ABSTRACT
The present study demonstrated the effect of jasmonic acid (JA) on pigments and vitamins involved in photosynthesis or other metabolic activities during salt stress in Brassica napus L. Exposure to different concentrations of NaCl decreased pigments such as total chlorophyll, β-carotene, and lycopene to significant low levels but interestingly salinity increased total protein of B. napus L. seedlings, which was further ameliorated via JA supplementation. Importantly, JA alone and in combination with different concentrations of NaCl enhanced growth, pigments, vitamins over control and salt-alone treatment groups. The observations suggested that JA induced salinity tolerance in B. napus L. seedlings by improving the biosynthetic level of various pigments and vitamins.
Key words: Jasmonic acid, Brassica napus, Chlorophyll, Carotenoides, β –carotene.
Abbreviation:
JA, Jasmonic acid; ROS, reactive oxygen species; TFs, trascription factors; PAP, production of anthocyanin pigmentation; EGL 3, enhancer of glabra 3; EL 3, extracellular loop; MYB 75, myeloblastosis 75; TTS, transparent testa; DAS, day after sowing; JAZs, jasmonate zim domain proteins.
Salt accumulation in agricultural soil is increasing day by day due to modern agricultural practices through artificial irrigation. Now a days, about 23% of total irrigated land have been damaged by high salts accumulation (Jouyban, 2012). The detrimental effect of high salinity on plants is the consequences of both drought and water logging conditions. Salinity stress results in ionization of Na+ and Cl- ions during water logging conditions. There after it induces wide variety of physiological and biochemical changes in plants that inhibit or reduced plant growth and development (Taffouo et al., 2010; Le Rudulier, 2005; Singha and Choudhuri, 1990). Growth inhibition in plants exposed to high salinity stress is associated with over production of reactive oxygen species (ROS) that hasten disintegration of plasma membrane and cell metabolites such as pigments and vitamins. Salinity shows high accumulation of superoxide radicals and H2O2 in Oryza sativa which consequently reduced growth (Chattopadhayay et al., 2002). Plant can tolerate high level of stress by controlling free radicals and ROS through their antioxidant defense system which consists of various enzymatic and non-enzymatic components (Sairam and Srivastava, 2002; Bor et al., 2003).
Many strategies have been considered or employed to maximize plant growth and productivity under various stresses. A fundamental approach is to develop salt-tolerant varieties through use of plant growth regulators.
Jasmonic acid is an important plant growth regulator involved in plant responses to biotic and abiotic stresses as well as in development. Recently, Wasternack and Hause (2013) reviewed the latest breakthrough in JA regulated processes such as seed germination, seedling development, root growth, flower development, seed development, tuber formation and senescence. JA is also involved in induction of anthocyanin formation by regulation of transcription factors (TFs) such as PAP, EGL 3, EL 3, MYB 75 and TTS which are involved in anthocyanin biosynthesis and trichome development (Qi et al., 2011). JA signaling regulated cellular network involved in biosynthesis of secondary metabolites (Chena et al., 2006).
This experiment was planned with an aim to relate a change in the level of pigments and vitamins, in salt stressed seedlings of B. napus with the induced resistance and to neutralize the effects of stress by the application of jasmonic acid. The hypothesis that the application of jasmonic acid will mitigate the toxic effect of salt on the growth of B. napus seedlings was tested.
Seeds of Brassica napusL.cv. ‘GSC 6’ (certified) were procured from Department of Plant Breeding, Punjab Agriculture University, Ludhiana, India. Seeds were surface sterilized with 0.5% sodium hypochlorite for 15 min, followed by repeated rinses in sterile distilled water. The sterilized seeds were presoaked in different concentrations of jasmonic acid (0, 10-6, 10-9, and 10-12 M) for 8 h and then germinated on Whatman No. 1 filter paper containing (0, 80, 100 and 120 mM NaCl) alone or in combination for 12 days. The experiment was conducted under controlled conditions (25±2°C, 16 h photoperiod) and repeated thrice with three replications for each treatment.
Growth analysis
Twelve days old seedlings (DAS) were harvested and their root and shoot length were recorded. Percentage germination was recorded at 3rd DAS. Twenty seedlings per Petri dish were used for the determination of morphological parameters.
Biochemical analysis
Total soluble protein content was estimated by homogenizing 1 g fresh seedling tissue in 3 ml of 100 mM phosphate buffer (pH 7.0) by following the method described by Lowry et al. (1951) using bovine serum albumin as a standard. Chlorophyll and carotenoids were extracted according to Arnon (1949). Brassica shoot tissue (0.5 g) was ground using mortar and pestle in 5 ml of 80% acetone and then the developed colour was measured at 470, 645, and 663 nm. The amount of pigments was calculated as described by Lichtenthaler (1987). For β-carotene and lycopene estimation, seedlings were crushed in acetone-hexane (4:6) mixture and absorbance was measured at 453, 645, 505, and 663 nm by following Nagata and Yamashita (1992). Anthocyanin content was extracted in 300 μl methanol (1% HCl) and the absorbance was read spectrophotometrically at 530 and 657 nm according to Michael et al. (1998).
Vitamin A content was estimated by using the method of Bayfield and Cole (1980) with slight modification, 3 ml reaction mixture contained 1 ml of chloroform and 2 ml of trichloroacetic acid solution. The absorbance was recorded at 620 nm. Vitamin C content was determined spectrophotometrically by following the method of Chinoy et al. (1976). Standard curve was prepared by using grades of ascorbic acid (10 to 100 μg). Vitamin E content was estimated following the method described by Rosenberg (1992).
Statistical analysis
All analysis was done on a completely randomized design. All data obtained was subjected to a two-way analysis of variance (ANOVA) and the mean differences were compared by Tukey’s test using prism software 5.5. Each data was the mean of three replicates (n=3) except for shoot and root length of B. napus L. seedlings (n=5); and comparisons of p-values <0.05 were considered significant and different from control.
Morphological parameters
Jasmonic acid treated seeds resulted in decreased percentage germination, root and shoot length (Table 1) of Brassica napus as compared to control. Maximum decrease in germination rate (40%) was observed in seedlings treated with 120 mM NaCl concentrations. Interestingly, seeds grown in presence of 100 mM salt after treatment with different concentrations of JA showed increase in germination rate (63%) as compared to control distilled water seeds.
Seedling growth in terms of root and shoot lengths also showed synergistic mechanism of positive effect on growth particularly on root lengths. Shoot lengths were also affected positively in the presence of JA alone. In the presence of different concentrations of NaCl solutions, an increase in seedling growth was observed (Table 1). Maximum shoot lengths (20%) and root lengths (49%) was found in 10-9 M JA+100 mM NaCl. Overall, JA showed positive effect on seedling growth in the presence or absence of NaCl.
Biochemical parameters
The significant effect of JA was observed on the biochemical parameters in Brassica napus seedlings. Total soluble protein content was increased with decreased concentrations of NaCl stress (Figure 1A). Seedlings treated with 120 mM NaCl showed (75%) decrease in total soluble protein content as compared to control. Seedlings treated with JA alone showed significant increase in total protein content (Figure 1A) in comparison to untreated seedlings. The soluble protein content was significantly higher in the seedlings treated with NaCl supplemented with JA than NaCl alone.
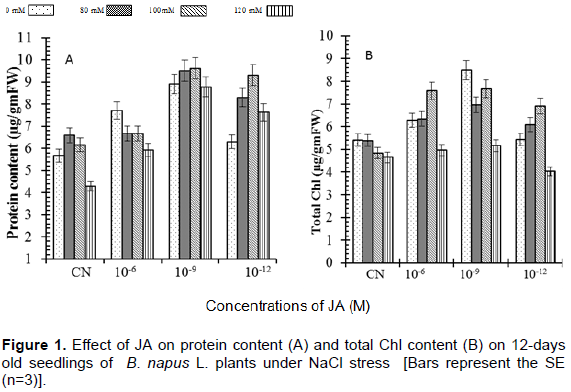
Total chlorophyll content was decreased with increased concentrations of NaCl as compared to control (Figure 1B) where it was (15%) lower as compared to control. Applications of different concentrations of JA under salt stress enhance chlorophyll content to significant high level. Maximum total chlorophyll content was observed in case of seedlings treated with 100 mM NaCl supplemented with 10-9 M JA which was (43%) high as compared to control.
Similar trend was observed when effect of JA was studied on the contents of total carotenoids and anthocyanin under NaCl stress (Figure 2). Carotenoids content (Figure 2A) was (40%) lower in 100 mM NaCl and anthocyanin (Figure 2B) was 53% lower in 120 mM NaCl as compared to control. Carotenoid and anthocyanin content were (160%) higher in seedlings treated with 10-9 M JA supplemented with 100 mM of NaCl.
β-Carotene and lycpene content (Figure 2) decreased significantly under 120 mM of NaCl (44%) and (78%) as compared to the control. JA alone was not able to alleviate the decreased contents of β-carotene and lycopene but in the presence of NaCl, an increase level of β-carotene and lycopene contents was observed (Figure 2C and D). β-carotene and lycopene contents were reported to be maximum in case of seedlings treated with 10-9 M JA supplemented with 100 mM NaCl which was 39 and 148%, respectively.
Conversely, vitamin A, C and E contents declined under NaCl stress and were found to increase significantly by application of different concentrations of JA under NaCl stress (Figure 3). Vitamin A content (Figure 3A) decreased significantly under 120 mM NaCl stress (32%) and the decrease was maximum (40%) in seedlings treated with 10-9 M JA supplemented with 100 mM of NaCl. Vitamin C content (Figure 3B) decreased under NaCl stress up to 120 mM (80%) and was not significantly improved when supplemented with JA. Vitamin E content (Figure 3C) was decreased (53%) in 120 mM of NaCl, when compared with control. Applications of JA improved vitamin E content significantly in the presence of 100 mM NaCl stress and maximum content was found (139%) in 10-9 M JA. It was observed that Jasmonic acid helped in mitigating the NaCl stress in Brassica napus by regulating the levels of photosynthetic pigments and non-enzymatic antioxidants but in dose dependent manner.
Salinity is the substantial cause of reduction in growth yield and productivity in plants (Munns, 2005). Stress caused as a result of high salt concentration alters the internal metabolic machinery of the plant which modulates the physiology and biochemistry of plants thereby adversely affecting growth and productivity of plants (Raza et al., 2007). JA is an oxylipin which is well established as a plant growth regulator and protects plants from biotic and abiotic stresses. The present study is an attempt to demonstrate the ameliorative role of JA in mitigating detrimental effect of salinity on physiology and biochemistry of plants. Detrimental effect of all concentrations of salt was observed on the studied morphological parameters (Table 1). Priming treatment of different concentrations of JA in seedlings exposed to different saline treatments mitigated the detrimental effect of salt stress via improvement in percentage germination compared to control (untreated group) though significant only to that of saline treated seeds. JA treatment also enhanced root: shoot content. JA is a signaling molecule which regulates signal pathway responsible for various gene expression involved in plant growth and development. Exogenous application of JA to seedlings exposed to different saline conditions might overcome the suppression of COI1/JAZ/MYC2 gene expression which is involved in plant growth through mitotic cell division control (Rymen and Sugimoto, 2012; Zhang and Turner, 2008).
In the present study, 80 mM NaCl increased protein content significantly to that of control, followed by 100 mM NaCl concentration (Figure 1A), but 120 mM NaCl treatment decreased total protein content of B. napus L. seedlings. High amount of total soluble proteins enable plants to respond favourably to high salinity and survive; this further improves in the presence of exogenous JA. It is proposed that JA induced changes that are mediated through jasmonates-induced stress proteins (Rakwl and Komatsu, 2001). Similarly in our results, it might be the case that JA application induced stress proteins, which were involved in various growth-related processes.
The obtained results for chlorophyll accumulation in JA treated seedlings exposed to various salinity conditions presented antagonistic data from that obtained in literature (Figure 1B). From a previous study, it was reported that JA induced two key enzymes which are involved in chlorophyll degradation in which gene encoding the chlorophyllase 1 is strongly induced by JA (Tsuchiya et al., 1999). In the present study, JA application induced high accumulation of total chlorophyll in simple distilled water seedlings. These results suggested that JA has great potential in protecting B. napus L. plants against salinity stress. JA induced higher accumulation of carotenoids, β-carotene, anthocyanin and lycopene along with vitamins A, C and E under normal as well as high salinity condition in plants of B. napus (Figures 2A to D and 3A to C). Accumulation of various pigments and vitamins decreased with increase in salinity stress in B. napus plants. These results are in accordance with Verma and Mishra (2005) who reported that high salinity decreased pigment accumulation in B. juncea seedlings. The degradation in pigments and vitamins accumulation observed may be due to production of more free radicals under salinity stress which caused secondary metabolites deterioration. JA regulated the level of all pigments and vitamins which suggested that JA might be activating and/or be involved synthesis of transcription factors (TFs) involved in formation of these pigments and vitamins. The regulatory role of JA in TFs-mediated metabolism of secondary metabolites has been reviewed recently by Memelink (2009) and Geyter et al. (2012). Qi et al. (2011) provided biochemical and genetic evidences of involvement of transcription factors (TFs) in anthocyanin biosynthesis through targeting of JAZs, an inhibitor of JA signaling. The present findings reveal that JA treated B. napus plants had high ability to deal with oxidative stress that might govern their sensitivity to salt stress status.
The influence of JA on pigments, vitamins and seedling growth was more prominent under NaCl stress, suggesting that JA treated seedlings were less affected by NaCl than the untreated seedlings. Also, JA induced accumulation levels of proteins, which increased the tolerance of Brassica napus seedlings to NaCl stress. However, further studies at molecular level are needed to elucidate the complete mechanism involved in JA inducing salt tolerance in plants.
The authors have not declared any conflict of interests.
REFERENCES
Arnon DI (1949). Copper enzymes in isolated chloroplasts. Polyphenoloxidase in Beta vulgaris.Plant. Physiol. 24:1-15.
Crossref
|
|
Bayfield RF, Cole ER (1980). Colorimetric estimation of vitamin A with trichloroacetic acid. Methods Enzymol. 67:189-195.
Crossref
|
|
|
Bor M, Ozdemir F, Turkan I (2003). The effect of salt stress on lipid peroxidation and antioxidants in leaves of sugar beet Beta vulgaris L. and wild beet Beta maritime L. Plant. Sci.164:77-84.
Crossref
|
|
|
Chattopadhayay MK, Tiwari BS, Chattopadhyay G, Bose A, Sengupta DN, Ghosh B, (2002). Protective role of exogenous polyamines on salinity-stressed rice (Oryza sativa) Plants. Physiol. Plant.116:192-199.
Crossref
|
|
|
Chena H, Daniel A, Jonesb C, Gregg A, Howea B (2006). Constitutive activation of the jasmonate signaling pathway enhances the production of secondary metabolites in tomato. FEBS Letters. 580: 2540–2546.
Crossref
|
|
|
Chinoy JJ, Singh YD, Gurumurthi K (1976). Colorimetric determination of ascorbic acid turnover in plants. J. Plant. Physiol. 22:122-130.
|
|
|
De Geyter N, Gholami A, Goormachtig S, Goossens A (2012). Transcriptional machineries in jasmonate elicited plant secondary metabolism. Trends. Plant. Sci. 17:349 -359.
Crossref
|
|
|
Jouyban Z (2012). The effects of salt stress on plant growth. Tech. J. Eng. Appl. Sci. 1:107-100.
|
|
|
Le Rudulier D (2005). Osmoregulation in rhizobia: the key role of compatible solutes. Grain Legume 42:18-19.
|
|
|
Lichtenthaler HK (1987). Chlorophylls and Carotenoids: Pigments of Photosynthetic Biomembranes (L Packer and R Douce, eds), Methods in Enzymol. 148:350-382, Academic Press, New York.
|
|
|
Lowry H, Rosenbrough NJ, Farr AL, Randall RJ (1951). Protein measurement with the folin phenol reagent. J. Biol. Chem.193: 265.
|
|
|
Memelink J (2009). Regulation of gene expression by jasmonate hormones.Phytochem.70: 1560-1570.
Crossref
|
|
|
Michael M, Neff J, Chory (1998). Genetic Interactions between Phytochrome A, Phytochrome B, and Cryptochrome 1 during Arabidopsis Development. Plant. Physiol.118:27-35.
Crossref
|
|
|
Munns R (2005). Salinity stress and its impact. In: Blum, A. (ed.) Plant Stress.
View.
|
|
|
Nagata M, Yamashita I (1992). Nippon Shokuhin Kogyo Gakkaishi. 39:925.
Crossref
|
|
|
Qi T, Song S, Ren Q (2011). The jasmonate-ZIM-domain proteins interact with the WD-Repeat/bHLH/MYB complexes to regulate jasmonate mediated anthocyanin accumulation and trichome initiation in Arabidopsis thaliana. Plant Cell. 23:1795-1814.
Crossref
|
|
|
Qi T, Song S, Ren Q (2011). The jasmonate-ZIM-domain proteins interact with the WD-repeat/bHLH/MYB complexes to regulate jasmonate-mediated anthocyanin accumulation and trichome initiation in Arabidopsis thaliana. Plant Cell 23:1795-1814.
Crossref
|
|
|
Rakwl R, Komatsu S (2001). Jasmonic acid induces necrosis drastic decrease in Ribulose 1, 5-biphosphate carboxylase / oxygenase in rice seedlings under light involves reactive oxygen species. Plant Physiol. 158:679-688.
Crossref
|
|
|
Raza SH, Athar HR, Ashraf M, Hameed A (2007). Glycine betaine induced modulation of antioxidant enzymes activities and ion accumulation in two wheat cultivars differing in salt tolerance. Environ. Exp. Bot. 60:368-376.
Crossref
|
|
|
Rosenberg HR (1992). Chemistry and physiology of vitamins: Interscience publishers Inc, New York pp. 452-453.
|
|
|
Rymen B, Sugimoto K (2012). Tuning growth to the environmental demands. Curr. Opin. Plant. Biol.15:683-690.
Crossref
|
|
|
Sairam RK, Srivastava G C (2002). Changes in antioxidant activity in sub-cellular fractions of tolerant and susceptible wheat genotypes in response to long term salt stress. Plant Sci. 162:897-904.
Crossref
|
|
|
Singha S, Choudhuri MA (1990). Effect of salinity (NaCl) stress on H2O2 metabolism in Vigna and Oryza seedlings. Biochem. Physiol. Pflanz. 186:69-74.
Crossref
|
|
|
Taffouo VD, Nouck AH, Dibong SD, Amougou A (2010). Effect of salinity stress on seedling growth, numeral nutrients, and total chlorophyll of some tomato (Lycopersicum esculentum L.) cutivars. Afr. J. Biotechnol. 9(33):5366-5372.
|
|
|
Tsuchiya T, Ohta H, Okawa K (1999). Cloning of chlorophyllase, the key enzyme in chlorophyll degradation: finding of a lipase motif and the induction by methyl jasmonate. Proc. Nat. Acad. Sci. USA. 96(26):15362-15367.
Crossref
|
|
|
Verma S, Mishra SN (2005). Putrescine alleviation of growth in Brassica napus by inducing antioxidant defense system. Plant Physiol. 162:669-677.
Crossref
|
|
|
Wasternack C, Hause B (2013). Jasmonates: biosynthesis, perception, signal transduction and action in plant stress response, growth and development: an update to the 2007 Review in Annals of Botany. 111:1021-1058.
Crossref
|
|
|
Zhang Y, Turner JG (2008). Wound-induced endogenous jasmonates stunt plant growth by inhibiting mitosis. PLoS ONE. 3(11): e3699. doi:10.1371/journal.pone.0003699
Crossref
|
|