ABSTRACT
Drought stress is a major factor decreasing cotton productivity in Malawi. To identify drought tolerant cultivars, a study was conducted in 2012 at Bunda College to evaluate the performance of 20 cotton genotypes under water stress conditions. A screen house pot experiment was carried out using a randomized complete block design and data were recorded on tap root length, lateral root number, fresh root weight, dry root weight, fresh shoot weight, dry shoot weight, shoot length, root volume, number of leaves per plant, and stem diameter. Results revealed significant differences among genotypes for response to drought stress. Six genotypes (06K485, 06K486, SPAN 837, FQMA (05) 5 bcp, Chureza, and RASAM 17) showed drought tolerance. The inclusion of these genotypes as parents in the drought tolerance breeding programme can have a significant impact to minimize the adverse effects of drought on cotton in Malawi.
Key words: Cotton, genotypes, water stress, growth, productivity traits.
Cotton (Gossypium hirsutum L.) is the most important fiber crop, providing half of the global fibre requirement (Pretorius, 2009; Poehlman and Sleper, 1995). Despite the availability of synthetic alternatives, it continues to serve as the most important source of fiber for textiles (Sunilkumar et al., 2006; Martin et al., 2006). The seed is also of economic importance (Pretorius, 2009) and used as a primary source of vegetable oil for culinary purposes, with the oilcake residue as a protein-rich feed for ruminant livestock (FAO, 1994). Cottonseed contains 21% oil and 23% protein, both of which are of relatively high quality (Rathore, 2007). Cotton seed oil is also used in products such as soap, margarine, emulsifiers, cosmetics, pharmaceuticals, rubber, and plastics (USDA, 2008). In Malawi, cotton is one of the most important cash crops (MoAFS, 2006). Rural households planting cotton rely almost solely on the crop for their cash income, which is used for buying food items for family consumption (Fortucci, 2002). Despite its importance as a cash crop for a considerable proportion of the country’s farming community, farmers generally obtain very low yields, which are about 25 to 30% of the potential production.
Drought is one factor contributing to the huge disparity in yields (MoAFS, 2005). Drought stress has the highest percentage (26%) when the usable areas on the earth are classified in view of stress factors (Farshadfar et al., 2012). Genetically, equivalent cotton plant populations, when subjected to water deficit show reduction in yield of up to 50% if compared to those that have been irrigated (Brito et al., 2011). Malawi depends on rain-fed agriculture which is vulnerable to extensive dry spells and droughts. The country has experienced changing rainfall patterns in recent years, including changes in the on-set of rains and irregular and uneven rainfall distribution (Ministry of Mines, Natural Resources, Energy and Environment, 2006). Irrigation has the potential to increase crop production; however, many farmers do not have access to adequate irrigation facilities. Cotton varieties with acceptable levels of drought tolerance are the only cost-effective drought management tactic available to small-scale farmers. Genotypic selection for adaptation to different water regimes is an important strategy in breeding programmes to develop drought tolerant varieties (Monneveux and Ribaut, 2011).
A lot of work has been done to develop drought tolerance in cotton, that is, Basal et al. (2005) reported that root characteristics play an important role in determining the response of plants to drought and that water deficit decreases shoot growth rate, plant height and yield, but root growth is less sensitive to drought than shoot growth. Root elongation during drought may help plants get deeper water, thus avoiding water deficits near the soil surface (Pace et al., 1999). Basal et al. (2005) reported that drought-stressed cotton seedlings showed some increase in root length but reduced diameter. Iqbal et al. (2011) found out that the differing measurement of root and shoot lengths of G. hirsutum seedlings indicated variability among varieties/lines to the adverse effect of water stress. Basal et al. (2005) indicated that root growth is a reliable indicator of the response to drought tolerance. Significant variability for taproot length and number of lateral roots among exotic cotton germplasm has been reported. It has been indicated that the day-neutral converted race stocks (CRS) accessions have useful genetic variability for root growth parameters which were root length (RL), lateral root number (LRN), root fresh weight (RFW), lateral root dry weight (LRDW) and total root dry weight (TRDW) (Basal and Unay, 2006).
Kohel and Lewis (1984) reported that significant genetic variability exists among the exotic strains of G. hirsutum for dry matter accumulation, heat tolerance and root growth; and that root growth and vigorous growth of root laterals are important to the adaptation of cotton to limited supplies of soil water. Ali et al. (2011) reported that the information about significant correlation among the traits is important for initiation of any breeding programme because it provides a chance for selection of desirable genotypes with desirable traits. Basal et al. (2005) found that root length, lateral root number, total dry root weight, and shoot dry weight were all positively and significantly correlated. Currently, there is no information on the performance of cotton genotypes under water stress conditions; hence, the screening of cotton varieties grown in Malawi is needed to identify drought tolerant varieties. The present study was carried out to determine the genotypic variation among 20 cotton genotypes for growth and productivity traits in response to water stress to identify drought tolerant varieties.
Because there is no information available on drought tolerance for cotton varieties grown in Malawi, 20 genotypes were randomly chosen from released varieties, promising lines and working accessions. The genotypes were SZMA (04) 4bcp, FQMA (05) 5bcp, MAP85 (05) 18bcp, Acala glandless, CHUFQ (06) 1bcp, Glandless NC-1, CHUMA (04) 17 bcp, 06K485, K502MA (05) 1bcp, IRMSZ (06) 3bcp, MACHU (06)1, BF26 (03) 4bcp, SPAN 837, MTB (84) 2, SZ9314, 06K486, Makoka 2000, RASAM 17, IRM 81, and Chureza. The genotypes varied for leaf colour, yield potential (seed cotton yield), ginning out turn, tolerance to jassid insect attack and bacterial blight disease, gossypol levels, and fibre colour (Table 1). Seeds were obtained from the Department of Agricultural Research Services (DARS) national cotton breeding programme in Malawi. The study was conducted in a translucent plastic screen house at the Student’s Research Farm of the Department of Crop and Soil Sciences, Bunda College of Agriculture (14°11´ S and 33°46´ E, 1100 m above sea level), Lilongwe, Malawi from March to June 2012.
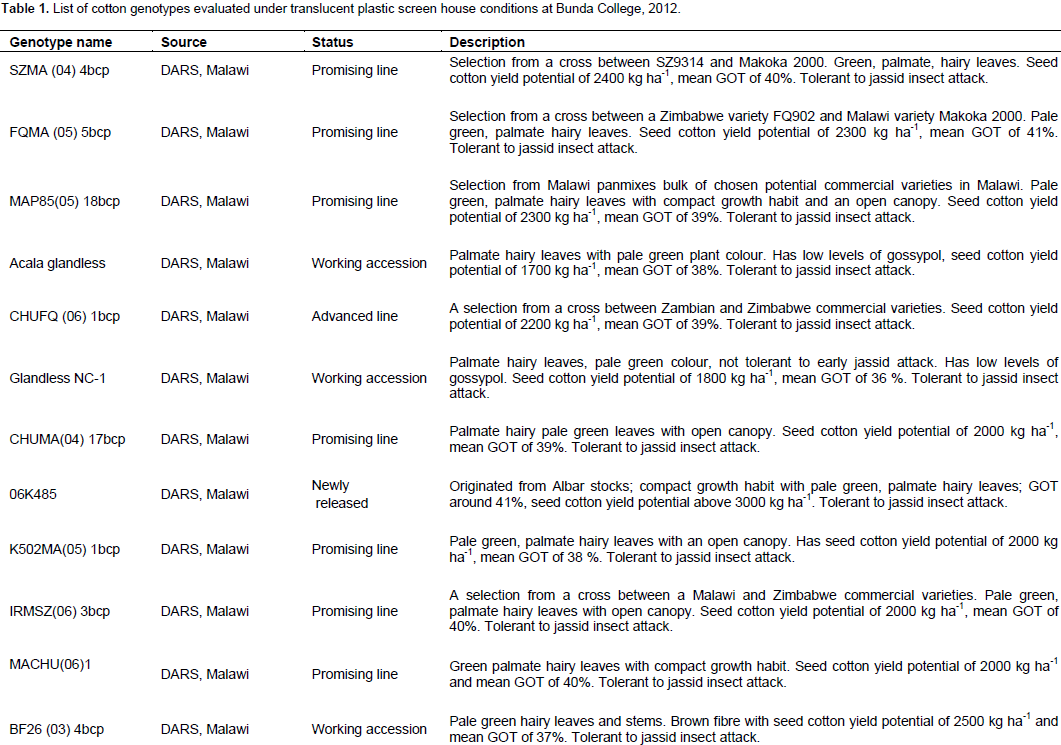
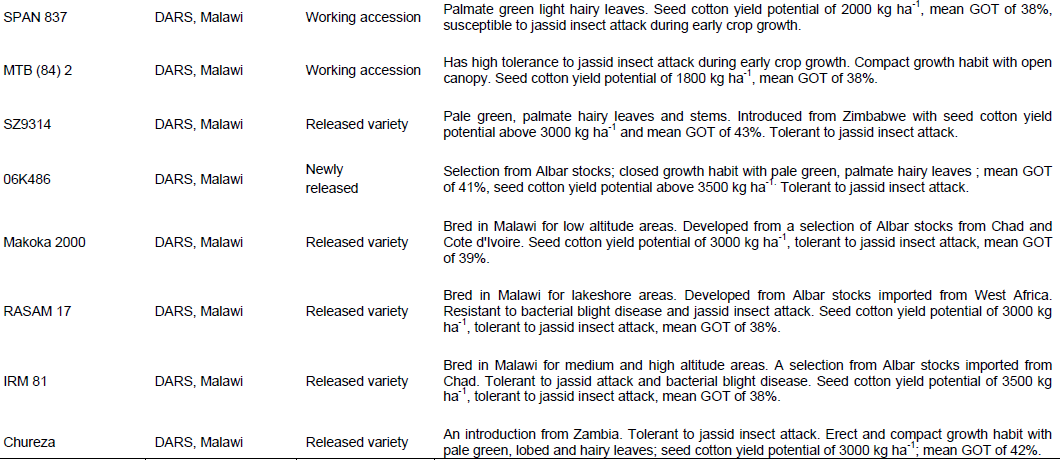
The experiment was a randomized complete block design with two watering regimes (well - watered and water - stressed), and 20 genotypes, making a total of forty treatment combinations. The treatments were replicated three times. Each experimental unit composed of two pots with three plants per pot, giving a total of 240 pots. Five-litre plastic pots, perforated at the base, were filled with 4.0 kg of soil composed of 2 parts loam soil and 1 part river sand. Pots were watered to field capacity before planting. NPK fertilizer (23:21:0 + 4 S) was thoroughly mixed in water and added to each pot prior to planting at rates equivalent to 34 kg ha-1 N, 45 kg ha-1 P2O5, and 22 kg ha-1 S (Sarrantonio, 1991). Eight fuzzy cotton seeds per pot were sown on 13 March, 2012. Seedlings were thinned to three plants per pot, three weeks after planting. Plants were allowed to grow under optimum water regime from sowing to 38 days after emergence (DAE). Thereafter, pots were divided into two sets; one set was treated as the well-watered (W1) control and the other set was the water-stressed (W2) treatment. For the non-stressed water regime, pots were maintained at field capacity throughout the growing period by irrigating four times a week with 500 ml of water per pot. In the water-stressed regime, stress was imposed by withholding water from the pots until 50 % of the plants showed signs of stress.
Drought stress was determined by visually evaluating plants for wilted or rolled leaves where the rolled leaf rim covered part of the leaf blade (Monneveux and Ribaut, 2011). These signs of drought stress appeared after four days, after which pots were irrigated four times per week with 250 ml of water per pot. Therefore, the water-stressed treatments received 50% of the quantity of water compared to the well-watered controls needed in the non-stress condition (Ali et al., 2011) in order to relieve the signs of wilting, but not enough water to reach soil field capacity (Loka and Oosterhuis, 2009). The treatments were maintained for 21 days. The effects of drought stress were determined by measuring 11 parameters including tap root length (TRL), lateral root number (LRN), root fresh weight (RFW), root dry weight (RDW), shoot fresh weight (SFW), shoot dry weight (SDW), shoot length (SL), root volume (RV), total biomass (TBM), stem diameter (SD), and number of leaves per plant. A mean of three plant measurements of each genotype was used for statistical analyses for all the parameters in each replicate under non-stress and stressed conditions. The methods of measurements are subsequently described in detail.
Taproot length (TRL) of each plant was determined by removing the soil together with the plants from the pot, uprooted the plants carefully as the soil was loose, washed them free of soil and then directly measured the tap roots in centimeters (cm), before oven drying, from the junction of the shoot and root to the terminal of the root with a measuring tape. Lateral root number (LRN) was determined by direct counting of roots before oven drying. Roots were washed free of soil, spread on a paper for determination of lateral root number, a technique similar to the one used by Basal et al. (2005). Plants were cut at the junction of the root and shoot to measure fresh weight of the roots. Fresh weight of the roots (RFW) was recorded in grams (g) before oven drying, using an electronic balance. In order to measure root volume (RV) in mm3, roots were washed free of soil and a graduated measuring cylinder with known water volume was used as the following. Root volume = (Water + roots volume) – water volume. Root dry weight (RDW) was determined by placing the roots in paper bags and oven drying for 48 h at 75°C (Ali et al., 2011), to have the roots completely dried. Root dry weight (g) was weighed with the help of an electronic balance. Shoot fresh weight (SFW) was determined after shoot was separated by cutting at the junction of root and shoot. SFW was obtained with an electronic balance in grams, a procedure as was used by Iqbal (2010). Shoot length (SL) was obtained after the shoot was separated by cutting at the junction of root and shoot (Iqbal, 2010).
A measuring tape was used to measure SL (cm) from the cotyledonary node to the apical bud.Shoot dry weight (SDW) was obtained after recording fresh weight. Shoot samples were then placed in paper bags and oven dried for 48 h at 75°C to get the shoots completely dried. Shoot dry weight (g) of each treatment was recorded with the help of an electronic balance. Weight of each plant (dry root weight + dry shoot weight) after oven drying was recorded after weighing on a digital balance to obtain total biomass (TBM). Stem diameter (SD) was measured on the shoot which was earlier separated from the root using a ruler from the middle of the lower first and second node of the plants (Iqbal, 2010). Number of leaves per plant was obtained by direct counting of leaves of each plant before uprooting (Mahmood et al., 2006).
Data analysis
The mean of three plant measurements of each genotype was used for statistical analyses for all the parameters in each replicate under non-stress and stressed conditions. Data were subjected to analysis of variance using General Statistics (GenStat 14th edition) to test for differences among genotypes, water regimes and interactions between cotton genotypes and watering regimes. Significant means were separated using the least significant difference at 5% probability level (LSD0.05). Correlation analysis for the traits was performed using GenStat 14th edition computer package to assess the relationships among them. Percent change in parameters measured under water-stress was derived from the difference in parameters between non-stress and stress conditions as follows:

Positive values indicated reduction of the parameter under water stress in relation to non-stress water regime; negative values represent an increase and zero indicated that there was no change in the parameter under water stress.
Mean squares computed through analysis of variance are presented in Table 2. The genotypes were highly significant with respect to the majority of the measured parameters. Water regimes were also highly significant for all the measured parameters. The results showed high significant differences for interactions between water regime and cotton genotypes except root dry weight. All the 11 parameters which were evaluated were affected by water stress. The variable expressions of 20 cotton genotypes for various traits under water stress indicated that there was genotypic variability for drought tolerance. The presence of variability among genotypes for different traits under water stressed conditions has been reported (Basal et al., 2005; Iqbal, 2010; Bibi et al., 2012). TRL and LRN have been shown to be increased by water stress (Pace et al., 1999; Chaturvedi et al., 2012). Increased TRL in response to water stress may permit cotton plants to survive drought by accessing water from deeper layers in the soil profile during periods of limited water supply. The majority of the genotypes in the present study showed a reduction in TRL and LRN (Table 3).
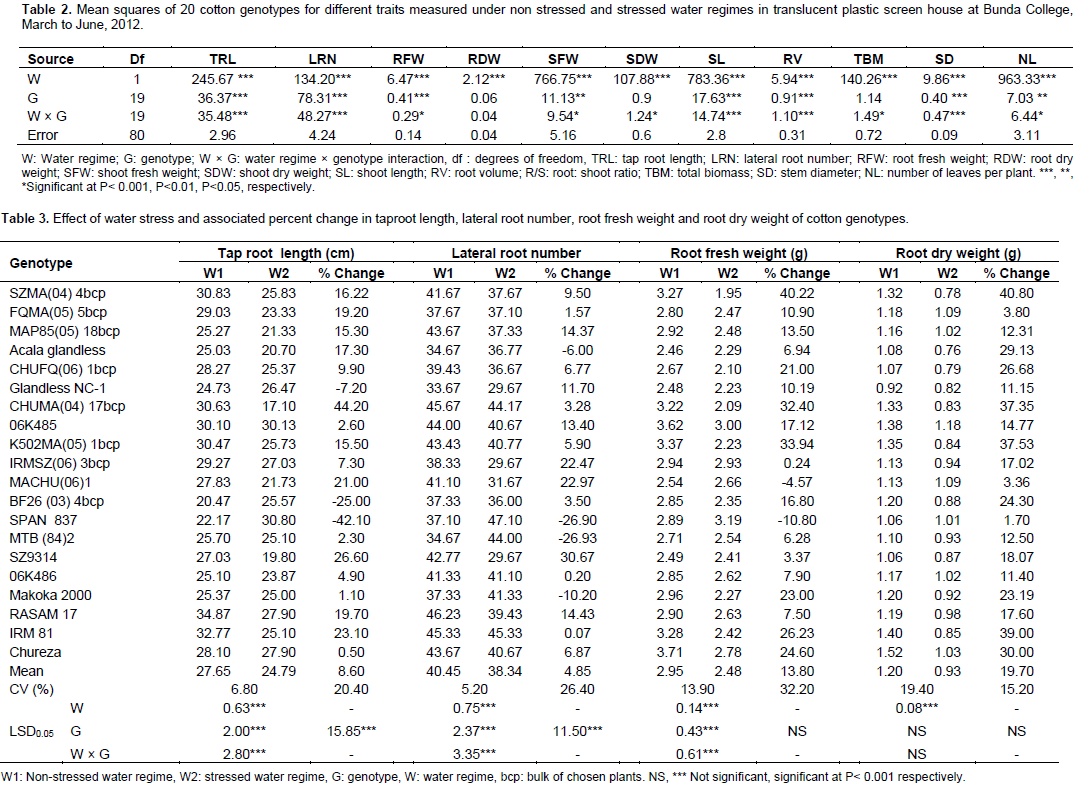
Only two genotypes (BF26 (03) 4 bcp and SPAN 837) showed a significant increase in TRL under drought stress; whereas, nine genotypes showed no significant change in TRL between the water regime treatments. For LRN, two genotypes (SPAN 837 and MTB (84) 2) showed a significant increase under stress with 11 genotypes showing no significant change in LRN. Root growth has been reported as a reliable indicator of the response to drought tolerance due to significant variability for TRL and LRN (Basal et al., 2005; Kohel and Lewis, 1984). Additionally, nearly all genotypes showed a reduction in RFW under stress with only genotype SPAN 837 showing a significant increase (Table 3). All genotypes showed a reduction in RDW under stress; although, genotypes SPAN 837 and MACHU (06) 1 were less affected by water stress due to minimum reduction in RDW (Table 3). Nearly all genotypes showed large reductions in SFW and SDW (Table 4). Only one genotype 06K486 showed no significant reductions in these two traits. SFW and SDW were much lower under water stressed conditions, suggesting that shoot growth was more sensitive to water stress than root growth. Basal et al. (2005) reported that SFW and SDW could be used as selection criteria for drought tolerance because of their ease of measurement and reliability.
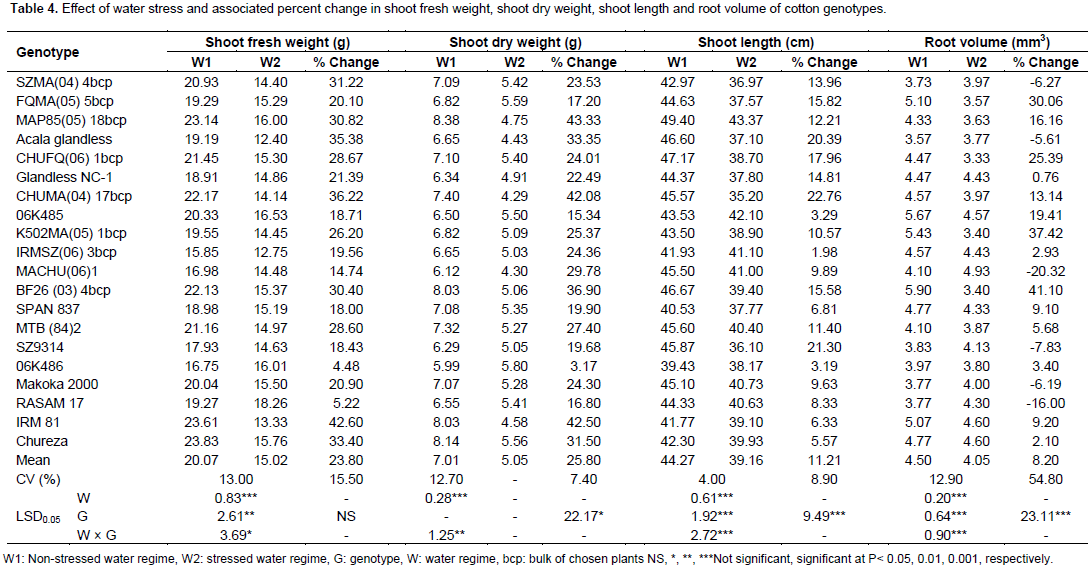
All genotypes showed a reduction in SL under drought stress (Table 4). The reduction of SL could be attributed to decrease in cellular expansion resulting from lower plant water content and turgor pressure under water stress (Abayomi and Abidoye, 2009). The majority of the genotypes also showed a reduction in RV; although, two genotypes showed a significant increase in RV under stress. Shoot length and root volume have been used as selection parameters for drought tolerance by Iqbal (2010) and Chaturvedi et al. (2012). Total biomass was significantly reduced under drought stress for all genotypes except 06K486 (Table 5). Genetic variability has been reported to exist for dry matter accumulation (Poehlman and Sleper, 1995); however, no significant variation was observed in the present study. Genotypes with higher biomass under water stress conditions are able to develop sufficient biomass early, as such, the available moisture would be utilized before it is lost through deep drainage and soil evaporation (Taiz and Zeiger, 2006). Stem diameter and number of leaves per plant were significantly decreased for all genotypes due to water stress (Table 5). Taiz and Zeiger (2006) indicated that for indeterminate plants, water stress limits leaf number.
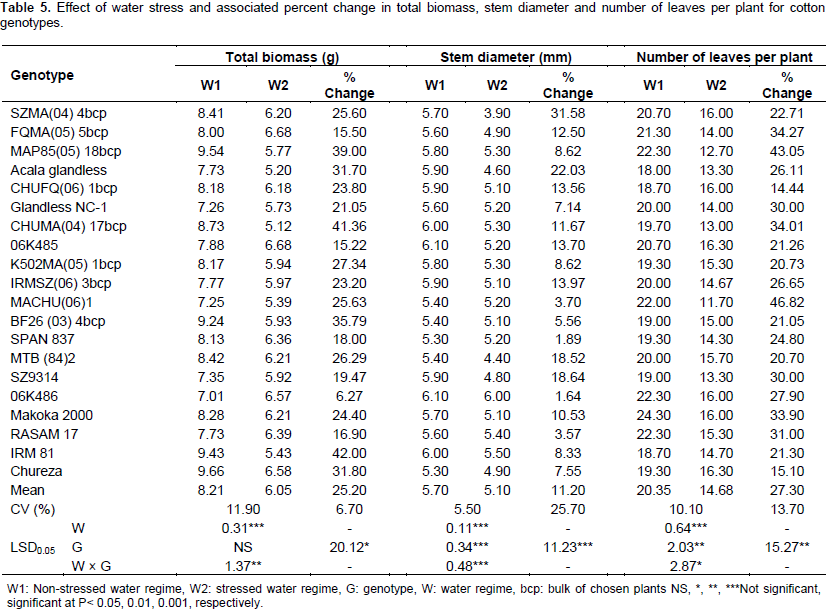
Akıncı et al. (2012) indicated that water stress caused major reductions in leaf number of cotton plants. Correlations among the traits (Table 6) revealed a lot of positive and significant associations among root traits as well as between root and shoot related traits. Taproot length, lateral root number, shoot fresh weight, shoot dry weight, root fresh weight, root dry weight, shoot length, root volume, stem diameter and number of leaves per plant were positively correlated with total biomass. The association between growth parameters and total biomass had positive correlation coefficients implying that selection for taproot length, lateral root number, shoot fresh weight, shoot dry weight, root fresh weight, root dry weight, shoot length, root volume, stem diameter and number of leaves might improve total biomass under water stressed conditions. Correlation analysis further suggested that simultaneous improvement could be possible for shoot fresh weight, shoot dry weight and shoot length due to positive and highly significant correlation between these traits.
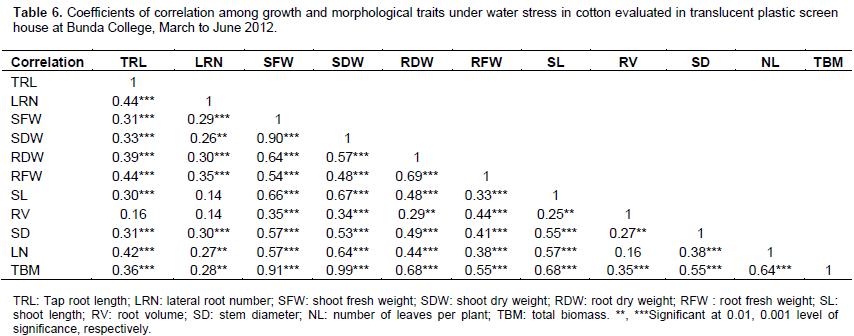
These traits showed significant correlation and strongest association with total biomass, revealing their importance for selecting genotypes with drought tolerance and higher biomass. The mentioned traits are easy and more practical to use for indirect selection. This gives breeders the opportunity to combine different growth characteristics to improve dry matter production. Paytas (2009) reported that any reduction in biomass production in cotton decreases final yield. Taproot length and lateral root number correlated significantly and positively with total biomass in this study. Kohel and Lewis (1984) noted that the correlations of taproot length and vigorous laterals with dry matter production suggested that root vigor may allow superior strains to be better competitors for limited soil water. In the current study, most of the parameters were significantly and positively correlated with each other, thereby providing a chance for selection of desirable genotypes with desirable traits.
Genotypic variation existed for growth and productivity traits in response to water stress under plastic translucent screen house, implying that selection for drought tolerance is possible. The significant and positive association of growth traits with total biomass implied that indirect selection for different morphological traits under water-limited conditions is possible. Overall, according to the current study, genotypes SPAN 837, 06K485, FQMA (05) 5 bcp, Chureza, 06K486, and RASAM 17 were the most tolerant to drought. In contrast, CHUMA (04) 17 bcp, Acala glandless, SZMA (04) 4 bcp, SZ9314, and IRM 81 were the most susceptible. Selecting tolerant cotton genotypes would assist to minimize the effect of drought on cotton in Malawi.
The authors have not declared any conflict of interests.
REFERENCES
Abayomi YA, Abidoye TO (2009). Evaluation of cowpea genotypes for soil moisture tolerance under screen house conditions. Afr. J. Plant Sci. 3(10):229-237.
|
|
Akıncı S, Lösel DM (2012). Plant water-stress response mechanisms. Retrieved from
View.
|
|
|
Ali MA, Jabran K, Awan SI, Awan A, Abbas A, Ullah E, Acet T, Farooq J, Rehman A (2011). Morpho-physiological diversity and its implications for improving drought tolerance in grain sorghum atdifferent growth stages. Austr. J. Crop Sci. 5(3):311-320.
|
|
|
Basal H, Smith CW, Thaxton PS, Hemphill JK (2005). Seedling drought tolerance in upland cotton. Crop Sci. 45:766-771.
Crossref
|
|
|
Basal H, Unay A (2006). Water stress in cotton (Gossypium hirsutum L.). Ege Üniv. Ziraat Fak. Derg. 43(3):101-111.
|
|
|
Bibi A, Sadaqat HA, Tahir MHN, Akram HM (2012). Screening of sorghum (Sorghum bicolor Var Moench) for drought tolerance at seedling stage in polyethylene glycol. J. Anim. Plant Sci. 22(3):671-678.
|
|
|
Brito GG, Sofiatti V, Lima MMA, Carvalho LP, Filho JLS (2011). Physiological traits for drought phenotyping in cotton. Acta Sci. Agron. 33:117-125.
Crossref
|
|
|
Chaturvedi GS, Anuradha S, Bahadur R (2012). Screening techniques for evaluating crop germplasm for drought tolerance. Plant Archives 12(1):11-18.
|
|
|
Food and Agriculture Organization of the United Nations (FAO) (1994). Cotton pests and their control in the near east. Rome: Food and Agriculture Organization of the United Nations.
|
|
|
Farshadfar E, Jalali S, Saeidi M (2012). Introduction of a new selection index for drought tolerance in common wheat (Triticum aestivum L.). Eur. J. Expt. Biol. 2 (4): 1181- 1187.
|
|
|
Fortucci P (2002). The contributions of cotton to economy and food security in developing countries.
|
|
|
Iqbal K (2010). The potential for breeding upland cotton under limited water conditions (PhD thesis). University of Agriculture, Faisalabad, Pakistan.
|
|
|
Iqbal K, Azhar FM, Khan IA and Ullah E (2011). Variability for drought tolerance in cotton (Gossypium hirsutum) and its genetic basis. Int. J. Agric. Biol. 1:61-66.
|
|
|
Kohel RJ, Lewis CF (1984). Cotton. Wisconsin. Crop Sci. Soc. America, Inc., Publishers.
|
|
|
Loka DA, Oosterhuis DM (2009). Effect of water-deficit stress on reproductive development in the cotton pistil. AAES Res. Series 582:7-43.
|
|
|
Mahmood S, Irfan M, Raheel F, Hussain A (2006). Characterization of cotton (Gossypium hirsutum L.) varieties for growth and productivity traits under water deficit conditions. Int. J. Agric. Biol. 8(6):796-800.
|
|
|
Martin JH, Waldren RP, Stamp DL (2006). Principles of field crop production. New Jersey. Pearson Prentice Hall.
|
|
|
Ministry of Agriculture and Food Security (MoAFS) (2005). Guide to Agricultural Production and Natural Resources Management in Malawi. Lilongwe, Malawi: Agricultural Communication Branch, Ministry of Agriculture and Food Security.
|
|
|
Ministry of Agriculture and Food Security (MoAFS) (2006). Cotton production in Malawi: Country report presented at the 65th international cotton advisory committee plenary meeting, 11-15 September 2006, Goiania, Brazil. Lilongwe: Ministry of Agriculture and Food Security.
|
|
|
Ministry of Mines, Natural Resources and Environment. (2006). Malawi's National Adaptation Programmes of Action (NAPA). (1st Ed.). Lilongwe, Malawi: Environmental Affairs Department, Ministry of Natural Resources, Energy and Environment.
|
|
|
Monneveux P, Ribaut JM (2011). Drought phenotyping in crops: from theory to practice. Retrieved from
View.
|
|
|
Pace PF, Cralle HT, El-Halaway SHM, Cothren JT, Senseman SA (1999). Drought-induced changes in shoot and root growth of young cotton plants. J. Cotton Sci. 3:183-187.
|
|
|
Paytas MJ (2009). Early water stress on growth, development and yield of high retention cotton (PhD thesis). University of Queensland. Retrieved from
View.
|
|
|
Poehlman JM, Sleper DA (1995). Breeding field crops. Ames, Iowa. Iowa State University Press.
|
|
|
Pretorius MM (2009). Evaluation of irrigated cotton cultivars in South Africa (MSc. Thesis). University of the Free State, South Africa.
|
|
|
Rathore KS (2007). Reducing gossypol in cottonseed may improve human nutrition. Texas A & M University, College Station, TX. Retrieved from
View.
|
|
|
Sarrantonio M (1991). Methodologies for screening soil-improving legumes. Pennsylvania. Rodale Institute. USA.
|
|
|
Sunilkumar G, Campbell LM, Puckhaber L, Stipanovic RD, Rathore KS (2006). Engineering cotton seed for use in human nutrition by tissue-specific reduction of toxic gossypol. Texas A&M University. Retrieved from
View.
|
|
|
Taiz L, Zeiger E (2006). Plant physiology. Massachusetts. Sinauer Associates, Inc.
|
|
|
United States Department of Agriculture (USDA) (2008). Oilseeds: World Markets and Trade.
|
|