ABSTRACT
Salinity negatively influences the physiology and productivity of plants which develop different strategies to resist to this stress. This study aims to evaluate the implication of sodium (Na), potassium (K), proline and soluble sugars accumulation in salt resistance of okra local cultivars after two weeks exposure to 0, 30, 60, 90 and 120 mM NaCl concentrations. Results revealed that the aerial part growth reduction under salt stress was more accentuated in the salt sensitive cultivar Keleya than the salt resistant Yodana. Na+ accumulation in leaves was more accentuated in Keleya than Yodana whereas proline accumulation was more accentuated in both leaves and roots of Yodana than Keleya. K+ content decrease was more accentuated both in leaves and roots of Keleya than Yodana. Consequently, the decrease in ionic selectivity ratio (K/Na) was more accentuated in the salt sensitive cultivar Keleya than the salt resistant Yodana in both leaves and roots. Soluble sugars accumulation in leaves depends on the NaCl concentration. Results indicated that the relative salinity resistance of cultivar Yodana is associated with sodium ions exclusion from leaves, the maintaining of good accumulation of potassium ions and a good K+/Na+ selectivity ratio, and the accumulation of high amounts of proline.
Key words: Abelmoschus species, NaCl, sodium, potassium, soluble sugars, proline.
Salt stress is one of the major environmental constraints limiting agricultural productivity (Wei et al., 2003). Salinity is the buildup of soluble salts by which saline soils are formed (Levy and Syvertsen, 2004). It was established in several studies that plant growth is compromised by salinity at all stages of development, but sensitivity varies greatly at different stages (Akram et al., 2002; Akinci et al., 2004; Gandonou and Senhaji, 2015; Loko et al., 2020).
Gama et al. (2007) reported that plants growing under saline conditions are affected in three ways: reduced water potential in root zone causing water deficit, phytotoxicity of ions such as Na+ and Cl- and nutrient imbalance (Ashraf and Foolad, 2007) depressing uptake and transport of nutrients. In saline environment, plant growth is affected by complex interaction of hormones, osmotic effects, specific ion effects and nutritional imbalances, probably all occur simultaneously (Arbona et al., 2005). Although most, if not all crop plants are glycophyte species, their overall responses to increased natrium chloride (NaCl) dose appear to be species specific (Levitt, 1980; Lutts et al., 1995; Karikalan et al., 1999; Lakra et al., 2006; Chukwu and Okpe, 2006; Gandonou et al., 2012). In addition, within the same given species, a substantial variation in salt sensitivity can appear in cultivars (Gandonou et al., 2012; Abbas et al., 2014; Sounou et al., 2021). This difference in species or cultivars’ behavior is linked to a number of physiological and biochemical mechanisms developed by plants to survive, grow and produce in the presence of high salt concentrations including sodium ion exclusion, potassium ion maintenance and organic compounds accumulation. Among the compounds involved, there may be mentioned amino acids, in particular proline, soluble sugars, soluble proteins and quaternary ammonium compounds.
Okra (Abelmoschus esculentus L. Moench) is an annual vegetable of the tropical and subtropical areas belongs to family Malvaceae. It is a popular vegetable among both the consumers and farmers because it is rich in vitamins and minerals (Oyelade et al., 2003). Almost all parts of okra plant are consumed, like fresh okra fruits are used as vegetable, roots and stems are used for clearing the cane juice (Chauhan, 1972) and leaves and stems are used for making fiber and ropes (Jideani and Adetula, 1993). Being an excellent source of K, calcium (Ca) and unsaturated fatty acids for instance, linolenic and oleic acid (Arbona et al., 2005), okra is very essential for human nutrition (Khan et al., 2015). Although the area under okra has progressively increased during last few years, there is a decreasing trend in its yield per hectare (Haq et al., 2012). Among identified biotic and abiotic stresses, salinity has been the key factor responsible for yield reduction (Khan et al., 2002).
Despite a considerable amount of research work on plant responses to salt stress, data on okra salt tolerance strategy is scarce. In Asia, some research works studied the salt tolerance of some okra genotypes using several growth parameters (Haq et al., 2012 ; Abbas et al., 2014). Some other works studied the physiological strategy for salt tolerance in Asian okra genotypes (Shahid et al., 2011; Habib et al., 2012; Khan et al., 2015). In addition, there is hardly any work on the effect of salt stress on mineral nutrition, in particular on Na+ and K+ contents and on the accumulation of a number of organic compounds in okra cultivars grown in Benin Republic. In a previous study, it was found that an important variability exists among okra cultivars produced in Benin in term of salinity resistance (Gouveitcha et al., 2021). The main objective of this study was to determine the physiological strategy developped by salt resistant okra cultivars grown in Benin to resist, to salt stress related to ion and organic solutes accumulation.
Plant
The experiment focused on two cultivars of okra (Abelmoschus esculentus L. Moench) which exhibited contrasting behaviors towards salinity at the young plant stage. These are the cultivars Yodana which appeared to be a resistant cultivar and Keleya which appeared to be sensitive to NaCl according to Gouveitcha et al. (2021).
Experimental conditions
The experiment was carried out in screening house of the National Institute of Agricultural Research of Benin (INRAB), Benin Republic. The seeds were germinated in tubs filled with potting soil for two weeks. The young plants were then transferred to small pots 5.8 cm in diameter and 6 cm in height containing a mixture of potting soil and sand (50:50) (one plant/pot) and grown for a week before application of the stress. Plants of the two cultivars were subjected to salt stress in large earthen pots (11.3 cm in diameter and 14 cm in height) filled with 3 kg of the same mixture as before. Treatments consisted of watering the plants every other day with 100 ml/pot of 0; 30; 60; 90 or 120 mM NaCl. The experimental set-up as a completely randomized design with two factors. The first factor represents the five (05) saline treatments (T0 = 0 mM; T1 = 30 mM; T2 = 60 mM ; T3 = 90 mM and T4 = 120 mM) and the 2nd is represented by the two (02) okra cultivars (Yodana and Keleya) with three replicates.
Growth determination
Plant height (cm), number of leaves, root length (cm), fresh and dry mass (FM and DM) of the shoots and roots were first determined before application of the salt treatments (X0). They were determined again after 2 weeks of treatment (X1). Relative height growth of plants (RHG) was determined according to the formula: (X1-X0) / X0. The fresh mass of the aerial and roots parts was determined by weighing. The samples from each part were then transferred to an oven at 80°C for 72 h for the determination of the dry mass. Data in the presence of NaCl were expressed in percentage of that of the control.
Extraction and estimation of ion concentrations
For the determination of the ions, the roots were quickly rinsed with distilled water to remove the ions fixed on them and those contained in the apoplasm (Bourgeais-Chaillou and Guerrier, 1992). The leaves and roots were individually dried in an oven at 80°C for 72 h, ground in a mortar, and the powder was dried for 24 h. To determine the concentrations of Na+ and K+, 20 mg of the leaf and root powders were placed in 10 ml jars and digested in nitric acid (68%) at room temperature. The solutions were filtered through Whatman paper (85 mm, Grade 1). The filtrate was used for the determination of cations (Na+ and K+) using a flame spectrophotometer (Sherwood Model 360). The quantities of ions were expressed in mg g-1 of dry matter (dm).
Extraction and determination of proline and soluble sugars
Proline concentration was determined spectrophotometrically using the method of Bates et al. (1973) and results were expressed as μg proline g−1 FM (Fresh Mass).
Total soluble sugars were estimated by the anthrone reagent method using glucose as the standard accords to Yemm and Willis (1954) as used by Manaa et al. (2014) using an UV-visible spectrophotometer (Jenway 7305). Soluble sugars concentration was expressed as mg soluble sugars g−1 FM (Fresh Mass).
Shoot water content
Shoot water content was determined according to the formula:
[(Shoot fresh Mass – shoot dry Mass)/Shoot fresh Mass] × 100
Statistical analysis
The data collected was processed using descriptive statistics using an Excel spreadsheet and presented in the form of tables and graphs. Analysis of cultivar effects and stress intensity was based on one or two-ways analysis of variance (ANOVA) as appropriate. Means were compared using the Student, Newman and Keuls test. Statistical analyzes were performed using JMP Pro 12 software (JMP Pro SAS Institute, 2015).
Plant growth
NaCl stress significantly (p< 0.001) reduced RHG in both cultivars from 90 mM NaCl but the reduction was more accentuated in the salt-sensitive Keleya (average of 20.16%) than the salt resistant Yodana (average of 12.95%) (Table 1). Salt effect induced a reduction in shoot fresh and dry mass in both cultivars but the reduction was more accentuated in the salt sensitive cultivar keleya than the salt resistance Yodana. The average reductions due to NaCl stress were 44.85, 44.16%, for cultivar Keleya and only 24.40 and 26.37% for cultivar Yodana, respectively for shoot fresh mass and shoot dry mass (Table 1). Roots growth also was adversely affected by salt stress in both root fresh and dry mass in both cultivars. However, the reduction was less accentuated in the salt sensitive Keleya than the salt resistant Yodana. The average reductions due to NaCl stress were 37.80 and 35.55%, for cultivar Keleya and 48.39 and 43.32% for cultivar Yodana, respectively for roots fresh mass and roots dry mass (Table 1). Thus, plant aerial part growth reduction by NaCl stress was more accentuated in the salt-sensitive Keleya compared to the salt-resistant Yodana.
Plant water content
NaCl stress induced similar effect on shoot water content in both cultivars characterized by slight non significant decrease (Table 2). Thus, shoot water content did not change significantly under salt stress in both cultivars.
Effects of NaCl on ions accumulation
Effect of NaCl on sodium ion (Na+) content
Salt effect induced a significant (p< 0.001) increase in the leaf Na+ content of both cultivars but this increase was significant from 60 mM NaCl for the sensitive cultivar Keleya whereas it was significant only at 120 mM NaCl for the resistant cultivar Yodana (Figure 1A). Leaf Na+ content passed from 1.478 mg g-1 DM to 1.739, 2.369, 2.391 and 2.521 mg g-1 DM for cultivar Keleya, respectively at 30, 60, 90 and 120 mM NaCl and from 0.978 mg g-1 DM to 1.108, 1.26, 1.478 and 1.63 mg g-1 DM for cultivar Yodana at 30, 60, 90 and 120 mM NaCl, respectively. Thus, salinity induced an increase in sodium content in the leaves in both cultivars but this increase is more marked in sensitive cultivar Keleya compared to the resistant cultivar Yodana. Likewise, Figure 1B shows a significant (P< 0.01) increase in the roots Na+ content of both cultivars but this increase was significant from 30 mM NaCl for the sensitive cultivar Keleya whereas it was significant from 90 mM NaCl for the resistant cultivar Yodana. Root Na+ content passed from 1.956 mg g-1 DM to 2.673, 2.804, 3.195 and 3.347 mg g-1 DM for cultivar Keleya, respectively at 30, 60, 90 and 120 mM NaCl, and from 1.739 to 2.043, 2.565, 2.978 and 3 mg g-1 DM for cultivar Yodana at 30, 60, 90 and 120 mM NaCl, respectively. Thus, salinity induced an increase in sodium content in roots in both cultivars but this increase is more marked in the sensitive cultivar Keleya compared to the resistant cultivar Yodana.
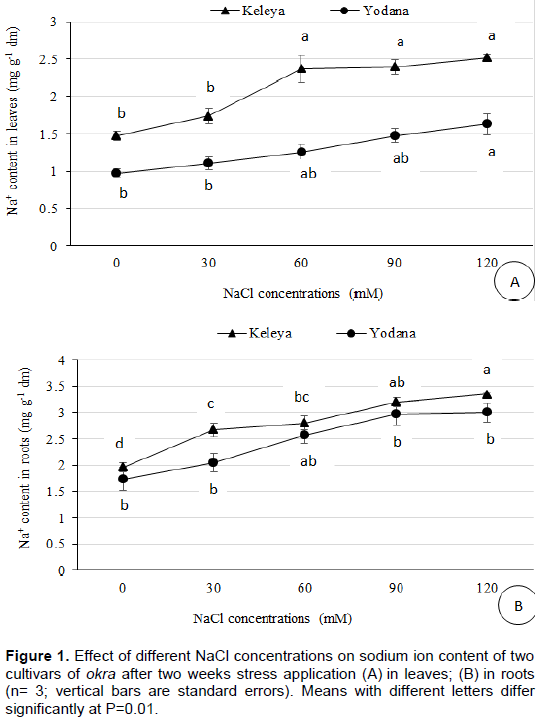
Effect of NaCl on potassium ion (K+) content
Salt effect induced a significant decrease in leaves K+ content of both cultivars but this decrease was significant (P<0.05) from 60 mM NaCl for the sensitive cultivar Keleya whereas it was significant (P<0.01) from 90 mM NaCl for the resistant cultivar Yodana (Figure 2A). Leaf potassium content passed from 725.37 mg g-1 DM to 674.29, 588.47, 557.82 and 504.7 mg g-1 DM for cultivar Keleya, respectively at 30, 60, 90 and 120 mM NaCl, and from 819.37 mg g-1 DM to 729.46, 745.81, 653.86, and 668.16 mg g-1 DM for cultivar Yodana. Thus, salinity induced a decrease in potassium content in leaves in both cultivars but this decrease was more marked in sensitive cultivar Keleya compared to the resistant cultivar Yodana. Likewise, Figure 2B shows a significant (p<0.05) decrease in roots potassium content from 60 mM NaCl in K+ content of both cultivars. Roots potassium content passes from 559.86 mg g-1 DM to 519, 429.10, 390.27, and 302.10 mg g-1 DM for cultivar Keleya and 762.16 mg g-1 DM to 753.98, 604.82, 576.22 and 596.65 mg g-1 DM for cultivar Yodana. Thus, salinity induced a decrease in potassium ion content in roots in both cultivars but this decrease was more marked in the sensitive cultivar Keleya compared to the resistant cultivar Yodana.
The selectivity ratio decreased in both cultivars in leaves and roots as the NaCl concentration increased (Table 3). For the sensitive cultivar Keleya, the decrease was significant (P<0.001) from 60 mM NaCl. For the resistant cultivar Yodana, the decrease was significant (P<0.01) from 90 mM NaCl. Thus, salinity induced a decrease in K+/Na+ selectivity ratio of leaves for both cultivars but this decrease was more marked in a sensitive cultivar Keleya compared to the resistant cultivar Yodana. In roots, the same tendency was observed with a decrease significant (P<0.001) from 30 mM NaCl for the sensitive cultivar Keleya, and significant (P<0.01) from 60 mM NaCl for the resistant cultivar Yodana.
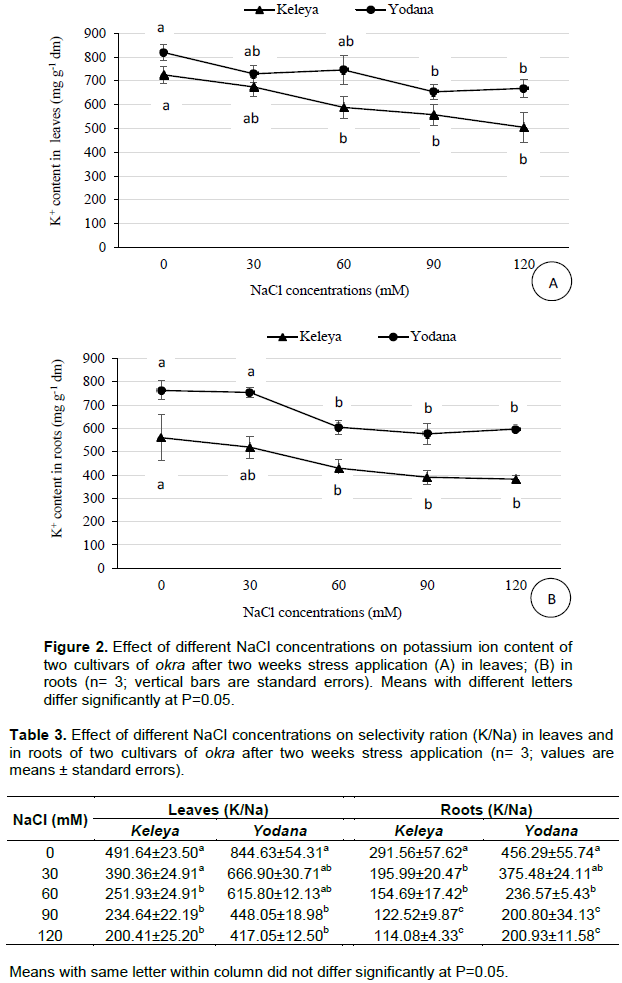
Effect of salt stress on proline content
Salt effect induced a significant (P< 0.001) increase in proline content in leaves of both cultivars but this increase was significant (P< 0.01) only at 120 mM NaCl for the sensitive cultivar Keleya whereas it was significant (p< 0.001) from 90 mM NaCl for the resistant cultivar Yodana (Figure 3A). Proline concentration passed from 0.018 µg g-1 FM to 0.021, 0.021, 0.026, and 0.036 µg g-1 FM for cultivar Keleya, respectively at 30, 60, 90, and 120 mM NaCl, and from 0.010 µg g-1 FM to 0.013, 0.018, 0.046, and 0.067 µg g-1 FM for cultivar Yodana at 30, 60, 90, and 120 mM NaCl, respectively. These increases correspond respectively to 16.66, 16.66, 44.44 and 100% compared to the control for the sensitive cultivar Keleya, and to 30, 80, 360, and 570% for the resistant cultivar Yodana. Thus, salinity induced an increase in proline content in leaves in both cultivars but this increase is much more marked in resistant cultivar Yodana compared to the sensitive cultivar Keleya. Likewise, Figure 3 shows a significant increase in roots proline content of both cultivars but this increase was significant (P< 0.01) only at 120 mM NaCl for the sensitive cultivar Keleya whereas it was significant (P< 0.001) from 60 mM NaCl for the resistant cultivar Yodana. Proline concentration passed from 0.013 µg g-1 FM to 0.019, 0.016, 0.021 and 0.027 µg g-1 FM for cultivar Keleya, respectively at 30, 60, 90, and 120 mM NaCl; and from 0.005 µg g-1 FM to 0.008, 0.019, 0.043 and 0.049 µg g-1 FM for cultivar Yodana at 30, 60, 90, and 120 mM NaCl, respectively. These increases correspond, respectively to 46.15, 23.07, 61.53, and 107.69% compared to the control for the sensitive cultivar Keleya, and to 60, 280, 760, and 880% for the resistant cultivar Yodana. Thus, salinity induced an increase in proline content in roots in both cultivars but this increase is much more marked in the resistant cultivar Yodana compared to the sensitive cultivar Keleya. Moreover, proline accumulation was more marked in roots than leaves in both cultivars.
Effect of salt stress on soluble sugars content
Salt effect induced a significant (P< 0.001) increase in soluble sugars content in leaves of both cultivars but this increase was significant from 30 mM NaCl for the sensitive cultivar Keleya whereas it was significant from 60 mM NaCl for the resistant cultivar Yodana (Figure 4A). Soluble sugars content passed from 0.441 mg g-1 FM to 0.73, 0.717, 1.059, and 1.804 mg g-1 FM for cultivar Keleya, respectively at 30, 60, 90 and 120 mM NaCl, and from 0.291 mg g-1 FM to 0.396, 0.529, 0.763, and 0.647 mg g-1 FM for cultivar Yodana at 30, 60, 90, and 120 mM NaCl, respectively. These increases correspond, respectively to 65.53, 62.58, 140.13 and 309.07% compared to the control for the sensitive cultivar Keleya, and to 36.08, 81.78, 162.19, and 122.33% for the resistant cultivar Yodana. Thus, salinity induced an increase in soluble sugars content in leaves in both cultivars but this increase is more marked in the salt sensitive cultivar Keleya compared to the resistant cultivar Yodana mainly at 30 and 120 mM NaCl. Likewise, Figure 4B shows a significant (P< 0.001) increase in roots soluble sugars content of both cultivars but this increase was significant from 60 mM NaCl for the sensitive cultivar Keleya whereas it was significant from 90 mM NaCl for the resistant cultivar Yodana. Soluble sugars content passed from 0.215 mg g-1 FM to 0.224, 0.514, 0.655, and 0.994 mg g-1 FM for cultivar Keleya, respectively at 30, 60, 90 and 120 mM NaCl, and from 0.135 mg g-1 FM to 0.180, 0.174, 0.190 and 0.310 mg g-1 FM for cultivar Yodana at 30, 60, 90 and 120 mM NaCl, respectively This increase corresponds, respectively to4.18, 139.06, 204.65 and 362.32% compared to the control for the sensitive cultivar Keleya, and to 33.33, 28.88, 40.74 and 129.62% for the resistant cultivar Yodana. Thus, salinity induced an increase in soluble sugars content in roots in both cultivars but this increase is much more marked in the sensitive cultivar Keleya compared to the resistant cultivar Yodana except at 30 mM NaCl.
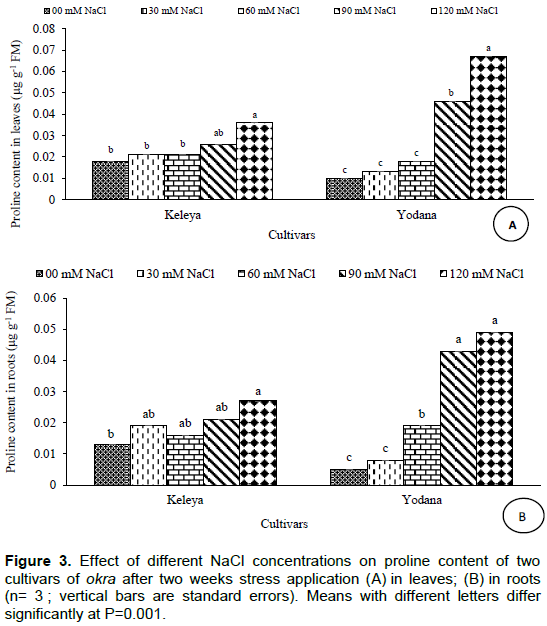
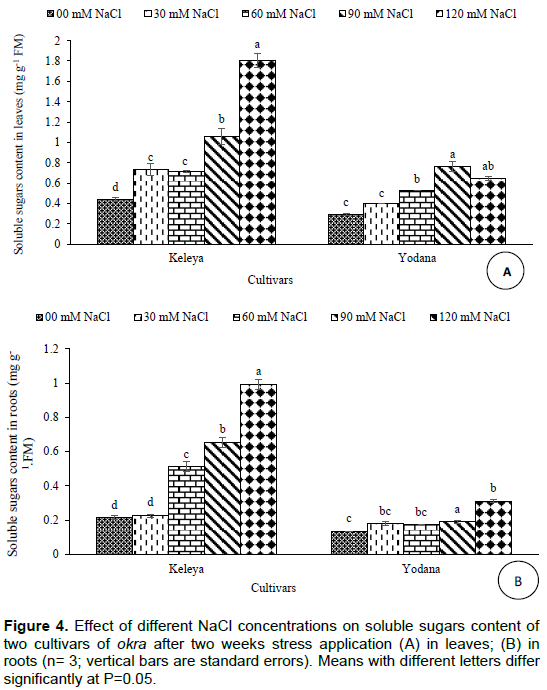
Effect of NaCl on plant growth of okra cultivars
The results revealed that plant growth reduction due to NaCl stress was more accentuated in the salt-sensitive Keleya compared to the salt-resistant Yodana confirming the salt-resistance status of both cultivars as previously reported (Gouveitcha et al., 2021). In other okra genotypes, Abbas et al. (2014) used growth parameters to discriminate salt tolerant genotypes from the salt sensitive one. Salinity classically induced cell dehydration at low water potential, nutritional imbalance caused by the interference of saline ions with essential nutrients in both uptake and translocation processes and toxicity due to the high accumulation of Na+ and Cl- in the cytoplasm. No change in plant water content was observed in our cultivars indicating that water content parameter is not the main aspect of salt stress effect in these cultivars as previously reported in amaranth (Wouyou et al., 2019).
Implications of ion accumulation in the salinity resistance of okra cultivars
The effect of salt stress on plants can induce the following three responses: dehydration of cells through low water potential; nutritional imbalance caused by the interference of salt ions with essential nutrients in both absorption and translocation processes; toxicity due to a high accumulation of Na+ in the cytoplasm and to a lesser extent of Cl-. In most species, Na+ appears to accumulate to toxic levels before Cl− does (Negrão et al., 2017) and Cl– is considered less toxic than Na+ (Munns and Tester, 2008).
Thus, we focus here on Na+, because reducing Na+ in the shoot, while maintaining K+ homeostasis, is a key component of salinity tolerance in many crops (Munns, 2011). Plants of both cultivars accumulated high amounts of Na+ in the leaves when subjected to NaCl in their growing medium. The same tendency was reported in other okra genotypes (Habib et al., 2012). The results revealed that the resistant cultivar Yodana accumulated less Na+ in the leaves and roots than the sensitive cultivar Keleya. However, it was observed that the harmful effects of Na+ on growth are more accentuated on the aerial part of plants of the sensitive cultivar Keleya, compared to those of the resistant cultivar Yodana. Thus, the detrimental effects of Na+ on growth are more accentuated in aerial part of the sensitive Keleya compared to cv. Yodana plants. This is a general trend in glycophytes in which salinity resistant varieties accumulate less Na+ and/or Cl- in the leaves than susceptible varieties (Lutts et al., 1996; Almansouri et al., 1999; Akhtar et al., 2003; Wahid, 2004; Niu et al., 2010; Wouyou et al., 2019). The relative resistance of the cultivar Yodana to NaCl is therefore explained by its ability to exclude Na+ ions from the leaves leading to an avoidance or exclusion mechanism. In okra, Habib et al. (2012) have reported that foliar application of both pure glycine betaine and sugarbeet extract significantly reduced the adverse effects of salt stress in terms of plant growth, yield and leaf Na+ content indicating that the salt tolerance acquired following the foliar application of both pure glycine betaine and sugarbeet extract was due, at least partially, to Na+ exclusion from leaves. This result confirmed the importance of Na+ exclusion from leaves as part of okra plants salt tolerance strategy.
Salt stress caused a decrease in potassium ion in the leaves and roots of the two cultivars tested. This observation is also made by Maggio et al. (2007) who proved that the presence of NaCl in the plant environment generally induces an increase in Na+ and a decrease in K+ in the various organs. Similar results were reported in other okra genotype (Shahid et al., 2011). Maintaining a good K supply is one of the major responses of salt stress resistant genotypes in glycophyte species, and potassium ions are known to be a major component of osmotic adjustment during stress (Wu et al., 1996). Thus, in rice, Lutts et al. (1996) reported that a salt tolerant variety maintained high amounts of K+ in the leaves compared to salt sensitive genotypes when both types were under salt stress. The same trend has been reported in durum wheat (Almansouri et al., 1999). In the present study, the reduction in potassium content observed is clearly less pronounced in the resistant cultivar Yodana compared to the sensitive cultivar Keleya in both leaves and roots. Thus, the relative resistance of cultivar Yodana to salinity appears to be primarily associated with maintaining a good K+ supply in the presence of NaCl. Comparing the effect of foliar application of both pure glycine betaine and sugarbeet extract on okra response to salt stress, Habib et al. (2012) have revealed that this application significantly reduced the adverse effects of salt stress in terms of plant growth, yield and leaf K+ content indicating that leaf K+ accumulation is part of the strategy which mediated the salt tolerance acquired following the foliar application of both pure glycine betaine and sugarbeet extract. This result confirmed the importance of leaf K+ accumulation as part of okra plants salt tolerance strategy.
The salt-resistant cultivar Yodana accumulated less Na+ in both leaves and roots and maintained higher K+ content than the salt-sensitive cultivar Keleya. Consequently, the salt-resistant Yodana maintained a significantly higher K+/Na+ selectivity ratio in both leaves and roots than the sensitive Keleya.
Implication of organic solutes accumulation in the salinity resistance of okra cultivars
Biosynthesis of osmoprotectants and compatible solutes are among the physiological principle and biochemical mechanisms developed by plants in order to survive in soils with high salt concentration (Gupta and Huang, 2014). Proline and soluble sugars are the main parts of these osmoprotectants and compatible solutes. Salinity caused an increase in proline content in both cultivars either in leaves or in roots. Accumulation of proline is frequently reported in plants subjected to salt stress (Mishra and Saxena, 2009; Bouassaba and Chougui, 2018). It has often been considered as a compatible osmoregulator which may be involved in the mechanisms of resistance to salt stress (Ehsanpour and Fatahian, 2003; Bouassaba and Chougui, 2018). Other functions have been suggested regarding the accumulation of proline in stressed tissues ; it could be: (1) a protective agent for enzymes and membranes (Van Rensburg et al., 1993; Solomon et al., 1994), (2) a free radical scavenger (Smirnoff and Cumbes, 1989), (3) a carbon and nitrogen storage compound (Jäger and Meyer, 1977) or (4) it could be involved in the regulation of cytosolic pH (Venekamp, ??1989). However, results of the present study on stressed okra plants showed that the accumulation of proline accumulation is much more marked in resistant cultivar Yodana compared to the sensitive cultivar Keleya in both leaves and roots. We can therefore suggest that the overproduction of proline is okra plants response to salt stress and that the salinity resistance of the cultivar Yodana is associated with high proline accumulation. These results indicated that proline plays an important role in salinity tolerance as previously reported in several species (Watanabe et al., 2000; Mishra and Gupta, 2005). However, other authors have reported an opposite tendency in several species including sugar cane (Wahid, 2004; Gandonou et al., 2005, 2011), rice (Lutts and Guerrier, 1995; Lutts et al., 1996), tomato (Pérez-Alfocea et al., 1994) and amaranth (Wouyou et al., 2019). In other okra genotypes, Habib et al. (2012) have reported that foliar application of both pure glycine betaine and sugarbeet extract significantly reduced the adverse effects of salt stress in terms of plant growth and yield but reduced leaf proline in comparison to salt stress indicating that the salt tolerance acquired following the foliar application of both pure glycine betaine and sugarbeet extract was accompanied by a low leaf proline accumulation. This result suggested that proline hyper accumulation in leaves is not part of the strategy developed by okra treated plants to tolerate salt stress. Thus, the implication of proline in salinity tolerance in okra depends on the genotype and the type of salt-tolerance (genetic or artificial) taking into account.
In general, salinity caused an increase in soluble sugars content in both cultivars either in leaves or in roots. The results are consistent with the general trend. Indeed, the effects of salt stress generally result in an increase in the content of soluble sugars in both the leaves and the roots in several plant species (Bouassaba and Chougui, 2018; Wouyou et al., 2019) including okra (Abbas et al., 2014). Generally, the more tolerant cultivars accumulate more soluble sugars than the sensitive ones. Thus, Gandonou et al. (2011) reported that in sugarcane, the salinity resistant cultivar CP66-346 accumulates more soluble sugars in the leaves than the sensitive CP65-357 in the presence of salt stress. The same trend has been reported in calli, selected or not in this same species (Gandonou et al., 2005, 2006). On the other hand, in Carthamus tinctorius, Ashraf and Fatima (1995) reported that two salt-resistant accessions showed different responses: one accumulates more soluble sugars than sensitive accessions, while the other resistant accession accumulates similar amounts of sugars as sensitive accessions, although it is more tolerant. Soluble sugars are known for their role in osmoregulation in plants exposed to osmotic stress. According to Cram (1976), among the osmotically active organic compounds, sugars contribute more than 50% of the total osmotic potential in glycophytes subjected to salt stress. The fact that the salt sensitive cultivar Keleya accumulated more soluble sugars in both leaves and roots than the salt resistant Yodana, seems to indicate that soluble sugars did not play an important role in cultivar Yodana salt resistance.
Salt stress caused an increase in sodium ions, free proline and soluble sugars content and a decrease in potassium ion content in both leaves and roots in both okra cultivars with a significant difference in the reaction of okra cultivars. The overall reaction of the cultivars indicates that the relative salt resistance of cultivar Yodana is reliable to a good sodium ion exclusion and a good potassium ion accumulation mainly in leaves associated with maintaining of a good K+/Na+ ratio. Free proline appears also as a good salt resistance indicator in this cultivar.
The authors have not declared any conflict of interests.
This study was partially funded by Projet d’Appui au Développement du Maraîchage (PADMAR). The authors thank Mr. Patrice Amoussou for proof-reading the manuscript.
REFERENCES
Abbas T, Pervez MA, Ayyub CM, Shaheen MR, Tahseen S, Muhammad-Adnan-Shahid MA, Bilal RM, Manan A (2014). Evaluation of Different Okra Genotypes for Salt Tolerance. International Journal of Plant, Animal and Environmental Sciences 4(3):23-30.
|
|
Akhtar S, Wahid A, Rasul E (2003). Emergence, growth and nutrient composition of sugarcane sprouts under NaCl salinity. Biologia Plantarum 46(1):113-116.
Crossref
|
|
|
Akinci IE, Akinci S, Yilmaz K, Dikici H (2004). Response of eggplant varieties (Solanum melongena) to salinity in germination and seedling stages. New Zealand Journal of Crop and Horticultural Science 32(2):193-200.
Crossref
|
|
|
Akram M, Hussain M, Akhtar S, Rasul E (2002). Impact of NaCl salinity on yield components of some wheat accession/variety. International Journal of Agriculture and Biology 4:156-158.
|
|
|
Almansouri M, Kinet JM, Lutts S (1999). Compared effects of sudden and progressive impositions of salt stress in three Durum wheat (Triticum durum Desf.) cultivars. Journal of Plant Physiology 154:743-752.
Crossref
|
|
|
Arbona V, Macro AJ, Iglessias DJ, Lopez-Clements MF, Talon M, Gomez Cadnas A (2005). Carbohydrate depletion in roots and leaves of salt-affected potted C. clementina L. Plant Growth Regulation 46(2):153-160.
Crossref
|
|
|
Ashraf M, Fatima H (1995). Responses of some salt tolerant and salt sensitive lines of safflower (Carthamus tinctorius L.). Acta Physiologiae Plantarum 17(1):61-71.
|
|
|
Ashraf M, Foolad MR (2007). Roles of Glycine Betaine and Proline in Improving Plant Abiotic Stress Resistance. Journal of Environmental and Experimental Botany 59(2):206-216.
Crossref
|
|
|
Bates LS, Waldren RP, Teare ID (1973). Rapid determination of free proline for water-stress studies. Plant and Soil 39(1):205-207.
Crossref
|
|
|
Bouassaba K, Chougui S (2018). Effet du Stress salin sur le comportement biochimique et anatomique chez deux variétés de Piment (Capsicum annuum L.). European Scientific Journal 14(15):159-174.
Crossref
|
|
|
Bourgeais-Chaillou P, Guerrier G (1992). Salt-Responses in Lycopersicon esculentum calli and whole plants. Journal of Plant Physiology 140:494-501.
Crossref
|
|
|
Chauhan DVS (1972). Vegetable production in India (3rd Ed.). Ram Prasad and sons, Agra, India.
|
|
|
Chukwu LO, Okpe HA (2006). Differential response of Tilapia guineensis fingerlings to inorganic fertilizer under various salinity regimes. Journal of Environmental Biology 27(4):687-690.
|
|
|
Cram WJ (1976). Negative feedback regulation of transport in cells. The maintenance of turgor, volume and nutrient supply. In: U. Luttge, M.G. Pitman (Eds.). Encyclopedia of Plant Physiology. New Series. Transport in Plant Springer-Verlag, Berlin 2:284-316.
Crossref
|
|
|
Ehsanpour A, Fatahian N (2003). Effects of salt and proline on Medicago sativa callus. Plant Cell, Tissue and Organ Culture 73(1):53-56.
Crossref
|
|
|
Gama PBS, Inanana S, Tanaka K, Nakazawa R (2007). Physiological response of common bean (Phaseolus vulgaris L.) seedlings to salinity stress. African Journal of Biotechnology 6(2):79-88.
|
|
|
Gandonou CB, Bada F, Abrini J, Skali Senhaji N (2011). Free Proline, Soluble Sugars and Soluble Proteins Concentrations as Affected by Salt Stress in Two Sugarcane (Saccharum sp.) Cultivars Differing in Their Salt Tolerance. International Journal of Biological and Chemical Sciences 5(6):1488-1493.
Crossref
|
|
|
Gandonou CB, Errabii T, Idaomar M, Abrini J, Skali Senhaji N (2006). Selection of Callus Cultures of Sugarcane (Saccharum sp.) Tolerant to NaCI and Their Response to Salt Stress. Plant Cell, Tissue and Organ Culture 87(1):9-16.
Crossref
|
|
|
Gandonou CB, Gnancadja SL, Abrini J, Skali Senhaji N (2012). Salinity tolerance of some sugarcane (Saccharum sp.) cultivars in hydroponic medium. International Sugar Journal 114(1359):190-196.
|
|
|
Gandonou CB, Idaomar M, Abrini J, Skali Senhaji N (2005). Effects of NaCI on growth, ions and proline accumulation in sugarcane (Saccharum sp.) callus culture. Belgian Journal of Botany 138(2):173-180.
|
|
|
Gandonou CB, Skali SN (2015). Sugarcane (Saccharum sp.) salt tolerance at various developmental levels. In: Chakraborty U and Chakraborty B, Editors, Abiotic Stresses in Crop Plants, CABI Publishing, Wallingford 1:102-111.
Crossref
|
|
|
Gouveitcha MBG, Mensah ACG, Montcho Hambada KD, Assogba Komlan F, Gandonou CB (2021). Réponse au stress salin de quelques cultivars de gombo (Abelmoschus esculentus (L.) Moench) produits au Bénin au stade jeune plant. Journal of Applied. Biosciences (In Press).
|
|
|
Gupta B, Huang B (2014). Mechanism of Salinity Tolerance in Plants: Physiological, Biochemical, and Molecular Characterization. International Journal of Genomics 18 p.
Crossref
|
|
|
Habib N, Ashraf M, Ali Q, Perveen R (2012). Response of salt stressed okra (Abelmoschus esculentus Moench) plants to foliar-applied glycine betaine and glycine betaine containing sugarbeet extract. South African Journal of Botany 83:151-158.
Crossref
|
|
|
Haq IU, Khan AA, Khan IA, Azma MA (2012). Comprehensive screening and selection of okra (Abelmoschus esculentus) germplasm for salinity tolerance at the seedling stage and during plant ontogeny. Journal of Zhejiang University Science B 13(7):533-544.
Crossref
|
|
|
Jäger HJ, Meyer HR (1977). Effect of water stress on growth and proline metabolism of Phaseolus vulgaris L. Oecologia 30(1):83-96.
Crossref
|
|
|
Jideani VA, Adetula HO (1993). The potential of okra seed flour for weaning foods in West Africa. Ecology of Food and Nutrition 29(4):275-283.
Crossref
|
|
|
JMP Pro SAS Institute (2015). JMP® 8. User Guide, Second Edition. Cary, NC: SAS Institute Inc. Cary, NC, USA.
|
|
|
Karikalan L, Rajan SN, Gopi R, Sujatha BM, Pannersevalam R (1999). Induction of salt tolerance by triadimefon in pigeon pea (Cajanus cajan L.) Mill sp. Indian Journal of Experimental Biology 37:825-829.
|
|
|
Khan A, Shaheen F, Ahmad K, Khan ZI, Shah A, Nawaz H (2015). Amelioration of Adverse Effects of Salt Stress in Okra (Hibiscus esculentus L.) By Foliar Application of Proline. American-Eurasian Journal of Agricultural and Environmental Science 15(11):2170-2179.
|
|
|
Khan FA, Ud-Din J, Abdul G, Waseem K (2002). Evaluation of Different Cultivars of Okra (Abelmoschus esculentus L.) under the Agro-climatic Conditions of Dera Ismail Khan. Asian Journal of Plant Sciences 1(6):663-664.
Crossref
|
|
|
Lakra N, Mishra SN, Singh DB, Pushpa CT (2006). Exogenous putrescine effect on cation concentration in leaf of Brassica juncea seedlings subjected to Cd and Pb along with salinity stress. Journal of Environmental Biology 27(2):263-269.
|
|
|
Levy Y, Syvertsen JP (2004). Irrigation water quality and salinity effects in citrus trees. In: Janick J, Editor, Horticultural Reviews, John Wiley and Sons, Inc. 30:37-82.
Crossref
|
|
|
Levitt J (1980). Responses of plants to environment stresses : water, radiation, salt and other stresses. Academic Press, New York 2:365p.
|
|
|
Loko B, Montcho Hambada KD, Mensah ACG, Gouveitcha MBG, Wouyou A, Kpinkoun KJ, Assogba Komlan F, Gandonou CB (2020). Response of three tossa jute (Corchorus olitorius L.) cultivars produced in Benin to salinity stress at germination and young plant stages. International Journal of Current Research in Biosciences and Plant Biology 7(10):8-21.
Crossref
|
|
|
Lutts S, Guerrier G (1995). Peroxydase activities of two rice cultivars differing in salinity tolerance as affected by proline and NaCl. Biologia Plantarum 37(4):577-586.
Crossref
|
|
|
Lutts S, Kinet JM, Bouharmont J (1995). Changes in plant response to NaCl during development of rice (Oryza sativa L.) varieties differing in salinity resistance. Journal of Experimental Botany 46(12):1843-1852.
Crossref
|
|
|
Lutts S, Kinet JM, Bouharmont J (1996). Effects of salt stress on growth, mineral nutrition and proline accumulation in relation to osmotic adjustment in rice (Oryza sativa L.) cultivars differing in salinity resistance. Plant Growth Regulation 19:207-218.
Crossref
|
|
|
Maggio A, Raimondi G, Martino A, De Pascale S (2007). Salt Stress Response in Tomato beyond the Salinity Tolerance Threshold. Environmental and Experimental Botany 59(3):276-282.
Crossref
|
|
|
Manaa A, Gharbi E, Mimouni H, Wasti S, Aschi Smiti S, Lutts S, Ben Ahmed H (2014). Simultaneous Application of Salicylic Acid and Calcium Improves Salt Tolerance in Two Contrasting Tomato (Solanum lycopersicum) Cultivars. South African Journal of Botany 95:32-39.
Crossref
|
|
|
Mishra N, Gupta AK (2005). Effect of salt stress on proline metabolism in two high yielding genotypes of green gram. Plant Science 169(2):331-339.
Crossref
|
|
|
Mishra N, Saxena P (2009). Effect of salicylic acid on proline metabolism in lentil grown under salinity stress. Plant Science 177(3):181-189.
Crossref
|
|
|
Munns R (2011). Plant Adaptations to Salt and Water Stress: Differences and Commonalities. Advances in Botanical Research 57:1-32.
Crossref
|
|
|
Munns R, Tester M (2008). Mechanisms of salinity tolerance. Annual Review of Plant Biology 59: 651-681.
Crossref
|
|
|
Negrão S, Schmöckel SM, Tester M (2017). Evaluating physiological responses of plants to salinity stress. Annals of Botany 119(1):1-11.
Crossref
|
|
|
Niu G, Rodriguez DS, Starman T (2010). Response of bedding plants to saline water irrigation. Hortscience 45(4):628-636.
Crossref
|
|
|
Oyelade OJ, Ade-Omowaye BIO, Adeomi VF (2003). Influence of variety on protein, fat contents and some physical characteristics of okra seeds. Journal of Food Engineering 57(2):111-114.
Crossref
|
|
|
Pérez-Alfocea F, Santa-Cruz A, Guerrier G, Bolarín MC (1994). NaCl Stress-induced organic solute changes on leaves and calli of Lycopersicon esculentum, L pennellii and their interspecific hybrid. Journal of Plant Physiology 143(1):106-111.
Crossref
|
|
|
Shahid MA, Muhammad A, Pervez MA, Balal RM, Ahmad R, Ayyub CM, Abbas T, Akhtar N (2011). Salt stress effects on some morphological and physiological characteristics of okra (Abelmoschus esculentus L.). Soil and Environment 30(1):66-73.
|
|
|
Smirnoff N, Cumbes QJ (1989). Hydroxyl radical scavenging activity of compatible solutes. Phytochemistry 28(4):1057-1060.
Crossref
|
|
|
Solomon A, Beer S, Waisel Y, Jones GP, Paley LG (1994). Effects of NaCl on the carboxylating activity of rubisco from Tamarix jordanis in the presence and absence of proline-related compatible solutes. Physiologia Plantarum 90(1):198-204.
Crossref
|
|
|
Sounou EGY, Mensah ACG, Hambada Montcho KD, Gouveitcha MBG, Assogba Komlan F, Gandonou CB (2021). Response of seven African eggplant (Solanum macrocarpon L.) cultivars produced in Benin to salinity stress at seedling stage. African Journal of Agricultural Research 17(2):292-301.
Crossref
|
|
|
Van Rensburg L, Krüger GH, Krüger H (1993). Proline accumulation as drought-tolerance selection criterion: its relation-ship to membrane integrity and chloroplast ultrastructure in Nicotiana tabacum L. Journal of Plant Physiology 141(2):188-194.
Crossref
|
|
|
Venekamp JH (1989). Regulation of cytosol acidity in plants under conditions of drought. Physiologia Plantarum 76(1):112-117.
Crossref
|
|
|
Wahid A (2004). Analysis of toxic and osmotic effects of sodium chloride on leaf growth and economic yield of sugarcane. Botanical Bulletin of Academia Sinica 45:133-141.
|
|
|
Watanabe S, Kojima K, Ide Y, Sasaki S (2000). Effects of saline and osmotic stress on proline and sugar accumulation in Populus euphratica in vitro. Plant Cell, Tissue and Organ Culture 63(3):199-206.
Crossref
|
|
|
Wei W, Bilsborrow EP, Hooley P, Fincham AD, Lombi E, Forster PB (2003). Salinity induced differences in growth, ion distribution and partitioning in barley between the cultivar Maythorpe and its derived mutant Golden Promise. Plant Soil 250(2):183-191.
Crossref
|
|
|
Wouyou A, Prodjinoto H, Zanklan AS, Vanpee B, Lutts S, Gandonou CB (2019). Implication of ions and organic solutes accumulation in amaranth (Amaranthus cruentus L.) salinity resistance. American Journal of Plant Sciences 10(12):2335-2353.
Crossref
|
|
|
Wu SJ, Ding L, Zhu JK (1996). SOS 1, a Genetic Locus Essential for Salt Tolerance and Potassium Acquisition. Plant Cell 8(4):617-627.
Crossref
|
|
|
Yemm W, Willis AJ (1954). The Estimation of Carbohydrates in Plant Extracts by Anthrone. Biochemical Journal 57(3):508-514.
Crossref
|
|