ABSTRACT
Good agricultural practices are an effective means of minimizing pre-harvest aflatoxin contamination in peanuts. A field experiment was conducted to evaluate the effect of gypsum on pod yield and aflatoxin contamination in three peanut cultivars (Kadononga, MGV 4 and MGV 5) in Zambia. The experiment was conducted in Chongwe and Lusaka districts. Gypsum (15.6 % calcium) was applied at rates of 0 and 400 kg/ha at flowering stage. Although gypsum had no significant effect on aflatoxin contamination, there were significant differences (p = 0.009) in cultivar susceptibility to aflatoxin contamination. The cultivar with the smallest kernels had 18.8% lower aflatoxin content than the large-kernelled cultivar. Additionally, gypsum did not have a clear effect on pod yield. For instance, gypsum was associated with 44.8% more grain-filled pods in Kadononga (p = 0.005) at the site in Lusaka, but this result did not apply to the other two cultivars. At the site in Chongwe, gypsum was associated with 34.6% higher pod yield of MGV 5 only (p = 0.006). These results further suggest that plant factors such as kernel size may have an influence on natural resistance to aflatoxin contamination in peanuts.
Key words: Aflatoxin, gypsum, peanut cultivar, pod-yield, Zambia.
The prevalence of high aflatoxin contamination in peanuts is a recurrent problem in most tropical climates including Zambia (Njoroge et al., 2017). This has prompted concerted efforts to combat aflatoxin contamination at various stages of the peanut value chain. In the pre-harvest stages, there is need to implement good agricultural practices to minimize contamination during crop growth. Measures that minimize plant stress especially during the pod development stage are recommended so as to minimize colonization of pods by toxigenic Aspergillus fungi and subsequent aflatoxin contamination in kernels (Waliyar et al., 2013; Torres et al., 2014).
One of the critical elements in the development of sound groundnut pods and kernels is calcium (Cox et al., 1976; Jain et al., 2011). Well-developed mature pods are not easily perforated by insects and this minimizes the entry of fungi into the seed tissue. It is on this principle that calcium-containing soil amendments such as gypsum are used to minimize pre-harvest aflatoxin contamination in peanuts (Reding et al., 1993; Gebreselassie et al., 2014). Additionally, amending soils with gypsum at the rate of 250 kg/ha was associated with higher grain yields compared with the control (Bairagi et al., 2017). According to Kabir et al. (2013) amending the soil with gypsum as a source of calcium resulted in higher number of pods per plant and 100 pod weights. Therefore, sufficient calcium fertilization in peanuts can both minimize aflatoxin contamination and increase kernel yield.
However, cultivar response to calcium inputs partly depends on kernel size. Large seeded cultivars require higher inputs of calcium than small-seeded kernels (Jordan et al., 2014). The objective of this study was to assess the effect of gypsum amendment on kernel yield and pre-harvest aflatoxin contamination on aflatoxin-susceptible cultivars. Pod-yield and aflatoxin contamination in three peanut cultivars of Zambia were evaluated following a gypsum amendment on two soils with contrasting exchangeable calcium content.
Location and soil properties
A field experiment was conducted at the University of Zambia (UNZA), Field Research Station (15° 28.646’ S, 28° 20. 278’ E) in Lusaka district and at Kasisi Agricultural Training Centre (KATC15°14.989’ S, 28° 29.013’ E) in Chongwe district. The experiment was done under rain-fed conditions from mid-December 2016 to April 2017. Both research sites are situated in the agro-ecological region II of Zambia with mean annual rainfall ranging from 800 to 1000 mm (Soil Survey Branch, 2002). The two research sites were characterised by soil with contrasting chemical properties in terms of soil pH and exchangeable calcium content. The soil at KATC was characterised by strong acidity (pH = 4.22) and very low exchangeable calcium (0.06 cmol/kg) while the soil at UNZA had near neutral pH of 6.98 and high exchangeable calcium content of 5.14 cmol/kg. It should be noted that most acidic soils are associated with calcium deficiency (Brady and Weil, 2010).
Treatments and experimental design
The treatments in the experiment were two rates of gypsum and three cultivars. The cultivars were: MGV 5, MGV 4 and Kadononga. The chosen cultivars were among the most popular ones among small-holder farmers and seed companies. MGV 5 and MGV 4 were among the most common commercial cultivars while Kadononga was among the most common local landrace mostly preferred for its early maturity and ‘tasty’ kernels. All the three cultivars have a
bunch type growth habit. MGV 5 and MGV 4 are Virginia market types taking between 120 and 130 days to physiological maturity with potential yields of 2.5 to 3.0 metric ton/ha and 1.5 to 2.5 metric ton/ha, respectively. Kadononga is a Spanish type that takes between 90 and 100 days to maturity with a yield potential of 1.0 to 1.5 metric ton/ha. In terms of seed size, MGV 5 is large-seeded; MGV 4 is medium-seeded while Kadononga is a small-seeded cultivar. The treatments were laid out in split-plot design with gypsum as the main plot factor and cultivar as the sub-plot factor, respectively. The experimental plots measured 4 m by 4 m with a 1 m isle between plots. Each factor was replicated thrice resulting in 18 experimental plots. Gypsum was applied on the soil surface covering the entire plant row span at flowering stage rates of 0 and 400 kg/ha. The time of application and the rate of 400 kg/ha of gypsum were adopted from the literature (Waliyar et al., 2013).
Seeding and field management
Seedbed preparation was done by ploughing with a tractor-mounted disc plough followed by levelling using a disc harrow. Seeding was done on a flat seedbed in 5 cm-deep planting holes made using a hand-hoe. The recommended seeding rate for each cultivar was followed. The fields were kept weed, pest and disease-free throughout the growing season. Weeds were uprooted by hand or dug-out using a hoe just as soon as they appeared. Appropriate pesticides and fungicides were sprayed regularly to control pests and fungal infections, respectively.
Harvesting and determination of pod-yield
Harvesting of peanut pods was done at physiological maturity. Plants were dug out using a hand hoe. Pod yield was determined by counting grain-filled pods from each of the 6 randomly selected plants from the middle plant rows of each experimental plot. The pods were then dried in an electric vacuum oven (Hereanus, Germany) set at 45°C to a gravimetric moisture content of 10%. The dried pods were then shelled by hand and prepared for aflatoxin testing.
Sampling and aflatoxin analysis
One third of the total kernel yield per treatment constituted the laboratory sample. The sample was constituted by aggregating several 100 g scoops from a single bulk sample. The bulk sample was shaken after each 100 g sub-sample was taken. Duly constituted laboratory samples were then ground into fine flour using an ordinary kitchen grinder (LM2211BM, Moulinex, China). Ground samples were homogenized by thorough shaking. Total aflatoxin content in the flour was extracted using 65% ethanol reconstituted from an original product (UN1170, Xilong Scientific Co., Shantou City, China) with a concentration of 95%. For each treatment, three sub-samples each weighing 10.0 g were mixed with 30 ml of ethanol and shaken on a rotary shaker (ISO-9001-2000, Navyug, India) at 120 rpm for 3 min. After shaking, the mixture was filtered through Whitman 42 filter paper. Total aflatoxin concentration in each sample was determined using Neogen Afla Reveal® Q+ aflatoxin kit (Neogen Corporation, USA). The lower and upper limits of detection of aflatoxin concentration were 1 and 50 µg/kg, respectively. All the tested samples had total aflatoxin concentrations within detection limits.
Statistical analysis
Data on pod yield and aflatoxin content were subjected to the analysis of variance test at 95% confidence interval. The separation of statistically significant treatment means was done using Fisher’s protected Least Significant Difference.
Effects of gypsum on pod-yield in selected peanut cultivars
Gypsum applied at flowering stage of peanuts resulted in higher number of grain-filled pods only in selected cultivars (Table 1). Although gypsum was associated with higher number of pods for Kadononga at UNZA, no similar result was observed at KATC. Similarly, gypsum was associated with a significantly higher number of grain-filled pods for MGV 5 at KATC and not at UNZA. MGV 4 did not show any response to gypsum at both sites. In general, these results are contrary to literature (Cox et al., 1976; Jain et al., 2011; Jordan et al., 2014; Bairagi et al., 2017) that report higher yields due to calcium-containing inputs such as gypsum. According to Kabir et al. (2013) peanut plants fertilized with calcium inputs recorded higher 100 pod weights compared with plants that did not receive a calcium input. As earlier noted by Smith et al. (1993) calcium is an essential element for pod filling in peanuts and a lack of it is reported to cause fruit abortions, resulting in fewer grain-filled pods.
According to Cox et al. (1976), peanut response to calcium-containing inputs is dependent on soil characteristics such as the exchangeable calcium content. Recommendations must therefore consider soil type and in particular the native calcium content at which additional amounts would trigger a response. The choice of 400 kg/ha in the current study was based on the recommendation by Waliyar et al. (2013). The poor response to gypsum observed in the study suggests that the chosen rate of gypsum application may not be adequate for the soil types at the two sites.
The observed result in this study could be attributed to the native exchangeable calcium content of the soil at the two experimental sites. Although the soil at Kasisi had low exchangeable calcium, it was still able to meet the calcium requirements for Kadononga and MGV 4. As for MGV 5 at the same site, the positive response to gypsum input could signify the need for extra calcium for optimal growth. In the case of the soil at UNZA that had high native exchangeable calcium content, the logical expectation would be that the small-kernelled Kadononga would not respond to additional calcium, unlike the larger-kernelled MGV 4 and MGV 5. On the contrary, only the small-seeded Kadononga responded, making it difficult to attribute the response to gypsum amendment.
Additionally, differences in plant nutrient and moisture requirements between cultivars have an effect on crop performance. For instance, small-kernelled peanut cultivars have a lower nutrient and soil moisture requirement than larger ones (Jordan et al., 2014). Thus, if there is a limited supply of nutrients, especially exchangeable calcium and plant-available-water in the soil, the small-kernelled cultivars would grow normally under given soil conditions while the larger-kernelled cultivars would need external inputs.
Effect of gypsum and cultivar on total aflatoxin content in kernels at harvest
Results from the study showed that the gypsum amendment had no effect on total aflatoxin content in kernels (p > 0.05). In contrast, other authors such as Reding et al. (1993) and Gebreselassie et al. (2014) reported decreased aflatoxin content in peanut kernels due to gypsum amendment. Nonetheless, there were significant differences (p < 0.01) in mean total aflatoxin content in the three cultivars (Figure 1). The aflatoxin content varied according to kernel size. The cultivar with smallest kernel size was the least contaminated. This pattern was observed at both experimental sites. The mean total aflatoxin concentrations across the two sites were 10.1, 10.8 and 12 ppb for Kadononga, MGV 4 and MGV 5, respectively, in the same order as their kernel size starting with the smallest to the largest. Although the aflatoxin contamination in all the cultivars was within the permissible limits of less than 15 ppb according to the Zambia Bureau of Standards ZS 723 safety standard, the result in this study suggests that more effort is needed to manage aflatoxin contamination in larger-kernelled cultivars than in smaller ones.
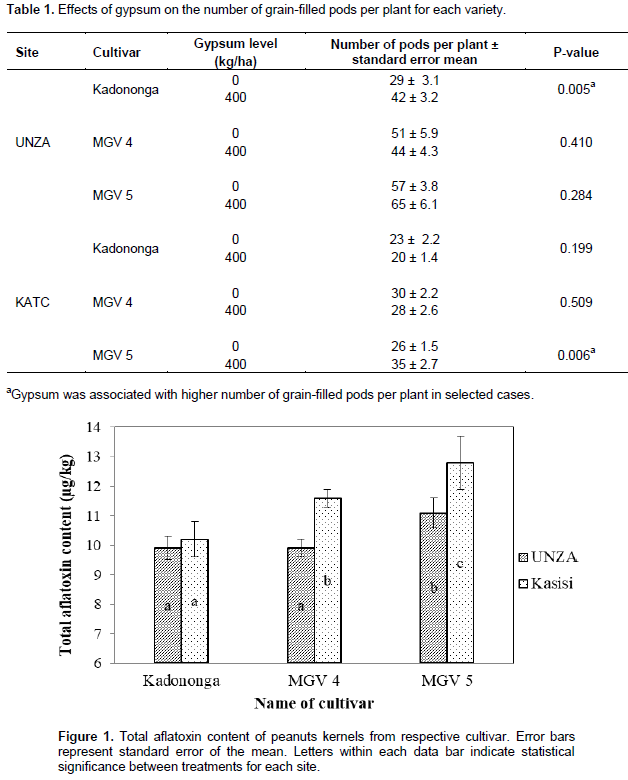
Soil moisture status assessed in terms of mean daily rainfall received during the pod-development phase of the crop did not show significant differences (p > 0.05) across cultivars at each of the two sites. Therefore, the observed differences in mean total aflatoxin levels cannot be explained by the moisture status. Reding et al. (1993) attributed the reduction in aflatoxin contamination following the application of gypsum amendment to inherent plant factors. Similarly, results from the current study suggest that there could be plant factors such as pod strength that may influence aflatoxin contamination. According to Waliyar et al. (2013), well-developed mature pods tend to be less susceptible to fungal infection and subsequent aflatoxin contamination than immature and weak pods. In a study involving ten peanut genotypes, it was reported that although all the studied cultivars supported the growth of Aspergillus flavus, one cultivar recorded significantly less aflatoxin contamination (< 20 ppb) regardless of the amount of fungal accumulation (Korani et al., 2017). This result may suggest that some peanut genotypes are naturally more resistant to aflatoxin contamination than others.
Gypsum at the rate of 400 kg/ha did not have a clear influence on pod yield and no significant effect on total aflatoxin contamination in harvested kernels. However, this study showed significant differences in cultivar susceptibility to aflatoxin contamination. For the three cultivars in the study, results showed a negative relationship between pre-harvest aflatoxin content and kernel size indicating that inherent factors such as kernel size may have a role in determining aflatoxin resistance. Further, it can be concluded that management of aflatoxin contamination in larger-kernelled cultivars requires more effort than in smaller ones.
The authors have not declared any conflict of interests.
This study was funded by the Peanut and Mycotoxin Innovation Laboratory under the Southern African Value Chain Project through the U.S. Agency for International Development, under the terms of Award No. AID-ECG-A-00-07-0001 to The University of Georgia as management entity for the U.S. Feed the Future Innovation Lab on Peanut Productivity and Mycotoxin Control.
REFERENCES
Bairagi MD, David AA, Thomas T, Gurjar PC (2017). Effect of different level of N P K and gypsum on soil properties and yield of groundnut (Arachis hypogaea L.) var. Jyoti. International Journal of Current Microbiology and Applied Sciences 6(6):984-991.
Crossref
|
|
Brady NC, Weil RR (2010). Elements of the nature and properties of soils. 3rd ed. Prentice Hall, Upper Saddle River, New Jersey, USA.
|
|
|
Cox FR, Sullivan GA, Martin CK (1976). Effect of calcium and irrigation treatments on peanut yield, grade and seed quality, Peanut Science 3:81-85.
Crossref
|
|
|
Gebreselassie R, Dereje A, Solomon H (2014). On-farm pre harvest agronomic management practices of Aspergillus infection on groundnut in Abergelle, Tigray. Journal of Plant Pathology and Microbiology 5(2):1-6.
Crossref
|
|
|
Jain M, Pathak BP, Harmon AC, Tillman BL, Gallo M (2011). Calcium dependent protein kinase (CDPK) expression during fruit development in cultivated peanut (Arachis hypogaea) under Ca2+- sufficient and -deficient growth regimens. Journal of Plant Physiology 168(18):2272-2277.
Crossref
|
|
|
Jordan DL, Brandenburg RL, Brown BA, Bullen GS, Roberson GT, Shew B (2014). Peanut Information. North Carolina Cooperative Extension Service, College of Agriculture and Life Sciences, North Carolina State University, NC, USA.
|
|
|
Kabir R, Yeasmin S, Islam AKMM, Sarkar MAR (2013). Effect of phosphorus, calcium and boron on the growth and yield of groundnut (Arachis hypogea L.), International Journal of Bio-Science and Bio-Technology 5(3):51-60.
|
|
|
Korani WA, Chu Y, Holbrook C, Clevenger J, Ozias-Akins P (2017). Genotypic regulation of aflatoxin accumulation but not Aspergillus fungal growth upon post-harvest infection of peanut (Arachis hypogaea L.) seeds. Toxins 9(7):218.
Crossref
|
|
|
Njoroge SMC, Matumba L, Kanenga K, Siambi M, Waliyar F, Maruwo J, Machinjiri N, Monyo ES (2017). Aflatoxin B1 levels in groundnut products from local markets in Zambia. Mycotoxin Research 33(2):113-119.
Crossref
|
|
|
Reding CLC, Harrison MA, Kvien CK (1993). Aspergillus parasiticus growth and aflatoxin synthesis on florunner peanuts grown in gypsum-supplemented soil. Journal of Food Protection 56(7):593-611.
Crossref
|
|
|
Smith DH, Wells MA, Porter DM, Cox FR (1993). Nutrient deficiencies and toxicities in crop plants', In: Peanuts, APS Press pp. 193-247.
|
|
|
Soil Survey Branch (2002). Agro-ecological map of Zambia. Republic of Zambia, Ministry of Agriculture, Mt. Makulu Research Station, Department of Agriculture, Private Bag 7, Chilanga, Zambia.
|
|
|
Torres AM, Barros GG, Palacios SA, Chulze SN, Battilani P (2014). Review on pre- and post-harvest management of peanuts to minimize aflatoxin contamination. Food Research International 62:11-19.
Crossref
|
|
|
Waliyar F, Osiru M, Sudini HK, Njoroge SMC (2013). Aflatoxins: Finding solutions for improved food safety-Reducing Aflatoxins in Groundnuts through Integrated Management and Biocontrol. International Food Policy Research Institute, 2033 K Street, NW, Washington, DC 20006-1002 USA.
|
|