeast induced fever in mice.
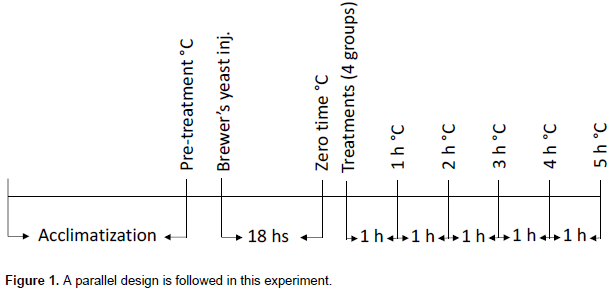
Brewer’s yeast-induced pyrexia model
The method described by
Tomazetti et al. (2005)was adopted with minor modifications to test the antipyretic activity of tilmicosin in mice. After recording the rectal temperature of each of the animal using a small sized digital thermometer digital thermometer (9SK-1250 MC, Sato Keiryoki Mfg Co. Ltd., Japan), pyrexia was induced by subcutaneous injection of 20 mL/kg of 20% (w/v) Brewer’s yeast (Sigma-Aldrich, St. Louis, USA) suspension into the animal’s scruff region. Animals were then fasted and 18 h later, the rectal temperature of each animal was re-measured and recorded. Only mice showing an increase in temperature of at least 1°C were used for experiment. Feverish animals were treated with either tilmicosin or standard or vehicle as described earlier; and rectal temperatures were measured at 1, 2, 3, 4 and 5 h post-treatment. Temperature readings of the second group at these different time points were compared to those of the first group; while the readings of the third, fourth and fifth groups were compared to those of the second group at the same time points. Percentage reduction in rectal temperature (antipyresis) was calculated using the following equation:
% Reduction = (B – Tn / B – Cn) × 100
where n is the time point = 1, 2, 3, 4 and 5; B is the Brewer’s feverish temperature; T is the treated temperature; C is the control temperature.
Data presentation and statistical analysis
All data are expressed as Mean ± standard error of mean (SEM) of eight observations (n = 8). Comparison among control and treated groups (small and large doses) were analysed for significance using a one-way analysis of variance (ANOVA) followed by least significant difference (LSD) test as a post-hoc. P-values of 0.05 or less were considered significant. The antipyretic potential of tilmicosin has been, in addition, standardized as % comparable to that of acetylsalicylic acid (standard antipyretic agent). All calculations and procedures of data statistical analysis were carried out using the computer programme SPSS v20 software.
Animals received tilmicosin 20 or 40 mg/kg of body weight exhibited localized tender swellings at the site of subcutaneous injection of both tested doses. Results of the adopted antipyretic test were recorded as the following. The results of the antipyretic assay of the test (tilmicosin, 20 and 40 mg/kg, SC) and standard (ASA, 200 mg/kg, SC) drugs, as well as those of the control are shown in Table 1 and depicted in Figure 2. Normal rectal temperatures of mice ranged between 36.43 and 36.55°C. All Brewer’s yeast suspension-injected animals became feverish after 18 h with rectal temperature range of 38.2 to 38.44°C. Vehicle-treated animals remained feverish until the end of the experimental period. On the other hand, ASA (200 mg/kg of body weight, subcutaneously) as well as tilmicosin (20 and 40 mg/kg of body weight, subcutaneously) have shown effective significant reduction of the elevated rectal body temperatures at all of the tested time points (1 to 5 h) post-administration as compared to the feverish control (P < 0.05; ANOVA followed by LSD test). Antipyresis percentage of tilmicosin and those of the standard ASA are shown in Table 2.
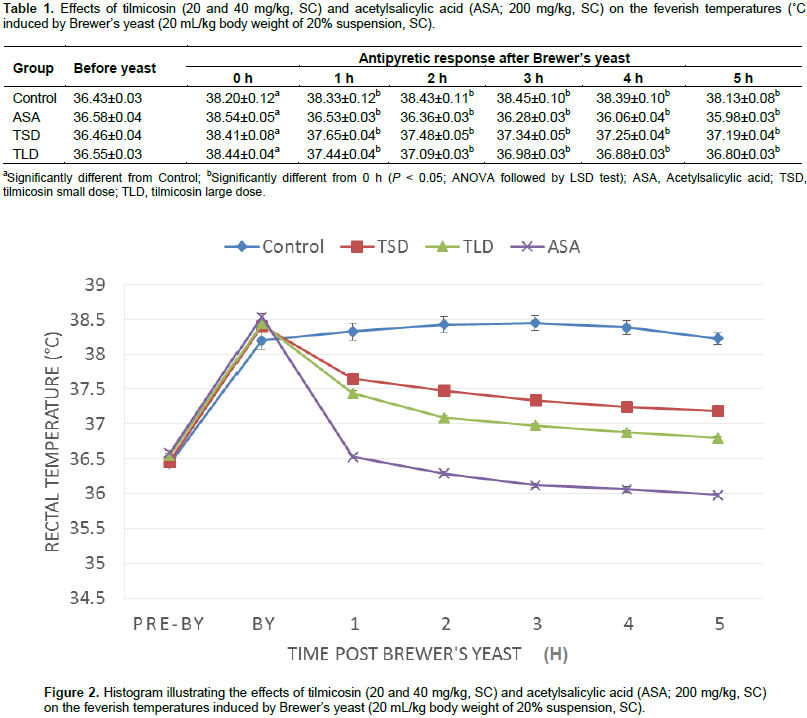
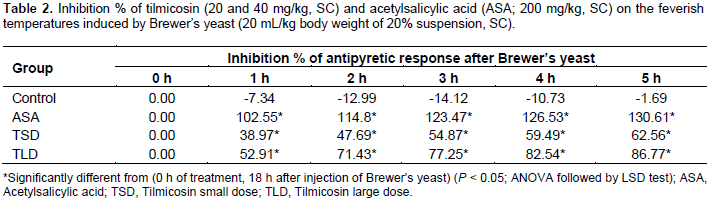
The chemotherapeutic group of macrolides has been long-used class of antimicrobials and still play an important role in treatment of many diseases caused by sensitive microbes. As they are effective in infective diseases caused by pathogens, intracellularly-penetrating in particular, encouraged the drug specialists for the development of newer derivatives with improved kinetics, dynamics and tolerance (
Seiple et al., 2016). However, the penetrating power and the ability of this drug group to accumulate intracellularly (
Labro, 1993)may, in addition, cause some alterations in the host cell functions with new interests in their pharmacologic and therapeutic potentials other than antimicrobial one (
Bryskier et al., 2008). The macrolide antibiotic tilmicosin is a tylosin-derivative being used in treatment of respiratory diseases in different animal species. Despite the inflammatory modulating potential of macrolide members, particularly that of erythromycin, has been reported (
Labro, 1998;
Steel et al., 2012), however, there is no, at least for our information, any data reported about antipyretic potential of this drug group. Normal body temperature is circadian and varies within about 0.5°C below and above from the morning to the late afternoon (
Faull et al., 2015;
Hunskaar and Hole, 1987). Thermoregulation is managed by integrated network of neural connections within CNS orchestrated in an area in the hypothalamus named the “preoptic area” which includes the preoptic nuclei located in the anterior hypothalamus (POAH). Simply, the POAH keeps mean body temperature around a particular set-point that is a characteristic for every species. Such normal set-point temperature could be modulated by the balance between functional activities of temperature-sensitive neurons. Such neurons integrate degrees of temperature according to afferent signals from inside the body and its periphery (skin) and evoke various behavioural and physiologic responsive activities integrating heat gain or heat loss (
Mackowiak, 1997;
Vriens et al., 2014).
Fever or pyrexia is an abnormal rise in the body temperature after an induced increase in the thermos-neutral set-point (
Mateusen et al., 2001)by various causes as infections, tissue damages and inflammatory disorders. Upon setting temperature to a higher degree in the hypothalamus, the actual body temperature becomes lower that was set centrally. Consequently, and under the control of the hypothalamus, body physiological and behavioural functional activities favouring heat gain and retention are stimulated until arriving the body at the newly induced raised set-point temperature. Behavioural alterations including shelter seeking and grouping (animals) or adding clothes (human) and physiological alterations, including goose skin, peripheral vasoconstriction, shivering, and non-shivering thermogenesis
via enhanced release of thyroid, glucocorticoid and catecholamine hormones occur till achieving the raised set-point of body temperature. Now, a condition of fever or pyrexia becomes settled as the thermoregulation mechanism modulates at this higher set point. Pathophysiological mediators (and hence the pharmacological targets) underlying pyrexia have been described. Endogenous pyrogens including pyrogenic cytokines, like interleukin-1β, tumour necrosis factor (TNF), and interleukin-6, are among those that are acting directly on the hypothalamus to modulate a feverish response (
Croft et al., 2000). Exogenous pyrogens, such as surface components of microbes as lipopolysaccharides lipopolysaccharides (LPS), induce fever most probably
via induction of pyrogenic cytokines capable of functioning at the level of the hypothalamus, in the same way as interleukin-1β (
Ramadan, 1997). These signals trigger the release of other mediators, most notably PGE2, in the region of the POAH (
Mateusen et al., 2001). Among prostaglandins, PGE2 has been proved to be the downstream mediator of the feverish response. Pre-optic neurons have, on their membranes, E-prostanoid receptors that alter their intrinsic firing rate upon its activation by PGE2, with a result of an elevated febrile set point. Four cellular receptors for PGE2: EP1 through EP4 were proved and reported (
McKay et al., 1996;
Mora et al., 2013). However, the specific receptor subtype involved in pyrogenesis is still not yet understood.
PGE2 is produced from its precursor arachidonic acid, which is freed from the injured phospholipid cell membranes by phospholipase-A2 (PLA2). Arachidonic acid is a substrate metabolized by COX enzyme that has two isoforms, COX-1 and COX-2. The isoform COX-1 is, usually, expressed constitutively and produces prostanoids important for some housekeeping functions supporting homeostasis as gastric mucosal integrity and renal artery blood flow (
Simon, 1999). While the second isoform, COX-2, on the other hand, is inducible by inflammatory signals such as the pyrogenic cytokines, interleukin-1β, tumour necrosis factor, and interleukin-6, and bacterial lipopolysaccharide (
Simon, 1999). The most likely cell type in the central nervous system responsible for producing PGE2 is the microvascular endothelial cell, which expresses COX-2 abundantly after stress (
Cao et al., 1996). Fever is regulated by the body immune response. Therefore, on the other hand, release of endogenous antipyretic substances is provoked (
Jordan et al., 1999)like arginine anti-diuretic hormone (vasopressin), melanocyte stimulating hormone (MSH), interleukin-10 and glucocorticoids act both centrally and peripherally to limit pyrexia. Besides, a group of lipid substances known as epoxy-eicosanoids produced by certain cytochrome P-450 enzymes play an important role in limiting the fever and inflammation (
Mackowiak, 2000). Moreover, fever itself appears to counter the release of pyrogenic cytokines (
Mackowiak, 1997). An effective antipyretic may suppress peripheral inflammation and/or central pyrogenic signals. Suppressing central production of PGE2 is a well-established mechanism of antipyretic drugs, however, stimulated leukocytic and endothelial cells in peripheral inflammatory areas also constitute potential peripheral drug target(s). Two hypotheses may explain the mechanism of antipyretics; the cyclooxygenase-dependent hypothesis and the cyclooxygenase-nondependent hypothesis. In the former, the action of an antipyretic is mediated by inhibiting the production of prostaglandins
via inhibiting COX as aspirin (
Xu et al., 1999). Inhibition of COX-2 by aspirin as well as other
NSAID occur via inhibiting its transcription by interrupting a transcriptional activator, named, nuclear factor-kB (NF-kB) (
Xu et al., 1999). NF-kB (normally kept inactive in the cytoplasm another protein named IkB) is a heterodimeric protein capable of binding DNA of many genes that are involved in the signalling pathway of inflammatory response. Once involved, NF-kB promotes the transcription of genes encoding pyrogenic cytokines (
Kirtikara et al., 2000). The non-cyclooxygenase hypothesis indicates that actions of antipyretics may also be mediated by suppressing tissue inflammation by diminishing leukocyte-endothelial cell interactions (
Pierce et al., 1996), reducing pyrogenic cytokine production (
Ahrens, 1996), enhancing expression of anti-inflammatory molecules (
Ahrens, 1996)or boosting the activity of endogenous antipyretic messengers (
Wilkinson and Kasting, 1990). In the Brewer’s yeast-induced pyrexia model, it was found that rectal temperature as well as the percentage of its reduction exhibited by tilmicosin at dose levels of 20 and 40 mg/kg body weight after yeast injection was quite noticeable and comparable to the standard ASA group. The observed antipyretic effect was found to be sustained and lasted up to at least 5 h after single subcutaneous administrations of the drug samples which showed dose-dependent pattern of antipyretic efficacy. The antipyretic effect of tilmicosin might be due to interruption of pyrexogenesis at any step that connects peripheral inflammation with the central production of PGE2. Although there is no data that supports this speculation, yet the anti-inflammatory effect of macrolides has been reviewed by Labro (1998) who reported that the macrolide antimicrobial drugs have immuno-modulatory potentials; where
in-vitro data suggested that derivatives of erythromycin may have a direct effect on neutrophil function with altered generation of cytokines involved in the inflammation pathway. In addition,
ex-vivo data indicated that short-term administration of macrolide(s) might increase the immune response while its long-term administration, in contrast, might result in immuno-suppression. Further studies are needed to improve the current concepts of the pharmacology and therapeutics of macrolides.
The authors have not declared any conflict of interests.