ABSTRACT
Utilization of stress tolerant maize is key to sustainable production and food security. Limited knowledge on genetics of drought tolerance hampers development of drought tolerant varieties. The aim of this study was to study inheritance and combining ability of drought tolerance and grain yield among early inbred lines of maize. Five inbred lines were mated in full diallel in 2015 major season. The resultant 20 hybrids, 5 parents and 2 checks were evaluated under drought stress and well watered conditions in a screen house. Data was collected on days to 50% tasseling (DT 50%), days to 50% silking (DS 50%), anthesis-silking interval (ASI), leaf rolling, leaf senescence, plant aspect (PASP), plant height (PHT), ear height (EHT), ears per plant (EPP), ear weight, hundred grain weight (100 GW) and grain yield per hectare (GY). Drought condition was induced 40 days after planting (DAP) but watering was continued once per week till maturity. Results from combined ANOVA showed there was high and significant (p<0.01) level of genetic variability among parental lines and hybrids used in all the traits studied except DT 50%, PHT, EHT, EPP and 100 GW. Both additive and non-additive gene actions were important as well as GCA/SCA ratio variance. Lines TZEI-23 (215.22) and TZEI-25 (76.84) had the highest and highly significant (p<0.01) positive GCA effects for GY under drought. Hybrids TZEI-25 x TZEI-13 showed the highest positive and highly significant (p<0.01) SCA effects for GY (385.74) followed by its reciprocal TZEI-13 x TZEI-25 (311.49) under water stress. High broad sense heritability was observed for almost all the traits. High narrow sense heritability were observed DT 50% (0.69), DS 50% (0.80) and leaf senescence (0.61) under drought condition. Eighteen and nineteen hybrids had positive high parent heterosis (HPH) under water stress and well watered conditions, respectively for GY. The parents TZEI-23 and TZEI-25 were identified as the best general combiners respectively under drought and well watered conditions. The highest HPH were observed in many traits for TZEI-13 x TZEI-25 and TZEI-124 x TZEI-13. It is recommended these hybrids are further evaluated in different environments for release to farmers to increase yield.
Key words: Maize, inheritance, combining ability, drought, tolerance.
Maize (Zea mays L.; 2n = 20) belongs to the family Gramineae and tribe Maydeae or by others Andropogoneae (Norman et al., 1995). It occupies the second position after wheat in terms of area of production but represents the most important cereal crop in terms of quantity produced worldwide (FAOSTAT, 2017). In terms of consumption and cultivation, maize crop is very adaptable and versatile. In 2016, worldwide production of maize was around 1 billion ton, with America being the largest producer, which produces 51.6% equivalent to 547,416,865 tons and United States of America with 384,777,890 tons (FAOSTAT, 2017). Africa produces 6.7% and Nigeria is the largest African producer with 10,414,012 million tons (FAOSTAT, 2017). In 2016, Ghana produced 1,721,910 (FAOSTAT, 2017). According to Breisinger et al. (2008), maize is the most important cereal crop in Ghana in terms of consumption and production. In developed countries, maize crop is mainly used as an animal feed while it is largely used as a human consumption in developing countries (Badu-Apraku et al., 2012). In African, people consume maize as a starchy base in a wide variety of paste, beer grits and porridge. In sub-Saharan Africa, it is a stable food for around 50% of the total population (USAID, 2010). Maize crop is an important source of protein, minerals, vitamin B, iron, and carbohydrate (USAID, 2010). As a result of continuous shortage and unpredictability of rains in the dried areas of the words, possibly due to the effect of changes in climatic (Sodangi et al., 2011), research attention is being directed toward producing maize hybrids that can withstand moisture stressed ecologies. Despite the level of adaptation that maize crop displays and its potential in savanna ecology, low yield are still obtained due to biotic and abiotic stresses. According to Kamara et al. (2004), drought and low soil fertility are among the most important stresses threatening food security, maize production and economic growth. In dry savanna zone of West Africa, drought effect on food supplies and maize crop production are most severe (Fajemisin et al., 1985), due to the unpredictable rainfall in the region in terms of establishment, quantity, and distribution (Izge and Dugie, 2011).
In addition, recurrent drought is the single most important factor limiting maize production in West and Central Africa, with several billion U.S. dollars in production lost annually to this stress factor (Badu-Apraku et al., 2011). In Ghana, frequent drought stress is a major constraint in the largely rain-fed agricultural system that limits the production of maize (Mercer-Quashie et al., 1993; Ohemeng-Dapaah 1994; SARI, 1995; Obeng-Antwi et al., 1999). Drought stress has often been cited by farmers in Ghana as one of the major constraints to high maize productivity in the Coastal, Guinea and Soudan savanna zones in the major season and in the forest, coastal savanna and transition zones in the minor season. Research attention should be directed toward maize hybrids production that can tolerate drought stressed ecologies. Predominant maize types cultivated by farmers in West Africa are open pollinated cultivars where hybrids accounted for just over 9%, OPVs for 41%, and local cultivars for 50% (Tsedeke et al., 2017). Therefore, the need for breeding maize crop tolerant to drought condition for high yield is important. International Institute for Tropical Agriculture (IITA) has developed a wide range of maize germplasm which are adapted to the climate conditions of sub-Saharan African countries (Laouali, 2014). A large number of their inbred lines were developed under stress conditions such as striga, drought and low nitrogen, and some of their early inbreds were used as source of germplasm in this study. However, information on combining abilities, heritability and heterosis of these inbreds is limited. Thus, information regarding combining abilities, heritability and heterosis is essential for selection of suitable parents for hybridization and identification of promising hybrids for the development of improved varieties for a diverse agro-ecology. Such information is useful in developing stable hybrid with high yield. Therefore, this study was conducted to estimate heritability and combining ability and determine performance of parents (inbred lines) and hybrids under water stress and well watered conditions among early inbred lines of maize.
Experimental site and genetic materials
The experiment was conducted on the research field of the Department of Crop and Soil Sciences, KNUST, Kumasi, Ghana during the major season of 2015 (from April to July) to develop hybrids. Five tropical inbreds maize, developed by IITA, which are adapted to the climate conditions of sub-Saharan African countries were obtained. These inbreds are TZEI-13, TZEI-17, TZEI-23, TZEI-25 and TZEI-124 and were used in full diallel 5 × 5 mating design. All possible crosses were done among five inbred lines. The evaluation of the twenty diallel single crosses (Appendix 1) produce, the five inbreds parents and two checks (MAMABA and OMANKWA from Crop Research Institute, Kumasi Ghana) for drought tolerance was carried out in the screen house of the Department of horTiculture, KNUST, Kumasi Ghana during the major season of 2016 (from March to June). The site is located geographically on latitude 06°40’N and 01°34’W of the Greenwich meridian. Sandy loam (sand 68.32%) is the physical property of the soil used in the screen house for the study. The pH of soil was 5.00 which indicates a very acidic soil condition.
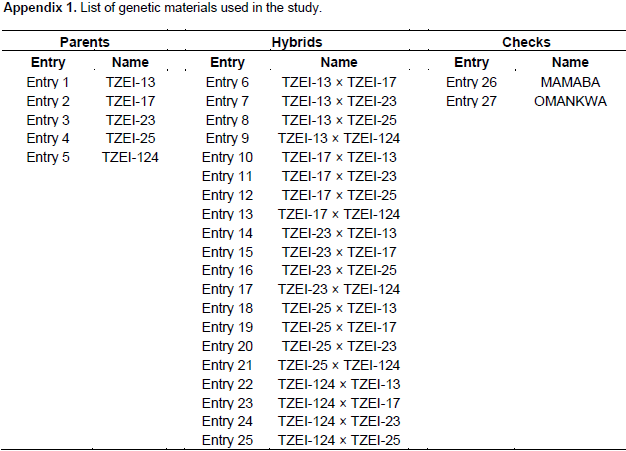
Experimental design
A Completely Randomized Design (CRD) was used for the evaluation of the 27 genotypes under managed drought and well watered conditions. The treatments were replicated three times. Each plot contained three pots. Therefore a total of 486 potted plants were evaluated. The water stress and the well-watered experiments were planted in the same plant house in two adjacent blocks.
Planting, stress management and quantity of water applied
Planting was done on 13th March, 2016. Water was withdrawn at 40 day after planting (one week before tasseling) from the water stress regime but watering continue once in the week, while irrigation was kept normally till eleventh week after planting for the well watered condition which coincided with end of the grain filling.
Fertilizer application and pest and weed control
All management practices were the same in both water stress and well watered conditions. Hand weeding was done at tenth, twentieth and thirtieth DAP to control weeds. NPK (15:15:15) was applied at a rate of 5 g to each pot at twelfth DAP followed by urea, also the same rate (5 g) at 32nd DAP.
Data collection
Data were collected on both treatments. Days to 50% tasseling (DT 50%) and days to 50% silking (DS 50%) were determined as a number of days that 50% of plant showed tassels and 50% of plants extracted silks. Anthesis-silking interval (ASI) was calculated as the difference between days to 50% silking and days to 50% anthesis. Leaf rolling (Leaf R) was scored by using a scale from 1 to 5 (Bänziger et al., 2000), 1 = unrolled, turgid, 2 = leaf rim stars to roll, 3 = leaf has the shape of V, 4 = rolled leaf rim covers part of leaf blade, 5 = leaf is rolled like an onion. Plant aspect (PASP) was recorded on a scale of 1 to 5 based on overall plant type, where: 1 = excellent plant type (desirable plant and ear characteristics), 2 = very good plant type, 3 = good plant type, 4 = tolerable plant type and 5 = poor plant type (undesirable plant and ear characteristics). The score of leaf senescence (Leaf S) was recorded on the scale from 1 to 10 by dividing the percentage of estimated total dead leaf area by 10 (Bänziger et al., 2000) as 1 = 0-10% dead leaf, 2 = 10-20% dead leaf, 3 = 20-30% dead leaf, 4 = 30-40% dead leaf, 5 = 40-50% dead leaf, 6 = 50-60% dead leaf, 7 = 60-70% dead leaf, 8 = 70-80% dead leaf, 9 = 80-90% dead leaf and 10 = 90-100% dead leaf. Plant height (PHT) and ear height (EHT) were measured from the base of the maize plant to the top of the largest leaf and ear leaf, respectively using measuring tape (cm) (Badu-Apraku et al., 2012). Ears per plant (EPP) was recorded as the total number of ears which developed at least one full grain and divided by the total number of all the plants harvested in the plot (Bänziger et al., 2000). Ear weight was recorded by dividing the weight of total ears in the plot that at least exists one fully grain by number of harvested ears. After bulking the grain results of all plants within the plot, hundred grains were counted and weighted. The results were given in gram (g) by using SARTORIUS scale. Grain yield per hectare (GY) was calculated by adjusting the actual moisture level of grain. The conversion of grain yield to grain moisture-standardized yield was calculated as follows (Badu-Apraku et al., 2012):

AQUA-BOY, KPM (moister tester) was used to determine moisture level of each sample.
Statistical analysis
Combined ANOVA was performed across all research conditions (drought and well watered) for DT 50%, DS 50%, ASI, leaf rolling, leaf senescence, PASP, PHT, EHT, EPP, ear weight, 100 grain weight and GY per hectare using a software Plant Breeding Tools version 1.4 of June 2014 (Griffing’s 1956) method 1 model 1. Mean squares and error were also computed. The GCA effects of inbreds and SCA effects of the hybrids as well as their mean squares in each environment, were estimated on the 5 × 5 diallel mating design, excluding the checks, following Griffing’s method 1 model 1 (fixed model), Griffing (1956) (Table 1) by using a software Plant Breeding Tools version 1.4 of June 2014. Standard errors for each parent and cross as well as LSD were computed. The program also computed genetic variance components (VA and VD). Also, GCA effects of inbreds and SCA effects of the hybrids as well as their mean squares were performed using Diallel SAS program developed by Zhang et al. (2005) modified in 2009 adopted to SAS software version 9.0. Means and coefficient of variation (CV) of all genotypes (parents, crosses and checks) were performed using GenStat (2009). High-parent heterosis (HPH) and mid-parent heterosis (MPH) were estimated according to the formulae given by Fehr (1987) as follow:
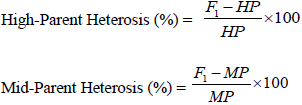
where HPH = High-Parent Heterosis, MPH = Mid-Parent Heterosis, F1 = Performance of hybrid, HP = Performance of best parent, and MP = Average performance of parents per se (parent 1 + parent 2)/2 Also, the estimate of genetic variability parameters and heritability were computed as follow:
(i) Genotypic and phenotypic variance as well as heritability in broad sense were computed using the formulae given by Singh and Chaudhary (1985) in case of one location.
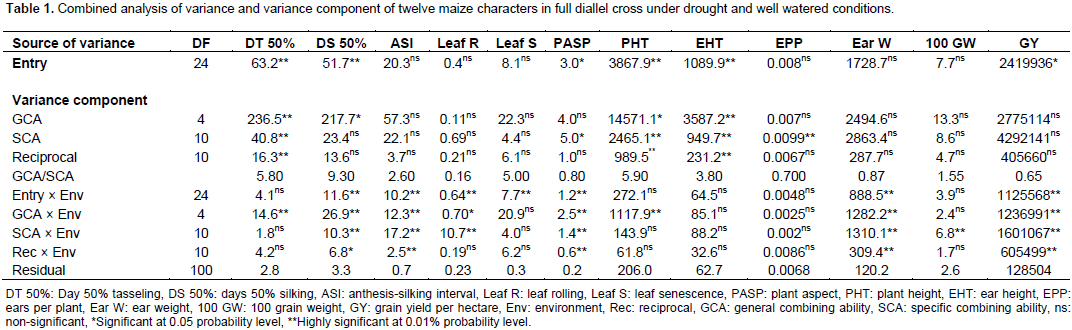
Broad sense heritability (h2b) = σ2g/ σ2ph × 100 For individual location, variance components were computed from mean squares and expected mean squares as follow:
σ2g = (MSg - MSe)/r
σ2e = MSe/r
σ2ph = σ2g + σ2e
where MSg = mean squares due to genotypes, σ2g = genotypic variance, MSe = error mean square, σ2e = error variance, r = number of replications, σ2ph = phenotypic variance.
(ii) Heritability in narrow sense was calculated by the formulae given by Grafius et al. (1952).
Narrow sense heritability (h2n) = (σ2f + σ2m)/( σ2f + σ2m + σ2fm + σ2e/r) × 100
where σ2f = genetic variance of female, σ2e = error variance, σ2m = genetic variance of male r = number of replication, σ2fm = genetic variance of females × males.
The heritability values were classified as low (<30%), moderate (30-60%) and high (>60%) according to Johnson et al. (1955). According to Singh and Chaudhary (1985), the estimates of variances due to GCAf, GCAm and SCA in one location werecomputed from mean squares as follow:
COV(H.S)f = σ2f = (MSf - MSfm)/rm
COV(H.S)m = σ2m = (MSm – MSfm)/rf
COV(F.S) = (MSfm – MSe)/r
where MSf = mean square of female, f = number of females, MSm = mean square of male, m = number of males, MSe = mean square of error, r = number of replications, MSfm = mean square of females × males.
The results of combined analysis of variance (ANOVA) for twelve maize traits studied under drought stress and well watered conditions are presented in Table 1. Mean squares for environments were highly significant (p<0.01) for all the traits except DT 50% and EEP, while only DT 50%, DS 50%, PHT and EHT were highly significant (p<0.01) for entries. The interaction effects of entry × environment, GCA × environment and SCA × environment were highly significant (p<0.01) for all the traits studied except DT 50%, leaf senescence, PHT, EHT, EPP and 100 seed weight. On the contrary, reciprocal × environment interaction effect was highly significant (p<0.01) for only ASI, PASP, ear weight and GY and significant (p<0.05) for DS 50%. Partitioning the entry mean squares into components showed that GCA mean squares was highly significant (p<0.01) for only DT 50% and EHT and significantly different (p<0.05) for DS 50% and PHT. Specific combining ability was significantly different (p<0.01) for DT 50%, PHT, EHT and EPP and significant (p<0.05) for PASP. All the characters showed more than unity GCA/SCA ratio values except leaf rolling, plant aspect, EPP, ear weight and GY. The results of GCA effects of inbreds in full diallel cross for eight traits under two conditions are presented in Table 2. Entry TZEI-23 exhibited highly significant (p<0.01) negative GCA (highest negative for DT 50% and DS 50%) effects under drought stress and well watered conditions for DT 50% (drought -4.02 and well watered -2.94) and DS 50% (drought -3.63 and well watered -2.07). In addition, this entry recorded also the highest highly significant (p<0.01) positive GCA effects for ear weight (3.47) and GY (215.22) under drought condition. Therefore in terms of GCA, for a set of the aforementioned traits, TZEI-23 comes out successful in general. On the contrary, TZEI-124 showed highly significant (p<0.01) positive GCA effect under water stress and well watered conditions for DT 50%, DS 50%, ASI, PHT and leaf senescence. Thus TZEI-124 was the lowest GCA effect in combination of these traits. TZEI-25 had the highest highly (p<0.01) negative general combining ability effect for ASI (-1.64) under drought condition. TZEI-13 recorded the highly negative significant (p<0.01) GCA for PHT (Table 2).
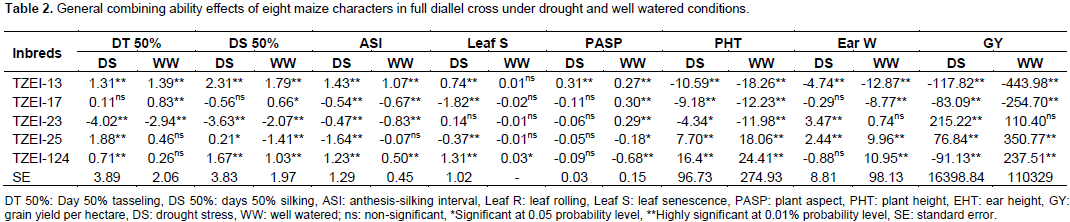
Considering the lines individually for each trait under the two conditions TZEI-13 was the best donor for plant height. In terms of grain yield and its component, TZEI-23 had the highest and highly positive (p<0.01) GCA under drought condition for ear weight (3.47) and grain yield per hectare (215.22) while under well watered condition TZEI-25 showed the highly positive GCA for grain yield (350.77). TZEI-25 exhibited highly significant positive GCA under both conditions for ear weight (2.44 and 9.96) and grain yield (76.84 and 350.77). Therefore, TZEI-23 was the best general donor for ear weight and GY under drought while TZEI-25 was the best general donor for ear weight and GY under well watered condition (Table 2). The estimates of SCA of eight characters of twenty hybrids (set of F1’s and reciprocal F1) under drought stress and well watered conditions are presented in Table 3. Hybrids TZEI-25 × TZEI-13 showed the highest positive and highly significant (p<0.01) SCA effects for GY (385.74) followed by its reciprocal TZEI-13 × TZEI-25 (311.49) under water stress while under well watered condition, TZEI-13 × TZEI-124 (1132.01), TZEI-17 × TZEI-13 (789.01) and TZEI-17 × TZEI-124 (789.01) were the highest and were highly significant (p<0.01) (Table 3). Cross TZEI-17 × TZEI-25 was the only one that exhibited best performance and showed highly positive and significant (p<0.01) SCA across both environments (218.76 under drought and 655.38 under well watered condition) for GY. For the reproductive traits, only entry TZEI-17 × TZEI-13 exhibited the highest and highly significant (p<0.01) negative SCA for DT 50%, DS 50% and ASI. However, TZEI-23 × TZEI-124 was the best in terms of SCA effect for DT 50% and DS 50% under water stress and well watered conditions, respectively. For ASI only TZEI-13 × TZEI-25 (-0.73), TZEI-13 × TZEI-124 (-1.13) and TZEI-17 × ZEI-13 (-1.67) showed highly negative significant SCA and TZEI-124 × TZEI-17 (-0.67) showed negative and significant (p<0.05) under well watered condition (Table 3).
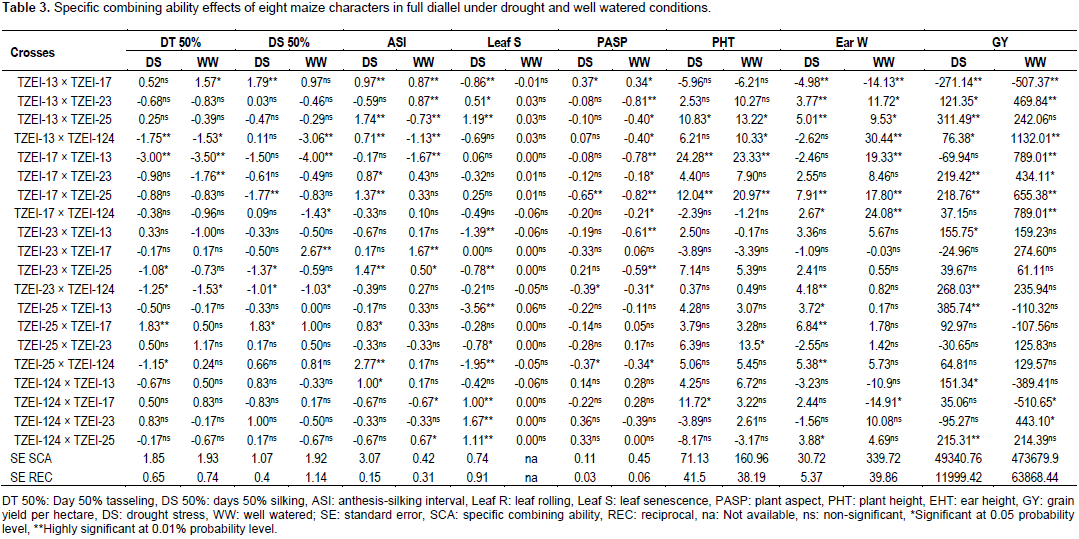
For leaf senescence under drought, five crosses showed highly negative significant (p<0.01) ranged from -0.78 (TZEI-23 × TZEI-25) to -3.56 (TZEI-25 × TZEI-13), whereas one cross TZEI-25 × TZEI-23 (-0.78) exhibited negative significant (p<0.05) SCA. For PHT, either under drought or normal conditions, only two hybrids TZEI-17 × TZEI-124 (-1.94) and TZEI-23 × TZEI-124 (-1.57) showed negative significant (p<0.05) SCA. Results from both narrow sense and broad sense heritability estimates in this study under drought stress and well watered conditions are presented in Table 4. Environment played its role in modifying narrow sense heritability while heritability in broad sense was not much influenced by environment. The heritability values were classified as low (<30%), moderate (30-60%) and high (>60%) according to Johnson et al. (1955). The estimates of narrow sense heritability were high for only DT 50% (0.69), DS 50% (0.80) and leaf senescence (0.61) under drought while for plant height (0.65) under well watered condition (Table 4). It was moderate (0.50) for DT 50% under well watered. High magnitudes of broad sense heritability were found in all characters under both water stress and well watered conditions except for leaf senescence (0.12) under well watered condition and hundred grain weight under both conditions (0.56 each). The following characters had high heritability above greater or equal to 0.90% under drought stress; DT 50% (0.94), DS 50% (0.90), ASI (0.96), leaf senescence (0.96), EHT (0.90), ear weight (0.92) and GY (0.94) while under well watered ASI (0.94), PASP (0.93), PHT (0.91), ear weight (0.91) and GY (0.93) had broad sense heritability greater or equal to 0.90%.
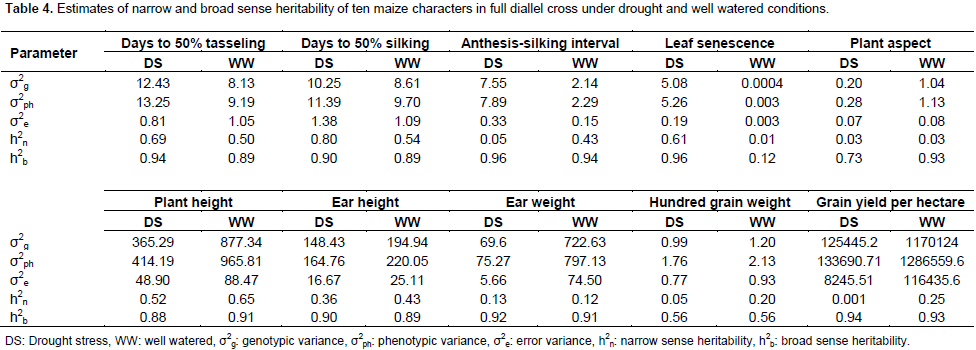
The estimates of high parent heterosis (HPH) were computed for GY and yield related traits under water stress and well watered conditions as presented in Table 5. The results revealed that hybrids TZEI-25 × TZEI-17 (-5.93% and -5.87% for DT 50% and -6.49% and -1.42% for DS 50%) and TZEI-124 × TZEI-13 (-7.04% and –9.30% for DT 50% and -0.60 and -11.60 for DS 50%) showed negative HPH for days to 50% tasseling and silking under both water stress and well watered conditions. However, hybrids TZEI-13 × TZEI-124 (-9.85%) and TZEI-124 × TZEI-17 (-9.35%) showed the highest negative high parent heterosis for DT 50% under drought stress and well watered conditions, respectively and TZEI-25 × TZEI-17 (-6.49%) and TZEI-13 × TZEI-124 (-12.80%) performed also the highest high parents heterosis for DS 50% under drought stress and well watered conditions, respectively. Regarding these results, hybrids TZEI-25 × TZEI-17 and TZEI-124 × TZEI-13 came out as the best for days to DT 50% and DS 50% under both water stress and well watered conditions. For ASI, four hybrids with line TZEI-25 as female parent exhibited negative high parents heterosis under drought but 16 under well watered. The inbred TZEI-25 gave maximum heterosis under well watered when used either female or male parent, it performed well under drought when used as female.
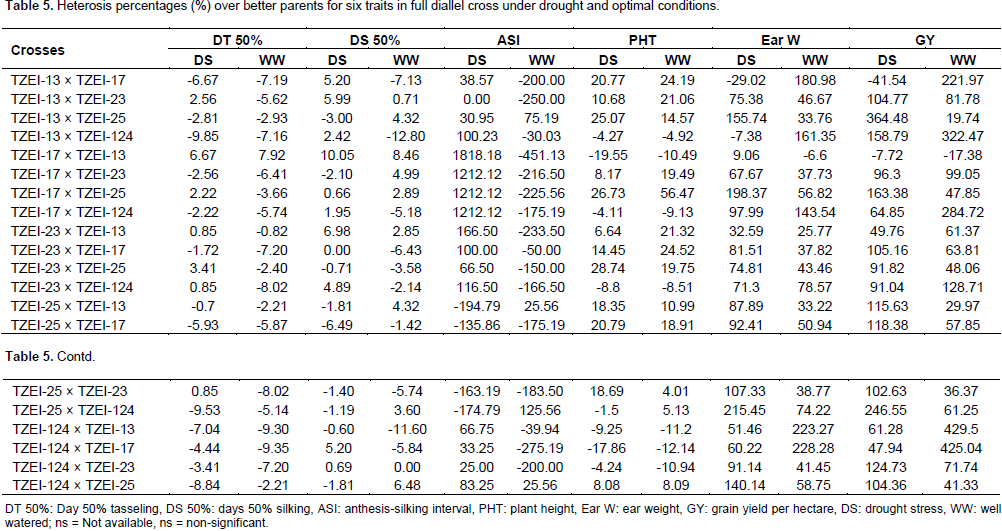
Eight and seven hybrids expressed negative high parent heterosis under both water stress and well watered conditions for plant height with TZEI-17 × TZEI-13 (-19.55 and -10.49%) and TZEI-124 × TZEI-17 (-17.86 and -12.14%) having the highest negative high parent heterosis. The lines TZEI-17 gave maximum heterosis in various cross combination when used as either female or male. Eighteen hybrids had positive HPH under water stress and ranged from 47.94% (TZEI-124 × TZEI-17) to 364.48% (TZEI-13 × TZEI-25) while under well watered condition 19 hybrids had positive HPH and ranged from 19.74% (TZEI-13 × TZEI-25) to 429.50% (TZEI-124 × TZEI-13) for GY. For ear weight 18 and 19 hybrids under water stress and well watered conditions, respectively, exhibited positive HPH. The values ranged from 9.06% (TZEI-17 × TZEI-13) to 215.45% (TZEI-25 × TZEI-124) under drought condition and from 25.77% (TZEI-23 × TZEI-13) to 228.28% (TZEI-124 × TZEI-17) under well watered condition. All the heterosis percentage above 100% under drought for ear weight were obtained when TZEI-25 was used either as female or male parent, whereas under normal condition TZEI-13, TZEI-17 and TZEI-124 were used as parents, hence under water stress TZEI-25 gave the maximum heterosis. TZEI-13 × TZEI-25 (364.48%) and TZEI-25 × TZEI-124 (246.55%) expressed the highest higher positive heterosis for GY under drought stress and TZEI-124 × TZEI-13 (429.50%) and TZEI-124 × TZEI-17 (425.04%) under well watered condition. TZEI-25 gave the maximum heterosis under drought condition when used either female or male parents while TZEI-124 gave the maximum under well watered condition when used as female.
Highly significant (p<0.01) environment mean squares for all the traits in the current study was observed except DT 50% and EPP. Similar results were published from other studies, such as grain yield (Doerksen et al., 2003; Laouali, 2014), DS 50% (Zare et al., 2011), leaf senescence (Badu-Apraku, 2011), plant aspect (Laouali, 2014) and ASI, PHT, EHT, leaf rolling (Premlatha and Kalamani, 2010; Aminu and Izge, 2013; Aminu et al., 2014a; Murtadha et al., 2016). This indicated that these traits were highly influenced by environmental factors and there is adequate genetic variability among the inbred lines to allow good progress from selection for improvement in the traits (Badu-Apraku et al., 2011). The significance of the entry in DT 50%, DS 50%, PHT, EHT and GY indicated that there was possibility for the improvement of these traits through selection (Badu-Apraku et al., 2011). Significant (p<0.05) and highly significant (p<0.01) GCA × environment, SCA × environment, reciprocal × environment and entry × environment mean squares for the following characters; DS 50%, ASI, PASP, ear weight and GY indicate that there is a significant variation in the combining ability of the inbred under different environmental conditions (Badu-Apraku et al., 2005, 2007, 2011). This exhibited that the potential performance of the inbreds and the F1 hybrids was affected by the frequency and the amount of water applied to each condition. Thus testing inbred lines (parents) under different environmental conditions will ensure selection of stable parents that can perform the potential of that environment (Machado et al., 2009; Murtadha et al., 2016) or interested in the influence of environment in phenotypic expression of traits (Bello and Olaoye, 2009; Murtadha et al., 2016).
The predominance of GCA mean square over SCA mean square indicates that additive genetic action was more important than non-additive genetic action. The results of seven traits corroborate the findings of Sharma et al. (2004) and Aminu and Izge (2013) who found the predominance of additive genetic effects in maize traits control. However, these results are in disagreement with the findings of other researchers such as Abdel-Moneam et al. (2009), Machado et al. (2009), Aminu et al. (2014a, b) and Murtadha et al. (2016) who reported the predominance of non-additive gene effects for DT 50%, DS 50%, ASI, leaf rolling, PHT, EHT and GY. For grain yield, results found in this study showed the predominance of SCA mean square over GCA mean square which indicates that non-additive genetic action was more important than additive genetic action. However, it contradicts finding of Ojo et al. (2007) who found the preponderance of additive genetic action for grain yield. The estimates of GCA effects of five parents used in this study revealed that none of the parents had good GCA for all the traits either under one or both environmental conditions, thus the exhibition of variation both in direction and magnitude. However, comparing the parents with each other, high significance negative GCA observed by TZEI-23 for DT 50% and DS 50% and by TZEI-13 for PHT, can rank TZEI-23 as the best combiner in breeding program for early maturity and TZEI-13 for resistance to drought. Besides inbred lines with high negative GCA effects for DT 50%, DS 50% and PHT are desirable for selection under drought environment as these parents could escape drought. Similar results were reported by Izge et al. (2007), Aminu and Izge (2013) and Aminu et al. (2014a).
Furthermore, TZEI-23 and TZEI-25 were good donors for ear weight and grain yield. This suggests that inbreds possess high frequency of favorable genes for selection of grain. Similar result has been reported by Haydar and Paul (2014). In addition, parents which showed good GCA for at least one trait can be as good donor parents for the accumulation of favorable genes. This result corroborated the findings of Khalil et al. (2010), Singh et al. (2012), and Haydar and Paul (2014). The worst general combiner for both reproductive traits and grain yield was TZEI-17 which did not exhibit even one best performance in any trait either under drought or well watered condition. The general best combiner was ranked as followed: TZEI-23 > TZEI-25 > TZEI-124 > TZEI-13 > TZEI-17. The high estimates of SCA for GY for TZEI-17 × TZEI-25, TZEI-25 × TZEI-13 and TZEI-13 × TZEI-124 under water stress and well watered conditions suggest these hybrids as good combiners and their selection would lead to improvement in these characters. However, hybrid TZEI-13 × TZEI-17 which showed higher negative SCA effects for GY indicates the unsuitability of both parents as good specific combiners for grain yield. This report supported the findings of Pswarayi and Vivek (2008) and Murtadha et al. (2016) who also observed differences in the expression of GCA and SCA with stress. Under both conditions, TZEI-17 × TZEI-25 was the most promising cross for improving GY followed by TZEI-13 × TZEI-23 due to the highest positive SCA on one hand and high significant SCA on other hand for the following characters GY and ear weight. In addition, in this study, the highest SCA effects for GY were obtained from high × low and low × high combiners in the crosses TZEI-25 × TZEI-13 and TZIE-13 × TZEI-25 under drought condition. However, low × high were observed in TZEI-13 × TZEI-124 and TZEI-17 × TZEI-124 under well watered condition.
Results are in agreement with those obtained from Alam et al. (2008), Singh et al. (2012) and Haydar and Paul (2014) who reported that the superiority of high × low or average × low could be explained on the basis of interaction between positive alleles from good/average combiners and negative alleles from the poor combiners as parents. The high yield of such hybrids would be non-fixable and thus could be exploited for heterosis breeding. Some of the hybrids were obtained from low × low general combiners as in the case of TZEI-17 × TZEI-13. This supported the results of Premlatha and Kalamani (2010) and Aminu and Izge (2013). Hallauer and Miranda (1988) and Majid et al. (2010) reported that in low × low GCA combination, the superior cross could result from over dominance or epistasis. Such type of gene action may be exploited in cross-pollinated species like maize. For reproductive traits, parents TZEI-124 was the best combiner, meanwhile it was one of the two worst parents. The performance of this hybrid can be explained by the fact that it was the cross from parents with high GCA. Therefore most of the superior hybrids were from either one of the parents with high GCA effect or parents that are low × low general combiners and suggests that the parents with either high GCA and/or low SCA would have a higher chance of having excellent complementary genes with other parents that have high general combining ability. This supports results of Premlatha and Kalamani (2010) and Aminu and Izge (2013). Even though, some few crosses showed negative and significative SCA effect under both environments in respect of plant height, negative values of SCA in these traits mostly under drought are desirable as found in the studies of Aminu et al. (2014a) and Umar (2015) who reported that negative SCA effects in stress environments for plant and ear height are desirable especially in drought prone and windy areas against water stress and lodging.
Percentage of heritability in narrow sense greater than 50% recorded indicates that these traits were controlled by additive gene action. Only days to 50% tasseling under well watered condition exhibited 0.5 narrow sense heritability and this suggests that both additive and dominance gene action are important in influencing the expression of this trait. The relatively low narrow sense heritability recorded in almost all the traits in both environmental conditions were less than 0.5. This indicates that the expressions of the traits are mainly controlled by dominance genes. Similar results were reported by Umar (2015) who said that the best exploitation of this type of gene action would be in F1 hybrids implying that breeding gains can be made through selfing than cross breeding, with selection being made in later generation. In this study, the heritability reported for grain yield under drought stress was lower than that reported by Bolanos and Edmeades (1996) which was 40% under drought and 60% under well watered condition. However, Falconer and Mackay (1996) reported that the magnitudes of heritability estimates are products of the traits being measured, the population being tested and the environments within which the testing is done. Therefore, the variation observed in magnitudes here are the results of the differences in the population, environment and trait of the heritability estimates. It should therefore be understood that heritability values reported for a given character, are specific to a particular population under particular environmental condition (Hallauer and Miranda, 1981). Hence, it would be better to evaluate genotypes in different target environments. All the results of heritability in broad sense showed high magnitude and this is in line with the results of studies of independent researchers such as Kashiani et al. (2008), Wannows et al. (2010), Olakojo and Olaoye (2011) and Umar (2015). This revealed that variations were transmitted to the progeny and implied the effective selection for genetic improvement of these characters. Hence, provides better opportunities for selection of plant material regarding these traits. This is in line with the results of early workers; Kashiani et al. (2008), Wannows et al. (2010), Bello et al. (2012) and Aminu et al. (2014c). Under water stress, the decreased heritability of traits indicates the need for selection of genotypes under particular environment for rapid genetic improvement.
This agrees with the findings of Bolanos and Edmeades (1996) who reported decreased heritability under drought. The negative HPH observed in days to 50% tasseling and silking indicate that these hybrids are desirable candidates for earliness since it has been reported that maize crop is most susceptible at flowering under drought stress (Claassmen and Shaw, 1970; Grant et al., 1989). Therefore, hybrid that can tassel and produce silk early can take advantage in a drought environment due to the fact that it could escape drought. For ASI only, all the four crosses with line TZEI-25 as female exhibited the highest negative HPH under drought. High negative value for DT 50%, DS 50% and ASI are actually desirable and this can be explained as these hybrids could escape drought. This result is in line with the findings of Izge and Dugie (2011), Aminu et al. (2014c) and Umar (2015). The high level of HPH has also been recorded by Umar (2015) who reported that these crosses which featured prominently in the expression of higher level heterosis could form an initial gene pool for further breeding program in developing high yielding varieties for cultivation in the Savannas. Negative values for HPH for PHT are desirable in breeding for drought tolerance. Therefore, selection of hybrids showed that negative value is important as it implied that these hybrids could resist lodging confirming the results of Aminu et al. (2014c). High positive HPH were obtained for grain yield per hectare and ear weight. Positive HPH is actually desirable in GY and ear weight. High heterotic values for grain yield have also been reported by Joshi et al. (2002), Ojo et al. (2007), Amanullah et al. (2011) and Aminu et al. (2014a). Therefore, these hybrids could contain genes that could be introgressed to exploit heterosis for earliness and high grain yield. Similar results were reported by Kumar et al. (1998), Joshi et al. (1998), Bello and Olaoye (2009) and Aminu et al. (2014a).
The study revealed that both additive and non-additive gene actions were important in controlling GY and other characters in maize, and additive gene action was more important in controlling most of the traits. The best exploitation would be in F1 hybrids implying that breeding gains can be made through selfing than cross breeding. TZEI-23 and TZEI-25 were identified as the best general combiners respectively under drought and well watered conditions. These parents could be more useful in hybridization programs with those parents with low combining abilities. Narrow sense heritability was high, medium and low. High value of heritability indicates considerable potential for development of drought tolerance and high yielding varieties through selection of desirable traits in succeeding generations. TZEI-13 × TZEI-25 in GY, ear weight, PAST, leaf senescence, DT 50% and DS 50%, and TZEI-124 × TZEI-13 in GY, ear weight, PHT, PAST, DT 50% and DS 50% had high parent heterosis.
The authors have not declared any conflict of interest.
The financial support of this research by AGRA is gratefully acknowledged. INRAN Niger and KNUST Kumasi are also gratefully acknowledged.
REFERENCES
Abdel-Moneam MA, Attia AN, El-Emery MI, Fayed EA (2009). Combining ability and heterosis for some agronomic traits in crosses of maize. Pak. J. Biol. Sci. 12(5):433-438.
Crossref
|
|
Alam AKMM, Ahmed S, Begun M, Sultan MK (2008). Heterosis and combining ability for grain yield and its contributing characters in maize. Bangladesh J. Agric. Res. 33(3):375-379.
Crossref
|
|
|
Amanullah SJ, Muhammad M, Muhammad AK (2011). Heterosis study in diallel crosses of maize. Sarhad J. Agric. 27(2): 5.
|
|
|
Aminu D, Izge AU (2013). Gene action and heterosis for yield and yield traits in maize (Zea mays L.), under drought conditions in northern guinea and sudan savannas of Borno State. Peak J. Agric. Sci. 1(1):17-23.
|
|
|
Aminu D, Garba YM, Muhammad AS (2014a). Combining ability and heteroris for phonologic and agronomic traits in maize (Zea mays L.) under drought conditions in the Northern guinea savanna of Borno State, Nigeria. University of Maiduguri, Nigeria.7p.
|
|
|
Aminu D, Mohammed SG, Jaliya MM (2014b). Gene action and Heterosis for Yield and other agronomic traits in Maize (Zea mays L.), Under Drought Conditions in Northern Guinea and Sudan Savannas zones of Borno State, Nigeria. Int. J. Adv. Biol. Res. 4(2):130-136.
|
|
|
Aminu D, Mohammed FK, Gambo FM (2014c). Heritability and correlation coefficients analysis of maize (Zea mays L.) agronomic traits for drought tolerance in savanna zone of Borno State, Nigeria. Sci. J. Agric. Res. Manage. P 4.
|
|
|
Badu-Apraku B, Fakorede MAB, Menkir A, Sanogo D (2012). Conduct and management of maize field trials. IITA, Ibadan, Nigeria. 59 p.
|
|
|
Badu-Apraku B, Oyekunle M, Akinwale RO, Fontem LA (2011). Combining ability of early- maturing white maize inbreds under stress and non-stress environments. Agron. J. 103(2).
Crossref
|
|
|
Badu-Apraku B (2007). Genetic variances and correlations in early tropical white maize population after three cycles of recurrent selection for Striga resistant. Maydica 52:205-217.
|
|
|
Badu-Apraku B, Menkir A, Lum AF (2005). Assessment of genetic diversity in extra- early Striga resistant tropical inbred lines using multi-variate analysis of agronomic data. J. Genet. Breed. 59:67-80.
|
|
|
Bola-os J, Edmeades GO (1996). The importance of the anthesis-silking interval in breeding for drought tolerance in tropical maize. Field Crops Res. 48:65-80.
Crossref
|
|
|
Bänziger M, Edmeades GO, Beck D, Bellon M (2000). Breeding for drought and nitrogen stress tolerance in maize. From theory to practice. CIMMYT, Mexico.
|
|
|
Bello OB, Ige SA, Azeez MA, Afolabi MS, Abdulmaliq SY, Mahamood J (2012). Heritability and genetic advance for grain yield and its component characters in Maize (Zea mays L.). Int. J. Plant Res. 2(5):138-145.
Crossref
|
|
|
Bello OB, Olaoye G (2009). Combining ability for maize grain yield and other agronomical characters in a tropical southern Guinea savanna ecology of Nigeria. Afr. J. Biotechnol. 8(11):2518-2522.
|
|
|
Breisinger C, Diao X, Thurlow J, Hassan R (2008). Agriculture for Development in Ghana. International Food Policy Research Institute and University of Ghana, Paper to be presented at the PEGNet Conference. Assessing Development Impact - Learning from Experience. Accra (Ghana), 11-12 September 2008 P 2.
|
|
|
Claassen MM, Shaw RH (1970). Water deficit effects on corn II. Grain components. Agron. J. 62:652-655.
Crossref
|
|
|
Doerksen TK, Kannenberg LW, Lee EA (2003). Effect of recurrent selection on combining ability in maize breeding populations. Crop Sci. 43:1652-1658.
Crossref
|
|
|
Fajemisin JY, Efron SK, Kim, FH, Khadr ZT, Dabrowsk J.H, Mareck M, Bjarnason V, Parkison LA, Evarett A, Diallo AO (1985). Population and varietal development in maize for tropical Africa through resistance breeding approach. In: A. Brondol in and F. Salamini (eds), Breeding strategies for maize production improvement in the tropics. FAO and Institutor Agronomico per Ľollremare, Florence. Italy. pp. 3854-407.
|
|
|
Falconer DS, Mackay TFC (1996). Introduction to Quantitative genetics. 4th ed. Longman Technical, Essex, UK. P 464.
|
|
|
FAOSTAT (2017). Production of commodity in selected country, production share by region and production of top 5 producers. [Available online] http://faostat3.fao.org
|
|
|
Fehr WR (1987). Principles of cultivar development. Macmillan publishing company. A division of Macmillan Inc. New York. 1:1-465. GenStat (2009). 12e edition. VSN international Ltd. 5 The Waterhouse, Waterhouse Street, Hemel Hempstead, Hertfordshire HP1 1ES, UK.
|
|
|
Grant RF, Jackson BS, Kiniry JR, Arkin GF (1989). Water deficit timing effects on yield components in maize. Agron. J. 81:61-65.
Crossref
|
|
|
Griffing B (1956). Concept of general and specific combining ability in relation to diallel crossing system. Austr. J. Biol. Sci. 9:463-493.
Crossref
|
|
|
Grafius JE, Nelson WL, Dirks VA (1952). The heritability of yields in barley as measured by early generation bulked progenies. Agron. J. 44:253-257.
Crossref
|
|
|
Hallauer AR, Miranda JB, (1981). Quantitative Genetics in Maize Breeding. First edition. Lowa State University Press. Ames lowa. pp. 267-292.
|
|
|
Hallauer AR, Miranda JB (1988). Quantitative Genetics in Maize Breeding 2nd ed. Iowa State University Press Ames USA. pp. 468.
|
|
|
Haydar FMA, Paul NK (2014). Combining ability analysis for different yield components in maize (Zea mays L.) inbred lines. University of Rajshahi, Bangladesh J. PIant Breed. Genet. 27(1):17-23.
|
|
|
Izge AU, Dugie IY (2011). Performance of drought tolerance three-way and top cross maize hybrids in Sudan savanna of north eastern Nigeria. J. Plant Breed. Crop Sci. 3(11):269-275.
|
|
|
Izge AU, Kadams AM, Gundula DT (2007). Heterosis and inheritance of quantitative characters in diallel cross of pearl millet (Pennisetum glaucum L.). J. Agron. 6(2):278-285.
Crossref
|
|
|
Johnson JL, Robinson HF, Comstock RS (1955). Estimates of genetic and environmental variability in soybeans. Agron. J. 47:314-318.
Crossref
|
|
|
Joshi VN, Dubey RB, Marker S (2002). Combining ability for polygenic traits in early maturity hybrids of maize (Zea maize L.). Ind. J. Genet. Plant Breed. 62:312-315.
|
|
|
Kamara AY, Menkir A, Fakorede MAB, Ajala SO, Badu-Apraku B, Kure I (2004). Agronomic performance of maize cultivars representing three decades of breeding in the Guinea Savannas of West and Central Africa. J. Agric. Sci. 142:1-9.
Crossref
|
|
|
Kashiani P, Saleh G, Abdullah SN, Abdullah NAP (2008). Performance, heritability correlation studies on nine advance sweet corn inbred lines. Proceeding of the 10th Symposium of Malaysian Society of Applied Biology, Nov. 6-8, 2008. Malaysia, pp. 48-49.
|
|
|
Khalil IA, Rahman H, Saeed N, Khan NU, Durrishawar NI, Ali F, Saeed M (2010).Combining ability analysis in maize single cross hybrids for grain yield: A graphical analysis. Sarhad J. Agric. 26(3):373-379.
|
|
|
Kumar A, Gangashetti MG, Kumar N (1998). Gene effects in some metric traits of maize. Ann. Agric. Biol. Res. 3:139-143.
|
|
|
Laouali MN (2014). Combining ability and yield performance studies of tropical maize (Zea mays L.) germplasm under drought stress and well watered environments. PhD thesis. University of Ghana, Legon. 66-99p.
|
|
|
Machado JC, de Souza JC, Ramalho MAP, Lima JL (2009). Stability of combining ability effects in maize hybrids. Sci. Agric. (Piracicaba, Braz). 66(4):494-498.
|
|
|
Majid S, Rajab C, Esman M, Farokh D (2010). Estimation of combining ability and gene action in maize using line x tester method under three irrigation regimes. J. Res. Agric. Sci. 6:19-28.
|
|
|
Mercer-Quarshie H, Amankwatia YO, Twumasi-Afriyie S, Marfo KO (1993). Crops Research Institute Medium Term Plan 1993-1997. Crops Research Institute, Kumasi.
|
|
|
Murtadha MA, Ariyo OJ, Alghamdi SS (2016). Analysis of combining ability over environments in diallel crosses of maize (Zea mays L.). J. Saudi Soc. Agric. Sci.
|
|
|
Norman MJT, Pearson CJ, Searle PGE (1995). The ecology of Tropical food crops, 2nd ed. Cambridge University Press, New York. NY USA. pp. 127-140.
Crossref
|
|
|
Obeng-Antwi K, Sallah PYK, Frimpong-Manso PP (1999). Performance of some maize genotypes in drought stress and non-stress environments. Ghana J. Agric. Sci. 35:49-57.
|
|
|
Ohemeng-Dapaah S (1994). Analysis of daily rainfall data and its application to maize production in the transitional zone of Ghana. pp 173-179. In Progress in food grain research and production in semi-arid Africa (Eds). Proceedings, SAFGRAD Inter-works Conference, Niamey, Niger, 7-14 March, 1991.
|
|
|
Ojo GOS, Adedzwa DK, Bello LL (2007). Combining ability estimates and heterosis for grain yield and yield component in maize (Zea mays L.). J. Sustain. Dev. Agric. Environ. 3:49-57.
|
|
|
Olakojo SA, Olaoye G (2011). Correlation and heritability estimates of maize. Afr. J. Plant Sci. 5(6):365-369.
|
|
|
Plant Breeding Tools (2014). Version 1.4. Biometrics and Breeding Informatics Plant Breeding, Genetics and Biotechnology Division. International Rice Research Institute.
|
|
|
Premlatha M, Kalamani A (2010). Heterosis and combining ability studies for grain yield and growth characters in maize (Zea mays L.). Ind. J. Agric. Res. 44(1):62-65.
|
|
|
Pswarayi A, Vivek BS (2008). Combining ability amongst CIMMYT's early maturing maize (Zea mays L.) germplasm under stress and non-stress conditions and identification of testers. Euphytica. 162:353-362.
Crossref
|
|
|
SARI (1995). Report of the workshop and planning session for the Upper West Region. Savanna Agriculture Research Institute. 1995.
|
|
|
Sharma S, Narwal MS, Kumar R, Dass S (2004). Line x Tester analysis in maize (Zea mays L.). Forage Res. 30:28-30.
|
|
|
Singh PK, Singh AK, Shahi JP, Rahman R (2012). Combining ability and heterosis in quality protein maize. The Bioscan 7(2):337-341.
|
|
|
Singh RK, Chaudhary BD (1985). Biometrical methods in quantitative genetic analysis. Kalyani publishers, New Delhi, India. pp. 67-78, 69-77, 205-207.
|
|
|
Sodangi IA, Izge AU, Maina TY (2011). Change in Climate: Causes and effects on African Agriculture. J. Environ. Issues Agric. Dev. Countries 3(3):22-33.
|
|
|
Tsedeke A, Monica F, Tahirou A, Girma TK, Rodney L, Paswel M, Woinishet A (2017). Characteristics of maize cultivars in Africa: How modern are they and how many
|
|
|
Umar U (2015). Genetics of drought tolerance in maize (Zea mays. L) under non-stress and water stress conditions. PhD thesis. Ahmadu Bello University. Zaria, Nigeria. pp. 131-142.
|
|
|
United States Agency for International Development (USAID) (2010). Packages of practice for maize production. United States Agency for International Development. P 23.
|
|
|
Wannows AK, Azzam HA, Al-Ahmad SA (2010). Genetic variances, heritability, correlation and path coefficient analysis in yellow maize crosses. Agric. Biol. J.f North Am. 1(4):630-637.
|
|
|
Zare M, Choukan R, Heravan EM, Bihamta MR, Ordookhani K (2011). Gene action of some agronomic traits in corn (Zea mays L.) using diallel cross analysis. Afr. J. Agric. Res. 6(3):693-703.
|
|
|
Zhang Y, Kang MS, Lamkey KR (2005). DIALLEL-SAS05: A comprehensive program for Griffing's and Gardner-Eberhart analyses. Agron. J. 97:1097-1106.
Crossref
|
|