ABSTRACT
Selection of wheat varieties that have improved adaptation to abiotic stress is important for increasing and stabilizing yields under fluctuating environmental conditions, especially as global climate changes. A trial to estimate adaptation of wheat (Triticum turgidum subsp. durum) genotypes to abiotic stress has been performed, in a growth chamber. By counting the number of dead (yellow) plants, together with yellow and green leaves, and hence traits that easily can be also detected by automatized phenotyping platforms, were analyzed for the effects of optimal watering, progressive water deficit and different levels of heat stress. “Trinakria” variety and two Trinakria mutants (“Water-mutant” and “Hg-mutant”) altered for water-related physiological traits were examined. The use of very genetically close genotypes had the aim to minimize differences in stress response due to asynchronous phenological development and to evaluate better the protocol usefulness to detect minimal phenotypic differences, such as those found between advanced breeding lines, at the final stages of a breeding program. Results showed that Trinakria had a significantly greater % of green leaves under drought stress and retained green leaf after heat stress ceased. In contrast, the two mutants had improved plant survival after moderate heat stress. In conclusion, an examination of leaf color changes under moderate water deficit and heat stress was sufficient in a differential comparison of genotypic performances.
Key words: Abiotic stress, leaf color, phenotyping, wheat.
Despite world-wide efforts to select high yielding varieties, a decline in wheat production has been observed from the beginning of this millennium; mainly due to a lack of varieties that resist abiotic stress (Dalal et al., 2017). Changes in weather and climate, probably related to global warming, are shown by an increasing incidence of extreme weather phenomena, even during phenological phases in which the problem of dehydration stress was rare. At the vegetative phase, dehydration stress can modify the growth and development of plants (Wittmer et al., 1982; Figueroa-Bustos et al., 2019), so affecting up to 56% of the final yield (Gallagher et al., 1976). According to the concepts advanced by Negin and Moshelion (2017), plants may differ for abiotic stress tolerance, resilience or resistance. Tolerance is the ability of the plant to continue photosynthesis, under stress conditions. Resilience denotes the capability to recover and continue growth when moisture is present after drought. Resistance is the plant capability to withstand extreme stress that generally occur at the end of the growth cycle (terminal stresses), and to complete the growth cycle even if most of the leaves (green biomass) has been lost.
In field conditions, the great variability for heat and water stress types occurring on, together with strong genotype × environment interactions and dependence of phenotype on multiple quantitative traits, make complex the selection for improved agronomic performance (Dhanda et al., 2004). For this reason, since the 1970s (Pomeroy and Fowler, 1973), pre-breeding phenotyping under controlled environmental conditions has been commonly employed for functional characterization of varieties, progenies of crosses, mutants, etc. Nowadays, high-throughput non-destructive phenotyping technologies have greatly increased the number of experimental analyses of the wilting process (Humplík et al., 2015; Watt et al., 2020). Controlled environments provide greater reproducibility of experimental conditions and allow multiple stresses to be tested. However, for both non-automated and automated systems, either used in field or indoor, the developmental stage of the plants, stress history, spatial and temporal randomization of plants and micro-environmental fluctuations affect the phenotype which is scored (Yeh et al., 2012). Using separate pots to impose stress on plant, with different morphological-physiological traits, results in application of stresses which are not comparable in timing relative to development stages, and different intensity of the stress. Thus, those plants with greater leaf area, with thinner laminae and/or increased stomatal conductance, and well developed roots, will suffer onset of a water deficit more rapid and greater stress intensity, due to a greater velocity of water loss (Lawlor, 2012). Finally, the trait type to be measured by pre-field screening should be evaluated based on the required performance of plants in field. As an example, a tolerant plant that does not change its physiological activity under early drought and hence has no heavy green leaf loss, will have only a small reduction in yield. Analogously, resilient plants that show the ability to recover their functional activity soon after the stress has ended, are suitable for cultivation in environments where stress is short and intermittent. Highly resistant plants that survive and produce seeds also if with heavy loss of leaves, could have stable yield in cultivation environments where stress generally occurs at the end of the growing cycle (Negin and Moshelion, 2017).
By counting the number of dead (yellow) plants, together with yellow and green leaves, and hence traits that easily can also be detected by automatized phenotyping platforms, a physiological characterization of wheat, during progressive dehydration or after heat stress treatment were analyzed. Water deficit was applied by stopping irrigation, while in a separate experiment, four levels of heat stress were applied by increasing temperature up to 46°C. To minimize differences for stress response due to non-synchronous phenological development and to evaluate the capability of our experimental conditions to detect minimal phenotypic differences (like those that can be found between advanced breeding lines, at the final stages of a breeding program), 3 very genetically closed genotypes were used. They were “Trinakria” variety and 2 mutant lines of Trinakria. The first, called “Water-mutant”, has a high affinity for water fraction that is bound to the macromolecule surfaces (Rascio et al., 1999) and the second, named “Hg-mutant” is partially insensitive to HgCl2, an aquaporin inhibitor. Both traits of the 2 mutants may have protective roles against dehydration stresses. Bound water is essential for structural integrity of bio-molecules (Vertucci and Leopold, 1987). Also, it may exert a passive control of osmotically active volume of the cell (Rascio et al., 2005). Aquaporins are membrane intrinsic proteins that facilitate water transport; their up-regulation or down-regulation under stress conditions is thought to be important for tolerance to drought stress (Sade et al., 2009).
Thirty plastic pots (Figure 3) were each filled with 4 L of a mixture of soil and sand (50:50 v/v) with a maximum water-holding capacity of 0.32 g H2O/g dry weight. The soil mixed to the sand was a clay-loam soil (Typic Chromoxerert), with the following physical and chemical characteristics: 36.9% clay, 50.5% silt, 12.5% sand, 15 mg/kg organic matter and pH 8. The pots were put in a 5 × 3.5 m2 growth chamber, at 20°C/16°C, for a 10 h/14 h light/dark period. Plants were grown under 250-W high-pressure sodium lamps (Philips) and 400-W high pressure metal halide lamps (Philips). Radiation at the pot surface was 1000 µmol photons m-2 s-1 (400-700 nm) PAR. Fertilizer was applied before sowing: 18 g m−2 ammonium sulfate and mineral superphosphate. Cultivation of one plant per pot may create differences among them for stress history and dehydration velocity of soil, due to different growth of plants. For this reason, all pots were subdivided in three parts. Each of them represented one replicate and contained 24 seeds: 8 of “Trinakria”, 8 of “Water-mutant” and 8 of “Hg-mutant”. Seeds are part of the genotypic working collections, stored at CREA-CI of Foggia (Italy). Distribution of genotypes into each pot section and of pots within the growth chamber was random. After the emergence, to avoid damages to the roots, the plants were not thinned to the same number of plants per genotype, so the final number of plants examined for each per genotype and treatment (Table 1) was different. Before drought and after heat stress, the pots were always kept well-watered, with water loss restored every 2 days to about 80% of maximum soil capacity. When most of the plants had four fully-expanded leaves and hence were at the phase 13-14 of the Zadoks’ scale (Zadoks et al., 1974) 25 uniform pots, with 3-5 well growth plants per genotype and without signs of disease, were selected. Four pots were kept well watered at 20°C/16°C, for a 10 h/14 h light/dark period till the end of the experiment and used as controls.
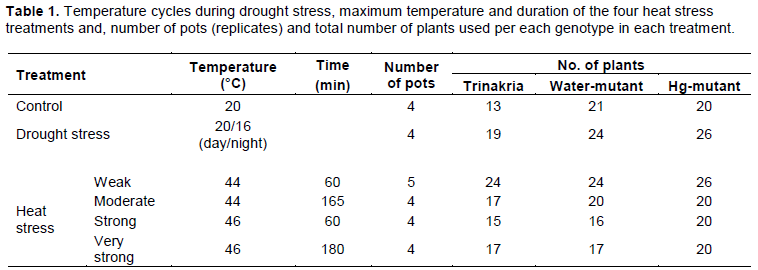
Stress treatments
To perform heat stress experiments, a total of 21 well-watered pots were used. Four stress types, differing for temperature (44-46°C) and heat stress duration for a minimum of 60 to a maximum of 180 min were applied and for each of them 4 or 5 pots at a time, were used (Table 1). They were transferred in a thermostatic cabinet with radiation measured at pot surface equal to 60 µmol m-2 s-1 (400-700 PAR). Seven days after exposure to heat stress treatment, the number of died plants, green and yellow leaves per plant of each genotype in each pot (replicate) were counted.
For drought stress exposure, irrigation was interrupted on four pots. A total of 42 plants per genotype were used to dehydrate in the growth at 20°C/16°C, for a 10 h/14 h light/dark period. Twenty days after, when some symptoms of wilting were visible, the number of dead plants (totally yellow), the number of yellow and green leaves of surviving plants of each genotype in each pot (replicate), were counted. Leaves were classified as yellow if less than 60% of lamina was green. Then, the same measurements were repeated every 2-3 days, for 20 days.
Statistics
All results were analyzed using GraphPad Prism software, version 3.0. Differences among genotypes for percentage of died plants and green leaves were processed by one way analysis of variance (ANOVA), with variable number of replicates (pots) shown in Table 1. Means were compared by the multiple comparison test. Tukey regression analysis was performed to define any associations between the variables.
Water deficit stress
The % of dead plants per genotype, concerning the total plants counted before exposure to the drought stress imposition, as shown in Figure 1. Controls had the same percentage of living plants all over the experiment. On average, 50% of plants died about 22 days after water withdrawal (DAWW), while 75% of plants died between 24 and 34 DAWW (Water-mutant and Hg-mutant, respectively). However, there were no significant differences between genotypes determined at increasing time intervals after water was withdrawn, on the basis of ANOVA (data not shown). Standard Error of the mean values of droughted plants increased with days of water withdrawal, because pot to pot differences in number of survived plants of each genotype were greater when drought stress intensity was stronger.
Using data collected from 13 to 32 days after water withdrawal, for each replicate of each genotype, the regression line was constructed and the number of days to have 50 and 75% of dead plants was interpolated. The estimated number of days required to kill 50% or 75% of plants did not differ significantly between genotypes (Table 2), based on ANOVA. At 22 DAWW (Figure 2) and hence under moderate drought stress, Trinakria cultivar had a significantly greater % of green leaves than the Hg-mutant (F=4.32; P=0.048), but with increased water deficit it showed a similar, decreasing trend to other genotypes and hence no significant differences were observed.
Heat stress
Figure 3A shows the plant’s appearance before the heat treatment (44°C for 2 h, 45’), immediately after (Figure 3B) and 7 days after (Figure 3C). The number of dead plants was counted seven days after exposure to the stress. Based on Tukey’s test (Figure 4), Trinakria mortality increased significantly compared to controls with moderate heat stress. For the other 2 genotypes, % mortality was significantly higher than controls after plant exposure to strong and very strong heat stress. None of the genotypes resisted to intense heat, because all plants died after strong or very strong heat stress (Figure 4). The % of green leaves, 7 days after stress relief is shown in Figure 5. Based on Tukeys’ test, Trinakria had a significant reduction of green leaf number under very strong heat stress intensity, as compared to controls. In contrast, after exposure to weak and moderate heat stress, the % of green leaves of both Water-mutant and Hg-mutant was lower than control.
In temperate climates, water deficit or high-temperature stresses that occur at vegetative stages are often intermittent and of low intensity, but they greatly affect crop yield. To save yield, late drought stress needs resistant plants that survive and produce seeds even if they completely lose the leaves. Tolerant and/or resilient varieties that under abiotic stress are photosynthetically active and hence do not lose their green biomass, could be better useful under early stress.
Results showed that in the system employed, water deprivation caused the death of about 50% of plants 22 days after withdrawing water. Days to kill 75% of plants, ranged from 24 days for Water-mutant, to 32 days for Hg-mutant, but this trait and days to kill 50% of plants did not differ significantly between genotypes. The magnitude of genetic components of variance is, generally, lower under stress conditions than under control conditions (Dhanda et al., 2004). Moreover, the drastic treatment necessary to kill plants increased the well-known variability of plant growth existing within controlled-environment chambers (Measures et al., 1973; Massonnet et al., 2010; Porter et al., 2015). Other authors (Sallam et al., 2018), by using higher average day-night temperature, a single genotype per pot and smaller pots had an average time to 50% wheat wilting, about 13 days shorter than that here. They also observed significant genotypic differences, within a ril population, derived from crosses of more genetically distant parents, as compared to wild type and mutant lines probably due to greater genotypic differences within the ril population; lower duration of the cultivation phase necessary to kill 50%; inability to impose the same speed of dehydration on separate pots if they contain genotypes with different morpho-physiological characteristics, already at the beginning of exposure to the stress.
In contrast to what observed for drought response, protective mechanisms that allow plant acquisition or loss of thermotolerance exist and they are under both genetic and epigenetic control (Larkindale et al., 2005; Liu et al., 2015). In this work, too genotypic differences were observed for heat stress effects on plant mortality, because Trinakria cv. performance was significantly worse than that of the mutants. Starting from moderate stress, its mortality increased compared to controls, while for the other 2 genotypes % mortality increased significantly only after the exposure to strong or very strong heat stress. Furthermore, some methodological factors could be the basis for the minor differences in the genotypic response to water stress compared to heat stress. Water withdrawal lasted twenty days. In this relatively long period, changes in the micro-environmental conditions occurred within the growth chamber which differentially modified the stress history of each pot and hence plant growth. The consequences were that, under drought conditions, large plant to plant differences were observed within replicates of the same genotype. On the contrary, thermal stress exposure lasted a few hours, after which all the surviving plants could express their recovery potentiality because optimal conditions were ensured to all plants.
Trinakria cv. Appeared to preserve better the photosynthesizing apparatus because, one week after the withdrawn of watering under weak or moderate stress, it had the same % of green leaves. In contrast, the 2 mutants had 50% fewer green leaves. The general lack of differences in genotype performance under strong high temperature stress, suggests that physiological mechanisms that differentiate the genotypes are unable to affect their performance at temperatures higher than 45°C.
This study was performed to evaluate the genotypic plant performance under abiotic stress by counting dead (yellow) plant, together with yellow and green leaves per plants of Trinakria cv., Water-mutant and Hg-mutant. Significant differences among genotypes for the examined traits have been observed. This result suggests that the used method was effective in showing differential plant performances plants under abiotic stress conditions, but further experiments in field are necessary to test the agronomic performance of the same genotypes after exposure to early stress. At the same time, to apply this approach in breeding programs by using automatized systems, pots will have to be designed for simultaneous sowing and screening of many genotypes, which will provide equal conditions of competitiveness of root systems and speed of soil dehydration. Because mutations have functional consequences in terms of abiotic stress response, these mutants are potential sources of traits to be used in traditional breeding programs.
The authors have not declared any conflict of interests.
The authors thank Dr. David Lawlor for constructive criticism of the manuscript and are grateful to Mr. Vito Miullo for plant cultivation and Mr. Luigi D’Angelo for data collection.
REFERENCES
Dalal A, Attia Z, Moshelion M (2017). To Produce or to Survive: How Plastic Is Your Crop Stress Physiology? Frontiers in Plant Science 8:2067.
Crossref
|
|
Dhanda SS, Sethi GS, Behl RK (2004). Indices of drought tolerance in wheat genotypes at early stages of plant growth. Journal of Agronomy and Crop Science 190:6-12.
Crossref
|
|
|
Figueroa-Bustos V, Palta JA, Chen Y, Siddique KH (2019). Early season drought largely reduces grain yield in wheat cultivars with smaller root systems. Plants 8:305.
Crossref
|
|
|
Gallagher JN, Biscoe PV, Hunter B (1976). Effects of drought on grain growth. Nature 264:541-542.
Crossref
|
|
|
Humplík JF, Lazár D, HusiÄková A, Spíchal L (2015). Automated phenotyping of plant shoots using imaging methods for analysis of plant stress responses-a review. Plant Methods 11:29.
Crossref
|
|
|
Larkindale J, Hall JD, Knight MR, Vierling E (2005). Heat stress phenotypes of Arabidopsis mutants implicate multiple signaling pathways in the acquisition of thermotolerance. Plant Physiology 138:882-897.
Crossref
|
|
|
Lawlor DW (2012). Genetic engineering to improve plant performance under drought physiological evaluation of achievements limitations and possibilities. Journal of Experimental Botany 64:83-108.
Crossref
|
|
|
Liu J, Feng L, Li J, He Z (2015). Genetic and epigenetic control of plant heat responses. Frontiers in Plant Science 6:267.
Crossref
|
|
|
Massonnet C, Vile D, Fabre J, Hannah MA, Caldana C, Lisec J, Perkovic J (2010). Probing the reproducibility of leaf growth and molecular phenotypes a comparison of three Arabidopsis accessions cultivated in ten laboratories. Plant Physiology 152:2142-2157.
Crossref
|
|
|
Measures M, Weinberger P, Baer H (1973). Variability of plant growth within controlled-environment chambers as related to temperature light distribution Canadian Journal of Plant Science 53:215-220.
Crossref
|
|
|
Negin B, Moshelion M (2017). The advantages of functional phenotyping in pre-field screening for drought-tolerant crops. Functional Plant Biology 44:107-118.
Crossref
|
|
|
Pomeroy MK, Fowler DB (1973). Use of lethal dose temperature estimates as indices of frost tolerance for wheat cold acclimated under natural and controlled environments. Canadian Journal of Plant Science 53:489-494.
Crossref
|
|
|
Porter AS, Gerald CEF, McElwain JC, Yiotis C, Elliott-Kingston C (2015). How well do you know your growth chambers? Testing for chamber effect using plant traits. Plant Methods 11:44.
Crossref
|
|
|
Rascio A, Russo M, Platani C, Ronga G, Di Fonzo N (1999). Mutants of durum wheat with alterations in tissue affinity for strongly bound water. Plant Science 144:29-34.
Crossref
|
|
|
Rascio A, Nicastro G, Carlino E, Di Fonzo N (2005). Differences for bound water content as estimated by pressure-volume and adsorption isotherm curves. Plant Science 169:395-401.
Crossref
|
|
|
Sade N, Vinocur BJ, Diber A, Shatil A, Ronen G, Nissan H, Moshelion M (2009). Improving plant stress tolerance and yield production is the tonoplast aquaporin SlTIP2; 2 a key to isohydric to anisohydric conversion? New Phytologist 181:651-661.
Crossref
|
|
|
Sallam A, Mourad A M, Hussain W, Baenziger PS (2018). Genetic variation in drought tolerance at seedling stage and grain yield in low rainfall environments in wheat (Triticum aestivum L.). Euphytica 214:169.
Crossref
|
|
|
Vertucci CW, Leopold AC (1987). Water binding in legume seeds. Plant Physiology 85:224-231.
Crossref
|
|
|
Watt M, Fiorani F, Usadel B, Rascher U, Muller O, Schurr U (2020). Phenotyping: New Windows into the Plant for Breeders. Annual Review of Plant Biology, p. 71.
Crossref
|
|
|
Wittmer G, Iannucci A, De Santis G, Baldelli G, De Stefanis E, Brando A, Ciocca L, Rascio A (1982). Adattamento e fattori fisiologici limitanti la produttività del frumento duro. Rivista di Agronomia 2:61-70.
|
|
|
Yeh CH, Kaplinsky NJ, Hu C, Charng YY (2012). Some like it hot some like it warm phenotyping to explore thermotolerance diversity. Plant Science 195:10-23.
Crossref
|
|
|
Zadok JC, Chang TT, Konzak CF (1974). A decimal code for the growth stages of cereals. Weed Research 14:415-421.
Crossref
|
|