ABSTRACT
Maize is staple cereal crop in Ethiopia despite the fact that its production is constrained by nitrogen deficiency due to the high cost of fertilizer, and risks of drought. Therefore, development of maize varieties for low nitrogen might be one of the options to overcome the problem. The objective of this study was to estimate combining ability of highland maize inbred lines for yield and yield related traits under low nitrogen (low N) stress and non-stress conditions. Twenty-six inbred lines (two heterotic testers and twenty-four lines) were crossed using line × tester mating design, which generated 48 F1 hybrids and along with two hybrid checks (AMH853 and AMH 851) that were evaluated using alpha lattice design with two replications for grain yield and yield related traits within 2017 cropping season at Ambo under low and optimum nitrogen. Analyses of variances showed significant mean squares due to crosses for all traits under both low N stress and non-stress conditions, except for ear per plant under low N stress condition. The mean squares for general (GCA) and specific (SCA) combining abilities were significant for most of the traits under both conditions. Generally, the study indicated the importance of both additive and non-additive gene effects in most cases, while non-additive gene effects are less important under low-N stress. Inbred lines L1, L2, L9 and L20 were found as good combiners for grain yield at optimum N environment, whereas L5 and L14 were good general combiners under low N stress condition. L20 were good combiner for grain yield in combine analysis across environments and hence were promising parents for hybrid cultivars development. Based on SCA effects and per se performance, L5×T2 and L7×T2 were identified as promising hybrids for majority of traits studied in combined analysis across environments.
Key words: General combining ability, gene action, heterotic group, specific combining ability.
Maize (Zea mays L) is an important cereal in the world, belonging to the tribe Maydeae of the grass family Poaceae (Acquaah, 2007). It is an important stable food crop for many people around the world. As the cultivation of early maize spread to different geographical regions from Mexico and Central America, where maize is widely believed to have originated, there was a rapid evolution of many races adapted to a wide variety growing conditions. Maize is a popular and widely cultivated food crop in Africa since its introduction to the continent around 1500 A.D. by Portuguese traders (McCann, 2005) and then arrived in Ethiopia slightly later, around the late 17th century (Huffnagel, 1961).
Ethiopia is the fifth largest producer of maize in Africa and smallholder farmers make up 94% of the crop production (http.//ethioagp.org, 2017). Maize ranks second after teff in area coverage and first in total production (CSA, 2017). It is the most widely consumed grain. According to CSA data, 80% of maize production is used for household consumption, 10% is sold on the open market, the remainder is used for seed, wages in kind, and animal feed (USDA, 2015). Maize research in Ethiopia started in 1950’s with the evaluation of introduced materials focusing mainly on grain yield, early maturity, decreased plant height, lower ear placement and resistance to major biotic stresses (Benti, 1992). Since then, the research system has developed and released a number of improved varieties with their accompanying agronomic practices and plant protection technologies for all maize growing agro-ecologies of the country.
Average yield of maize in Ethiopia is 3.67 t ha-1 (CSA, 2017) which is lower than the world average 5.65 t ha-1 (USDA, 2018). The wide yield gap is attributed to an array of abiotic and biotic stresses. In spite of its wide adaptation and efforts made to develop improved maize technologies for different maize agro-ecological zones, many biotic and abiotic constraints still, limit maize production and productivity in different maize producing area of Ethiopia (Abate et al., 2017). The major abiotic stresses in the highland zones are frost, hail and water-logging (on Vertisols). Soils are characterized by undulating terrain, low fertility, and the region is characterized by wide variations in climatic conditions (Twumasi et al., 2002). Low soil fertility is mainly due to low soil nitrogen (N); N deficiency is common where N is applied at below optimal levels because of high cost of mineral fertilizer relative to the economic returns, or when there are significant risks of drought (Lafitte and Edmeades, 1994).
The high altitude, sub-humid maize agro ecology (1800-2400 m.a.s.l.) in Ethiopia is estimated to cover 20% of the land devoted to annual maize cultivation. Adoption of maize is increasing in the highland agro-ecology (Demissew et al., 2013). To meet the needs of increasing maize production in the highlands of Ethiopia, the Highland Maize Breeding Program was established in collaboration with the International Maize and Wheat Improvement Center (CIMMYT), in 1998. Since 1999, the breeding program has released seven superior highland maize cultivars for wide production.
The use of cultivars that utilize nitrogen more efficiently could greatly improve maize productivity in maize-based cropping systems. So far, combining ability effects in maize inbred lines has been extensively studied under non-stressed conditions for different sets of new maize inbred lines developed/introduced and adapted at different times (Amare et al., 2016; Ziggiju et al., 2016; Abakemal et al., 2016; Assefa et al., 2017). Even though CIMMYT has made significant progress in developing maize germplasm tolerant to low N (Banziger and Lafitte, 1997; Banziger et al., 1997; Worku et al., 2008; Dagne, 2008; Mohamed et al., 2014; Mafouasson et al., 2017). Information is still limited regarding combining ability of maize inbred lines and choosing the best testers to use when developing stress tolerant single and three-way cross hybrids for the highlands.
Understanding the relative importance of general and specific combing ability effects for different traits for newly developed and/or introduced inbred lines is of paramount importance to design future breeding strategies for the development of hybrid and/or synthetic varieties. In the current study, therefore, an attempt was made to identify high yielding hybrids tolerant to low N soil, determine the combining abilities and mode of gene action of elite maize inbred lines for hybrid development under low and optimum nitrogen conditions.
Experimental site
The field experiments were conducted at Ambo Agricultural Research Centers during the 2017 main cropping season. Geographically, Ambo is located at 8°57'N latitude, 38°7'E longitude and at an altitude of 2225 m.a.s.l with average annual rainfall of 1110 mm, maximum and minimum temperature of 26 and 11°C, respectively. The soil type of the experimental field is vertisols (http.//www.eiar.gov.et/index.php/research-centers). The total precipitation during the growing season (May to December 2017) was 864.1 mm, and the mean minimum and maximum temperatures were 10.51 and 24.1°C, respectively (Ambo Agricultural Research Centers Meteorological Station, Unpublished Data).
Experimental design
The hybrids were planted in alpha-lattice design (Patterson and Williams, 1976) with two replicates. Design and randomization of the trials were generated using CIMMYT’s software known as field book (Banziger and Vivek, 2007). One-row plots of 5.25 m length and 75 × 25 cm spacing between rows and plants were used to achieve 53,333 plants/ha. Two seeds were hand planted per hill and later thinned out to have one plant per hill after seedlings established well.
The hybrids were evaluated under optimal and low N conditions in adjacent fields with the same soil type in 2017. The experiment under low N-stress condition was laid in a field that had been depleted of N by continuous cropping of maize for several seasons and removing the crop residues after each season. No additional N fertilizer was applied. Under non-stress N conditions, the recommended rate of diammonium phosphate (DAP) fertilizer was applied once at planting using a rate of 100 kg ha-1 while 200 kg ha-1 of Urea was applied in split at planting, knee height and flowering stages of the crop. Other crop management practices were carried out as recommended for the location.
Soil sampling and analysis
Soil samples from the experimental sites were taken before planting. First, one representative composite soil sample was taken from ploughed and leveled field at three places diagonally across the plot (in zigzag method) with auger. Samples were taken from 0 to 30 cm and 30 to 60 cm depth of top soil and composited to make one representative soil sample for each depth before planting. The composited soil samples were subjected to analysis before planting. Results of the soil analysis are shown in Table 1.
Experimental materials
The experiment consisted of 48 test crosses produced by crossing 24 inbred lines to two testers in line × tester mating design, and two standard checks (AMH851 and AMH853). The inbred lines were introduced from CIMMYT-Zimbabwe. The two testers, FS59 (Tester 1) and FS67 (Tester 2) are adapted lines locally developed at Ambo. FS59 is heterotic group B while FS67 is heterotic group A. The lines × tester crosses were made by highland maize breeding program during the main season of 2016. AMH851 and AMH853 are commercial hybrid checks released for and produced in the highland agro-ecologies of Ethiopia. The list and pedigrees of the inbred lines and testers used for the study are shown in Table 2.
Data recorded
Data on grain yield and other agronomic traits were collected on plot and individual plant basis. Anthesis date (AD) and silking date (SD) were recorded as ‘number of days after planting’, when 50% of plants were shedding pollen and silking, respectively. The anthesis-silking interval (ASI) was calculated as silking date minus anthesis date. Leaf senescence (SEN) was scored 10 and 12 weeks after planting on a scale from 0 to 10, dividing the percentage of the estimated total leaf area below the ear that is dead by 10. A score of 1 = less than 10% dead leaf and 10 = more than 90% dead leaf. Plant height (PH) was measured as the average height of five randomly selected plants measured in cm from base of the plant to the first tassel branch. Ear height (EH) was measured as the average height of five randomly selected plants measured in cm from base of the plant to the node bearing the upper most ear of the same plants used to measure plant height. At harvest, the number of ears per plant (EPP) was computed as the total number of harvested ears in each plot divided by the stand count at harvest. Number of kernels per row (NKR) was recorded by counting kernels in each row from five randomly taken ears and the average value was recorded as kernels per row. Number of kernel rows per ear (KRE) this was measured as total number of kernel rows of the ear was counted from five randomly taken ears and the average value was used as kernel rows per ear. Thousand kernels weight (TKWT) was recorded as the weight in grams of 1000 random kernels was weighed from each plot using sensitive balance and was adjusted to 12.5% moisture level. Grain yield (t ha-1) was measured as the total grain yield in kg per plot and adjusted to 12.5% moisture level was used to calculate grain yield per hectare.
Data analysis
Prior to data analysis, anthesis-silking interval (ASI) was normalized using lnSQRT (ASI +10) as suggested by Bolaños and Edmeades (1996). Analysis of variance per environment was conducted with the PROC MIXED procedure in SAS (2002) considering genotypes as fixed effects and replications and blocks within replications as random. Relative reductions in grain yield and agronomic traits under low N was calculated as (1 - MV low N / MV optimum N), where MV low N are mean traits values obtained in experiment under low N and MV optimum N are mean traits values obtained in experiment under optimum N (Banziger et al., 1997). Combined analysis across environments also computed using PROC GLM in SAS software version 9.0 (SAS, 2002). The combined analysis was done for the significant trait in individual location analysis after testing the homogeneity of error variances through the application of the F-test (Gomez and Gomez, 1984).
Further analysis was done according to the line × tester analysis (Kempthorne, 1957) to partition the mean square due to crosses into lines, tester and line by tester effects (Dabholkar, 1999) using SAS computer program (SAS, 2002) for traits that shows significant differences among crosses. General combining abilities of lines and testers, and specific combining abilities of lines by testers were computed for the characters that show significant differences among crosses in the ANOVA.
Hybrids exhibited highly significant (P<0.01) differences in most traits under low and optimum N conditions at Ambo except number of kernels per row under low N (Table 3). Combined analyses were performed for the traits that showed significant genotypic mean squares for individual location analysis and homogenous error variance analyzed using F-test (Gomez and Gomez, 1984). Combined analysis of variance across environments revealed that all traits exhibited highly significant (P<0.01) differences among the hybrids (Table 4). Significant differences observed among hybrids for individual and across environments indicated the existence of a high level of variation for various characteristics, which makes selection possible for improved grain yield and agronomic traits under low N stress and non-stress conditions. Similar results have been reported (Dagne, 2008; Amare et al., 2016; Abakemal et al., 2016; Bullo and Dagne, 2016; Keno et al., 2017; Mafouasson et al., 2017).
Mean performance of genotypes
The mean grain yield for hybrids tested under optimum N ranged from 5.62 to 10.76 t ha-1 with a mean value of 7.73 t ha-1 (Table 5). Among the crosses, 17 crosses showed significantly higher yield than the hybrid check Jibat and one cross-revealed significantly higher yield than the check hybrid Kolba. Under low N experiment, mean grain yield for all hybrids were 3.23 t ha-1 ranging from 2.13 to 4.61 t ha-1 (Table 5). Worku et al. (2008), Mohamed et al. (2014), Mafouasson et al. (2017), Talukder et al. (2016) and Assefa et al. (2017) in their studies reported that experimental varieties showed better performance than the best check for most yields and other traits.
Mean relative grain yield loss under low N was 58.2%.
Mean relative loss of days to anthesis, days to silking and anthesis-silking interval increased by 18.1, 18. 5 and 0.8%, respectively. Plant height, ear height and ears per plant decreased by 16.3, 16.5 and 33.1, respectively (Table 5). The level of yield loss between low and high N varied depending on the degree of N depletion in the soil (Banziger and Lafitte, 1997). Banziger and Lafitte (1997) reported a significant reduction in plant height (27.1%), ears per plant (11.2%), grains per ear (47.8%) and grain weight (30.7%) under low N. Presterl et al. (2003) and Worku et al. (2008) reported that low-N stress reduced grain yield by 37 and 64%, respectively.
Combining ability analyses
The partitioning of significant crosses mean squares into general combining ability (GCA) and specific combining ability (SCA) showed that SCA mean squares were significantly different for grain yield under optimum N condition (Table 3). Line GCA means squares were significantly different for grain yield at Ambo under optimum and low condition (Table 3). In combined analysis across environments, significant GCA and SCA mean squares were observed for grain yield (Table 4) which implied that importance of both additive and non-additive gene actions in governing grain yield. In agreement with the present study, Tamirat et al. (2014), Girma et al. (2015), Bullo and Dagne (2016) and Amare et al. (2016) were reported the importance of both additive and non-additive gene actions in governing grain yield in maize.
GCA sums of squares were larger than SCA sums of squares for grain yield under low, optimum N and across environments 55, 66 and 67.4%, respectively (Tables 3 and 4). The predominance of GCA sums of squares to SCA sums of squares for grain yield indicated the relative importance of additive gene action to non-additive gene action for this trait (Beck et al., 1990). In line with this study, Tamirat et al. (2014) reported the preponderance of additive gene action in the inheritance of grain yield while in contrast to these findings, Kanagarasu et al. (2010) and Melkamu (2013) reported the dominant role of SCA gene action in the grain yield of maize.
In combined analysis, significant GCA and SCA mean squares were observed for anthesis and silking date (Table 4) that implied the importance of both additive and non-additive gene actions in governing these traits. Results of this study are in accordance with the findings of Melkamu (2013), Shushay et al. (2013), Tamirat et al. (2014) and Girma et al. (2015) who reported significant mean squares due to GCA and SCA for days to anthesis and silking.
Mean squares due to crosses for plant and ear height were highly significant (P < 0.01). Combining ability analysis revealed that highly significant GCA effects of lines and testers for plant and ear height under both environments. SCA mean squares were highly significant under optimum N condition but non-significant under low N (Table 3). In combined analysis across environments, significant GCA and SCA mean squares were observed for plant and ear height (Table 4). In line with these findings, Worku et al. (2008) reported high mean square due to GCA, SCA effects under high N and also Demissew et al. (2011) found significant GCA and SCA mean squares for plant and ear height, in contrast to these finding. Gudeta (2007) reported significant GCA and non-significant SCA mean squares for plant height.
Mean squares due to crosses for number of ears per plant were highly significant (P < 0.01) at Ambo optimum N condition while it is non-significant under low N condition. Significant GCA of lines and SCA mean squares were observed under optimum nitrogen environment. In contrast to these findings, Abakemal et al. (2016) reported non-significant mean square due to line GCA and significant SCA mean squares. GCA sums of squares were larger than SCA sums of squares for number of ears per plant under both conditions (Table 3). Highly significant differences were observed among crosses and tester GCA for thousand-kernel weight under both N condition (Table 3). In combine analysis across environments, significant GCA and SCA mean squares were observed for the trait (Table 4). In agreement with the present results, Kanagarasu et al. (2010) and Abakemal et al. (2016) reported significant mean squares due to GCA and SCA for thousand-kernel weight.
Estimates general combining ability effects
The estimates of line GCA effects for grain yield and yield related traits under optimum N at Ambo are shown in Table 6. The inbred lines varied significantly in GCA for all traits. Line GCA for grain yield varied from -1.91 to 1.26 t ha-1. Even though a total of 13 lines showed positive GCA effects for grain yield only four inbred lines L1, L2, L9 and L20 showed highly significant positive GCA effects for grain yield. In contrast, L4, L13, L15, L16 and L21 had significant negative GCA effects for grain yield. Among the testers, none of them showed significant GCA effects for grain yield per hectare. Line GCA effects for days to anthesis ranged between -4.55 and 3.45. The female parents L9, L10, L14, L18 and L20, revealed positive and significant GCA effects contributed to late maturity. The female parents L3, L7, L8, L12, and L20 were the best general combiners for days to anthesis, which exhibited negative and significant GCA effects. The GCA estimates of parental lines ranged from -4.1 to 3.4 for days to silking. The female parents L3, L7, L8, L12 and L23 were negative and significant difference of GCA effects. Result of the current study are in accordance with the findings of Melkamu (2013), Girma et al. (2015), and Amare et al. (2016) who reported significant positive and negative GCA effects for these traits in their combining ability study.
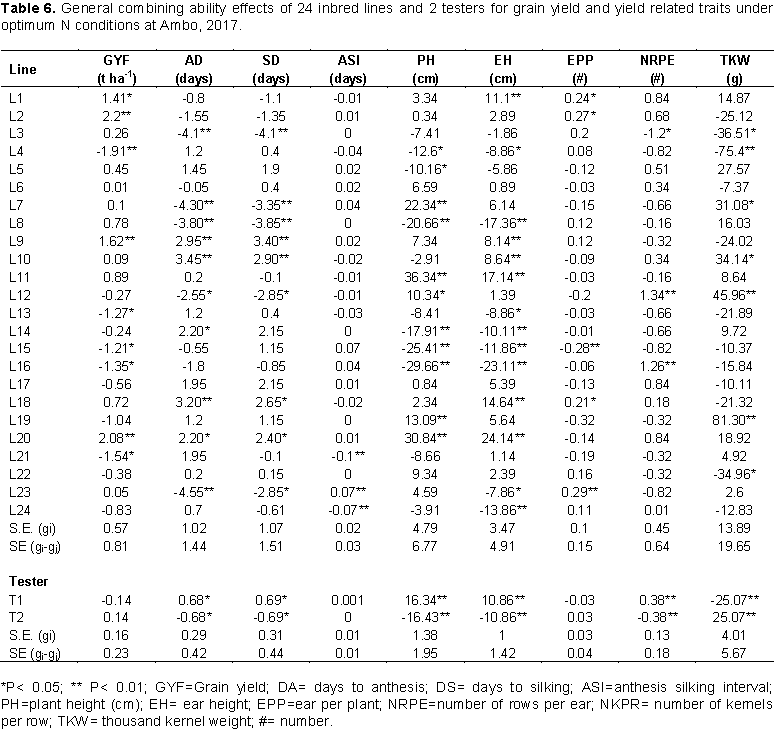
General combining ability estimate of lines for plant and ear height ranged from -29.66 to 36.34 cm and -23.11 to 24.14 cm, respectively. Inbred lines L7, L11, L12, L19 and L20 showed significant negative line GCA effects for plant height and inbred lines L8, L14, L15, L16 and L24 showed highly significant negative line GCA effects for ear height. In maize, shorter plant height is desirable for lodging resistance. L1, L2, L18 and L23 had significantly high positive line GCA effects for ears per plant while L15 showed significantly high negative GCA effect. Kanagarasu et al. (2010) and Shushay et al. (2013) reported similar results for these traits.
Inbred lines L12 and L16 showed highly significant (p<0.01) positive line GCA effects for number of rows per ear while inbred lines L18 and L20 showed highly significant (p<0.01) positive line GCA effects for number of kernels per row. For thousand-kernel weight, significant positive line GCA effects were observed for L7, L11, L12 and L19 while significant negative line GCA effects were observed for L13, L14, and L22. Both positive and negative GCA effects were reported in maize by several investigators (Tamirat et al., 2014; Chandel and Mankotia, 2014). The estimates of line GCA effects of the inbred lines for various traits under low N conditions at Ambo are shown in Table 7. Inbred lines L5 and L14 had significant positive line GCA effects for grain yield while none of inbred line had significant negative line GCA effects. L3, L7, L8 and L14 had highly significant negative line GCA effects for days to anthesis while inbred lines L9, L10, L18, L20 and L21 showed highly significant positive line GCA effects for this trait. The female parents L3 and L23 were negative and highly significant difference (P<0.01) GCA effect for days to silking. The result of this study is in accordance with Mafouasson et al. (2017), who found desirable GCA effects for these traits in combining ability and gene action of tropical maize inbred lines under low and high nitrogen conditions.
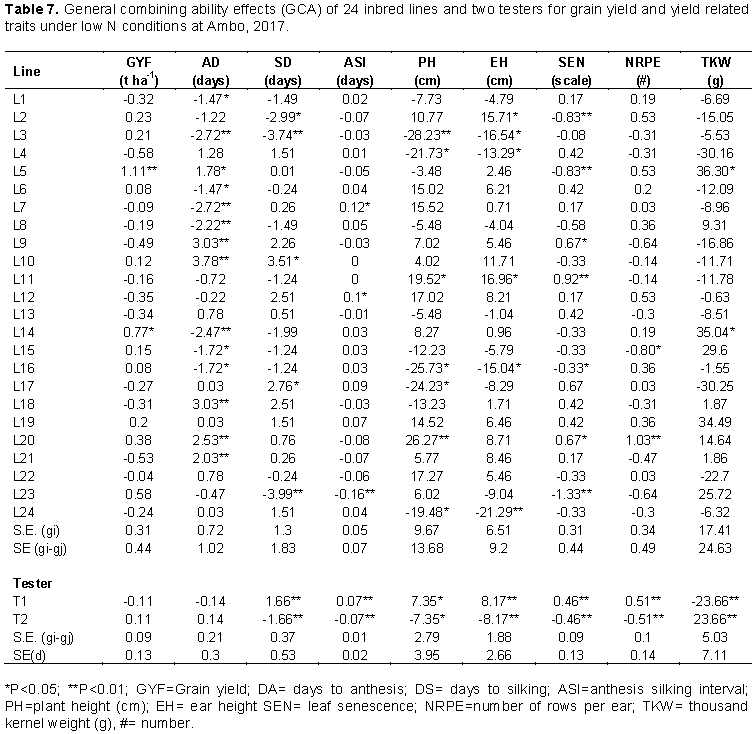
Significant negative line GCA effects for plant and ear height were observed for L3, L4, L16, and L24 while significant positive GCA effects were observed for L11. Inbred lines L2, L5 and L23 showed highly significant negative line GCA effects for leaf senescence while L9, L11 and L20 had significant positive GCA effects for this trait. Inbred lines L20 had positive highly significant GCA effects for number of rows per ear but negative and significant for L15. For thousand-kernel weight, L5 and L14 showed significant positive GCA effects. Worku et al. (2008) reported similar results for these traits.
Across environments combined analysis, fourteen inbred lines showed positive GCA effects for grain yield only one inbred line L20 (1.21 tha-1) showed positive and significant GCA effects indicating the potential advantage of the inbred lines for the development of high-yielding hybrids. Among the testers (males), none of them showed significant GCA effects for grain yield per hectare (Table 9). Results of the current study are in accordance with the findings of Shushay et al. (2013), Kamara et al. (2014), Tamirat et al. (2014), Amare et al. (2016), Abakemal et al. (2016) and Assefa et al. (2017) who reported significant positive and negative GCA effects for grain yield in maize germplasm. Lines with positive GCA effects for grain yield can be extensively used in hybridization program as they contribute favorable alleles in the development of high yielding varieties.
Estimates of specific combining ability effects
The specific combining ability effects at individual and across locations were computed for traits that showed significant SCA mean squares in combining ability analysis. At Ambo optimum N, 50% of the crosses showed positive SCA effects for grain yield out of which two crosses, namely; L3 × T1 and L7 × T2 (Table 8) showed positive and significant SCA effects for grain yield with SCA values of 1.9 and 1.67 t/ha, respectively, indicating that these crosses were good specific combinations for grain yield. In combined analysis across environments two crosses, L7×T2 and L5×T2 (Table 9) showed positive and significant SCA effects for the trait. Crosses with the higher value of SCA effect also showed higher values of mean grain yield performance, indicating good correspondence between SCA effects and mean grain yield. Hence, such cross combinations could effectively be exploited in hybrid breeding program in maize research. On the other hand, two cross combinations L3 × T2 and L7 × T1 expressed negative and significant SCA effects for grain yield under optimum condition, which are undesirable as these crosses showed a tendency to reduce grain yield performance. The finding of the current study is in agreement with that of Mohamed et al. (2014) who reported significant positive and negative SCA effects for grain yield in line × tester analysis experiment under two nitrogen fertilizer levels. Mafouasson et al. (2017) who reported significant positive and negative SCA effects for grain yield in study 42 tropical maize inbred lines for grain yield and yield related traits under low and optimal N conditions.
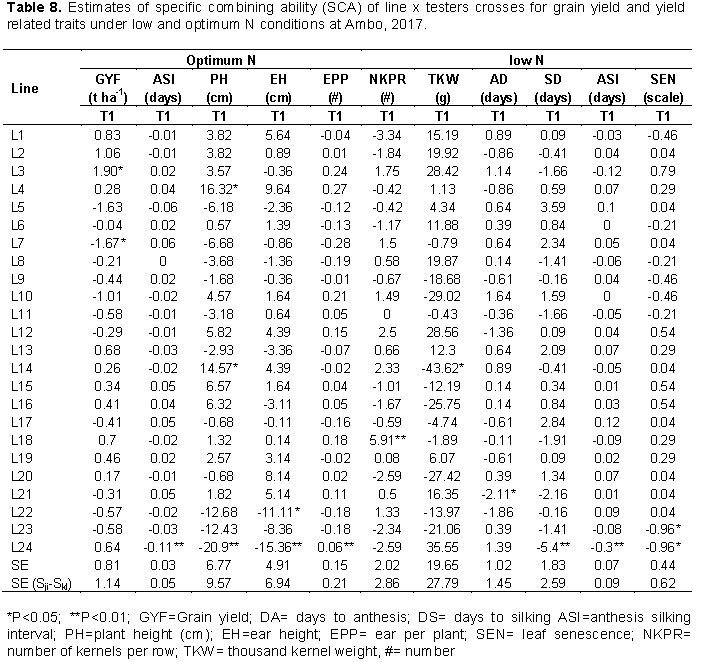
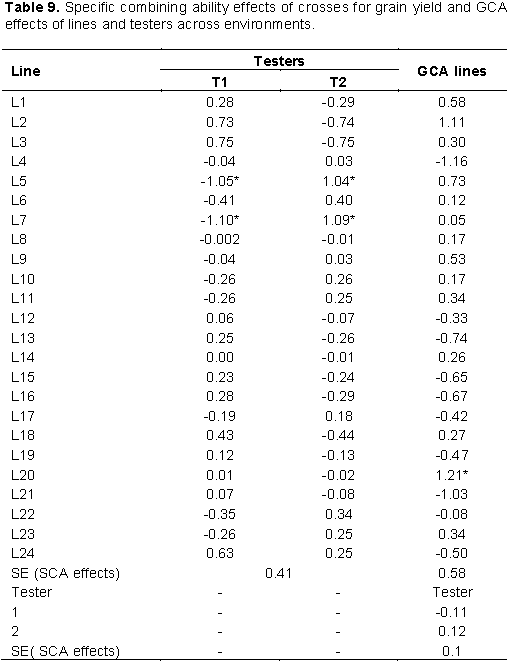
At Ambo low N, cross L21×T2 (-2.11) showed significantly negative SCA effects for days to anthesis and cross L24×T1 (-5.41) showed highly significantly negative SCA effects for days to silking and Anthesis silking interval. Which were desirable for earliness (Table 8). For plant height, three crosses (L24 × T1, L4×T2 and L14×T1) under optimum N condition (Table 8) showed negative and significant SCA effects for the trait, indicating that these crosses had good specific combination for shorter plant stature. For ear height, crosses L24 × T1 and L22 × T1 under optimum N condition (Table 8) showed negative and significant SCA effects for the trait. For thousand-kernel weight, one cross (L14×T2) with SCA values of 43.62 at Ambo (optimum N) was good combinations for thousand-kernel weight as they showed positive and significant SCA effects for this trait. In line with the present results (Melkamu, 2013). Shushay et al. (2013) and Tolera et al. (2017) found significantly positive and negative SCA effects for plant and ear height.
The current study was conducted with the objective of estimating the general and specific combining abilities of highland maize inbred lines and mode of gene action using line × tester mating design. Fifty maize hybrids including 48 testcrosses developed by crossing 24 elite maize inbred lines with two testers and two standard checks were planted at Ambo (low and optimum N) during the 2017 cropping season in alpha lattice design replicated twice. Data were recorded on grain yield and agronomic traits. Analysis of variance indicated hybrids exhibited highly significant (P<0.01) differences in most traits under low and optimum N conditions except number of kernels per row under low N. Mean squares due to crosses were significant for all traits in both environments except ear per plant under low N stress condition. Among the crosses, L2 × T1 (10.76) t ha-1 showed high yield at Ambo (optimum N). At Ambo low N, L5 × T2 (4.61 t ha-1), L6 × T2 (4.37 t ha-1), L14 × T2 (4.31 t ha-1), L20 × T2 (4.14 t ha-1), and L23 × T2 (4.11 t ha-1) were crosses with high yield.
Combining ability analysis is important in identifying the best parents or parental combinations for a hybridization program. Line GCA and SCA mean squares where significant for most traits at each and across environments except SCA at low N stress environment, which is concluded that non-additive gene effects are less important for the inheritance of characters under low N stress condition. Significant GCA and SCA mean squares for most traits measured indicated that both additive and non-additive gene actions are important in determining the inheritance of these traits. General combining ability sum of squares component was greater than SCA sum of squares for all of the studied traits, suggesting that variations among crosses were mainly due to additive rather than non-additive gene effects; and hence, selection would be effective in improving grain yield and other agronomic traits. Based on combining ability analysis, L20, L2, L1 and L9 were found to be the best general combiners for grain yield at optimum N environments whereas L5 and L14 were best general combiners under low N stress condition. Inbred lines with a high GCA effect for grain yield are desirable for synthetic and open pollinated varieties development as well as for inclusion in breeding program. For grain yield, crosses L3×T1 and L7×T2 had good specific combining ability for optimum N location. These hybrids could be included for further studies for the improvement of grain yield and related traits.
The authors have not declared any conflict of interests.
REFERENCES
Abakemal D, Shimelis H, Derera J (2016). Analyses of Combining Ability and Genotype-By-Environment Interaction of Quality Protein Maize Inbred Lines Adapted to Tropical-Highlands. Crop Science 56:3078-3089.
Crossref
|
|
Abate T, Fisher M, Abdoulaye T, Kassie GT, Lunduka R, Marenya P, Asnake W (2017). Characteristics of maize cultivars in Africa: How modern are they and how many do smallholder farmers grow? Agriculture and Food Security 6(1):30.
Crossref
|
|
|
Acquaah G (2007). Principles of plant genetics and breeding. Blackwell Publishing Ltd., pp. 485-497.
|
|
|
Amare S, Dagne W, Sintayehu A (2016). Test Cross Performance and Combining Ability of Elite Highland Maize (Zea Mays L.) Inbred Lines at Jimma, Southwest Ethiopia. International Journal of Trend in Research and Development 3(2):2394-9333.
|
|
|
Assefa T, Zeleke H, Afriye T, Otyama P (2017). Line ×Tester Analysis of Tropical High Land Maize (Zea mays L.) Inbred Lines Top Crossed with Three East African Maize Populations. American Journal of Plant Sciences 8:126-136.
Crossref
|
|
|
Banziger M, Lafitte HR (1997). Efficiency of secondary traits for improving maize for low nitrogen target environments. Crop Science 37:1110-1117.
Crossref
|
|
|
Banziger M, Vivek BS (2007). Fieldbook Software for managing a maize breeding programme. CIMMYT 7:1103-1109.
|
|
|
Banziger M, Betrán FJ, Lafitte HR (1997). Efficiency of high nitrogen selection environments for improving maize for low nitrogen target environments. Crop Science 37:1103-1109.
Crossref
|
|
|
Beck DL, Vasal SK, Crossa V (1990). Heterosis and combining ability of CIMMYT's tropical early and intermediate maturity maize germplasm. Maydica 35:279-285.
|
|
|
Benti T (1992). The need and objectives of the first national maize workshop. pp.3. In Benti T, Joel KR (eds.), The first national workshop, May 5-7th 1992. Addis Ababa, Ethiopia.
|
|
|
Bolaños J, Edmeades GO (1996). The importance of the anthesis-silking interval in breeding for drought tolerance in tropical maize. Field Crops Research 48:65-80.
Crossref
|
|
|
Bullo NT, Dagne W (2016). Combining ability of inbred lines in quality protein maize (QPM) for varietal improvement. International Journal of Plant Breeding and Crop Science 3(1):079-089.
|
|
|
Chandel U, Mankotia BS (2014). Combining Ability in Local and Cimmyt Inbred Lines of Maize (Zea mays L.) for Grain Yield and Yield Components Using Line × Tester Analysis. SABRAO Journal of Breeding and Genetics 46(2):256-264.
|
|
|
CSA (2017). Agricultural Sample Survey 2016/2017: Report on area and production of major crops (private peasant holdings, 'Meher' season). Statistical Bulletin. Vol. 1. CSA, Addis Ababa, Ethiopia.
|
|
|
Dabholkar AR (1999). Elements of biometrical genetics. Concept Publishing Company, New Delhi, India.
|
|
|
Dagne W (2008). Genotypic variability and combining ability of quality protein maize inbred lines under stress and optimal conditions. Doctoral Dissertation, University of the Free State, South Africa.
|
|
|
Demissew A, Habtamu Z, Kanuajia KR, Dagne (2011). Combining ability in maize lines for agronomic traits and resistance to weevil. Ethiopian Journal of Agricultural Science 2:37-48.
|
|
|
Demissew A, Shimelis H, John D, Mark L (2013). Farmers' Perceptions of Maize Production Systems and Breeding Priorities, and Their Implications for the Adoption of New Varieties in Selected Areas of the Highland Agro-Ecology of Ethiopia. Journal of Agricultural Science 5:11.
Crossref
|
|
|
Girma C. Hosana, Sentayehu A, Berhanu T, Temesgen M (2015). Test Cross Performance and Combining Ability of Maize (Zea Mays L.) Inbred Lines at Bako, Western Ethiopia. Global Journal of Science Frontier Research Agriculture and Veterinary 15:4-6.
|
|
|
Gudeta N (2007). Heterosis and combining abilities in QPM versions of early generation highland maize (Zea mays L.) inbred lines. MSc. Thesis. School of Graduate studies, Alemaya University, Ethiopia.
|
|
|
Gomez AK, Gomez AA (1984). Statistical Procedure for Agricultural Research (2nd ed.), John Wiley and so. New York.
|
|
|
Huffnagel HP (1961). Agriculture in Ethiopia. Rome. FAO. Kassie M, Teklewolde H, Jaleta M, Marenya P, Erenstein O (2015). Understanding the adoption of a portfolio of sustainable intensification practices in eastern and southern Africa. Land Use Policy 42:400-411.
Crossref
|
|
|
Kanagarasu S, Nallathambi G, Ganesan KN (2010). Combining ability analysis for yield and its component traits in maize (Zea mays L.). Electronic Journal of Plant Breeding 1(4):915-920.
|
|
|
Kempthorne O (1957). An introduction to genetic statistics. John Wiley, New York.
|
|
|
Lafitte HR, Edmeades GO (1994). Improvement for tolerance to low soil nitrogen in tropical maize. I. Selection criteria. Field Crops Research 39:1-14.
Crossref
|
|
|
Mafouasson AHN, Kenga R, Gracen V, Yeboah AM, Mahamane NL, Tandzi NL, Ntsomboh-Ntsefong G (2017). Combining Ability and Gene Action of Tropical Maize (Zea mays L.) Inbred Lines under Low and High Nitrogen Conditions. Journal of Agricultural Science 9(4):222-235.
|
|
|
McCann JC (2005). Maize and grace. Africa's encounter with a new world crop, 1500-2000. Cambridge. Harvard University Press.
Crossref
|
|
|
Melkamu E (2013). Estimation of combining ability and heterosis of quality protein maize inbred lines. African Journal of Agricultural Research 8(48):6309-6317.
|
|
|
Mohamed MK, Ibrahim SE, Hiroyuki K (2014). Estimation combining ability of some maize inbred lines using line × tester mating design under two nitrogen levels. Australian Journal of Crop Science 8(9):1336-1342.
|
|
|
Patterson HD, Williams ER (1976). A new class resolvable incomplete block designs. Biometrika 63(1):83-92.
Crossref
|
|
|
Presterl T, Seitz G, Landbeck M, Thiemt EM, Schmidt W, Geiger HH (2003). Improving nitrogen-use efficiency in European maize. Estimation of quantitative genetic parameters. Crop Science 43:1259-1265.
Crossref
|
|
|
SAS Institute, Inc (2002). SAS proprietary Software. SAS Institute, Inc, CARY, NC, Canada.
|
|
|
Shushay WA, Habtamu Z, Dagne WG (2013). Line x tester analysis of maize inbred lines for grain yield and yield related traits. Asian Journal of Plant Science and Research 3(5):12-19.
|
|
|
Talukder MZA, Karim ANMS, Ahmed S, Amiruzzaman M (2016). Combining ability and heterosis on yield and its component traits in maize (Zea mays L.). Bangladesh Agricultural Research 41(3):565-577.
Crossref
|
|
|
Tamirat T, Alamerew S, Wegary D, Menamo T (2014). Test Cross Mean Performance and Combining Ability Study of Elite Lowland Maize (Zea mays L.) Inbred Lines at Melkassa, Ethiopia. Advances in Crop Science and Technology 2:140.
|
|
|
Tolera K, Mosisa W, Habtamu Z (2017). Combining ability and heterotic orientation of mid altitude sub-humid tropical maize inbred lines for grain yield and related traits. African Journal of Plant Science 11(6):229-239.
Crossref
|
|
|
Twumasi A, Habtamu Z, Kassa Y, Bayisa A, Sewagegne T (2002). Development and improvement of highland maize in Ethiopia. Proceeding of the Second National Maize workshop of Ethiopia. 12-16 November, 2001. Addis Ababa, Ethiopia pp. 31-37.
|
|
|
United States Department of Agriculture (USDA) (2015). Global Agricultural Information Network. Foreign Agricultural Service Grain and Feed Annual Report Grain and Feed Annual ET-1503, Ethiopia.
|
|
|
United States Department of Agriculture (USDA) (2018). World agriculture production.
|
|
|
Worku M, Banziger M, Friesen D, Schulte G, Auf'm Erley WJ, Horst BSV (2008). Relative importance of general combining ability and specific combining ability among tropical maize (Zea mays L.) inbreds under contrasting nitrogen environments. Maydica 53:279-288
|
|
|
Ziggiju M, Habtamu Z, Legesse W (2016). Standard heterosis of pipeline maize (Zea mays L.) hybrids for grain yield and yield related traits at Pawe, Northwestern Ethiopia. International Journal of Plant Breeding and Crop Science 3(2):6-8.
|
|