ABSTRACT
Cowpea is an important source of food and income for small scale farmers in Uganda. Production is, however, affected by both biotic and abiotic stresses. Drought stress has recently emerged as a serious concern due to the effects of climate change. This study was therefore undertaken to estimate the general and specific combining ability effects of parents and crosses as well as estimate the heritability of delayed leaf senescence, seed yield and its components under drought stress. Five drought tolerant genotypes were crossed with four drought sensitive genotypes in a North Carolina II mating design. The study revealed that drought tolerance is conditioned by both additive and non-additive genetic effects with the predominance of non-additive genetic effects for seed yield, 100 seed weight and number of pods per plant. Delayed leaf senescence was however, controlled by additive genetic effects, implying that progenies performance could be predicted from parents General Combining Ability (GCA) effects. The cultivars SECOW 5T, IT93K-452-1 and IT98K-205-8 were good combiners for drought tolerance. The F2 families: SECOW 3B x IT98K-205-8, SECOW 5T x IT98K-205-8, SECOW 4W x IT98K-205-8 and SECOW 1T x IT98K-205-8 had positive Specific Combining Ability(SCA) effects in seed yield, number of pods per plant and 100 seed weight, implying that they performed better than what was predicted by their parents GCA effect. As such, they are promising cross combinations that can be advanced for later generation selection.
Key words: Drought stress, combining ability, water use efficiency.
Drought remains a challenge in the eastern and north eastern regions of Uganda where cowpea is predo-minantly grown. These areas are already grappling with high water stress, rapid population growth, environmental degradation, and low socio-economic growth (Bigirimana, 2011). Cultivation of plants that are adapted to water
stress conditions is necessary in order to reduce crop production losses and meet the need for food for the growing population (Fageria et al., 2007). Breeding for drought tolerance lessens the danger of crop failure by improving the crop’s ability to extract water from the soil, improving its water use efficiency and ability to survive longer periods without water. Breeding for drought tolerance, however, requires knowledge of the inheritance and genetic variability for traits conferring drought tolerance (Chiulele, 2010). This information is required for the identification of the best parents and selection strategies to use in breeding. In light of this, two approaches have been proposed for screening and breeding for drought tolerance in cowpea. These include: One that uses grain yield and its components, as the primary criteria and one that characterizes specific morpho-physiological traits that contribute to growth and yield in the event of drought (Agbicodo et al., 2009).
Cowpea exhibits genetic variability for drought tolerance (Muchero et al., 2008). Gene action studies conducted elsewhere have reported the predominance of additive genetic effects over non-additive genetic effects in controlling yield components such as days to flowering, number of seeds per pod, number of pods per plant and hundred seed weight (Chiulele, 2010; Romanus, 2008). Non-additive genetic effects were, however, more important for seed yield (Chiulele, 2010; Alidu et al., 2013). Thus, information regarding combining ability and nature of gene action governing the inheritance of desirable traits are basic requirements for breeding high yielding drought tolerant cowpea genotypes. However, such information is not available for cowpea genotypes in Uganda. Combining ability and heritability estimates are specific to germplasm being tested and the testing environment (Falconer, 1989). Knowledge of the genetic control of complex quantitative traits and the magnitude of genetic variability that exists among the available germplasm are important for selection and genetic improvement of the crop (Umar et al., 2014). Selection of parental genotypes based on combining ability estimates has been used as an important breeding approach in crop improvement (Umar et al., 2014). This study was, therefore, carried out in order to estimate the general and specific combining ability effects of parents and crosses as well as estimate the heritability of delayed leaf senescence, seed yield and its components. The information generated will help in planning and implementation of an efficient breeding program for improvement of drought tolerance levels of cowpea in Uganda.
Study area
The study was conducted in a water proof screen house at the Makerere University Agricultural Research Institute - Kabanyolo (MUARIK).
MUARIK is located at an altitude of 1217 m above sea level on coordinates 0.16° 24ʹ 16 N and 32.5° 27ʹ 34 E, approximately 19 km northeast of Kampala within the Lake Victoria Crescent. The average temperature and relative humidity in the screen house during the study ranged from 25 to 38°C and 70 to 92%, respectively.
Developing a breeding population
Four confirmed exotic drought tolerant lines from IITA, Kano (IT 98K-205-8, IT99K-573-1, IT 93K-452-1, IT 89KD-288) and five released Ugandan lines (SECOW 1T, SECOW 2W, SECOW 4W, SECOW 3B, SECOW 5T) with desirable disease resistance characteristics were crossed to generate a total of 20 F1 families. The nine parents were crossed in the screen house using a North Carolina II mating design (Acquaah, 2007). The NC II mating design is utilized in general and specific combining ability estimation and in determining the relative importance of additive over non- additive genetic effects (Acquaah, 2007).
Population advancement and drought tolerance screening
The F1 plants of each of the 20 F1 crosses were selfed to generate F2 seeds. However, one cross involving SECOW 2W with IT 99K-573-1 did not germinate possibly due to seed dormancy attributed to both the embryo and seed coat factors. The F2 seeds together with the nine parents and seven checks were planted in 10 L plastic pots and the plants were phenotypically characterized for drought tolerance for the following traits: Delayed leaf senescence, number of pods per plant, number of seeds per pod, 100 seed weight and seed yield. The genotypes were subjected to drought stress after the emergence of the flower bud on individual pot basis. The experiment was laid out in a randomized complete block design in a split plot arrangement with five replications and two watering regimes. The watering regime was the main plot and test genotypes, each represented by four plants per water regime, formed the sub plots. The watering regimes were: T1, as no stress (NS) and T2, as severe water stress (DS) treatments. Plants in the well-watered treatment (no stress) were maintained at field capacity (50% soil water capacity) throughout the experimental period by measuring the soil moisture content using an MO750 soil moisture meter twice a week and applying water to restore the appropriate moisture level. The severe water stress treatment did not receive water for 20 days, then it was re-watered.
Data collection
Data was collected on individual plant basis in line with the international plant genetic resources cowpea descriptors; days to 50% flowering and delayed leaf senescence. Plants were scored for leaf senescence on a scale of 1 to 5, with 1 = totally green and turgescent, 2 = green and slightly wilted, 3 = green yellow and wilt, 4 = yellow-green and severely wilt and 5 = completely yellow to brown / almost died (Muchero et al., 2008). At maturity, the plants were harvested to determine yield components: number of pods per plant, number of seeds per pod, 100 seed weight and seed weight per plant.
Data analyses
The data collected were analysed using Genstat software 12th edition of VSN International. The means of the 19 F2 family crosses, seven checks and nine parents were compared in an analysis of variance using the following linear model:The Linear Mathematical Model for split plot experimental design used was as follows:
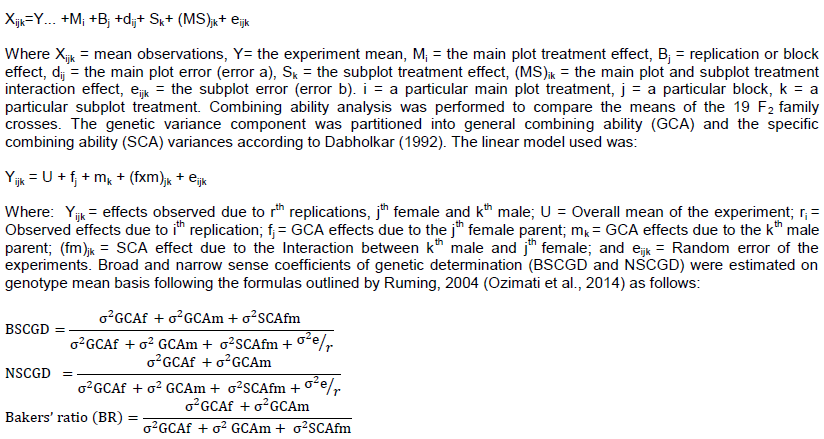
Where, δ2GCAf = variance component general combining ability for female parents, δ2GCAm = variance component general combining ability for male parents, δ2SCAfm = variance component specific combining ability for the hybrids, δ2e = variance component error, r = number of replications. The variance component for males were calculated by subtracting their mean squares from the error mean squares and dividing the males by the number of females involved in the crossing vice-versa (Dabholkar, 1992). To quantify drought severity, the drought intensity index (DII) was calculated using the formula suggested by Fischer and Maurer (1978) as follows: DII = 1 - Xs/Xp, where Xs and Xp are the mean grain yield of all the genotypes of the same maturity group under drought stress (DS) and non-stress(NS) conditions, respectively.
Response of F2 population and parental genotypes to drought stress
The analysis of variance for seed yield, number of pods per plant, number of seeds per pod and 100 seed weight for parents, checks and F2 population showed that the parents, checks and F2 population were significantly different (P ≤ 0.001). The watering regimes explained much of the variation that was seen in seed yield and its components as indicated by the high mean squares (Table 1). The mean performance of all the crosses in seed yield, number of seeds per pod, 100 seed weight was higher than the average performance of all the parents except for delayed leaf senescence (Table 2).
Combining ability analysis
Mean squares of crosses (Table 3) were significant under water stress for delayed leaf senescence (P ≤ 0.05), number of pods per plant (P ≤ 0.01), 100 seed weight (P ≤ 0.01) and seed yield (P ≤ 0.001). Further partitioning of variance of crosses into that due to male and female parents attributed to GCA, and that due to male and female interaction attributed to SCA showed that under water stress conditions, the mean squares for general combining ability (GCA) for male parents (Table 3) were significantly different for number of pods per plant, number of seeds per pod (P ≤ 0.01), 100 seed weight (P ≤ 0.01) and seed yield (P ≤ 0.001). The mean squares for GCA for female parents (Table 3) were only significantly different for delayed leaf senescence (P ≤ 0.05). The mean squares for the specific combining ability (SCA) of parental combinations were significantly different for number of pods per plant (P ≤ 0.01), 100 seed weight (P ≤ 0.05) and seed yield (P ≤ 0.001). The results also showed that in the absence of water stress, the mean squares for GCA for male parents were only significantly different (P ≤ 0.01) for 100 seed weight and seed yield (Table 3).
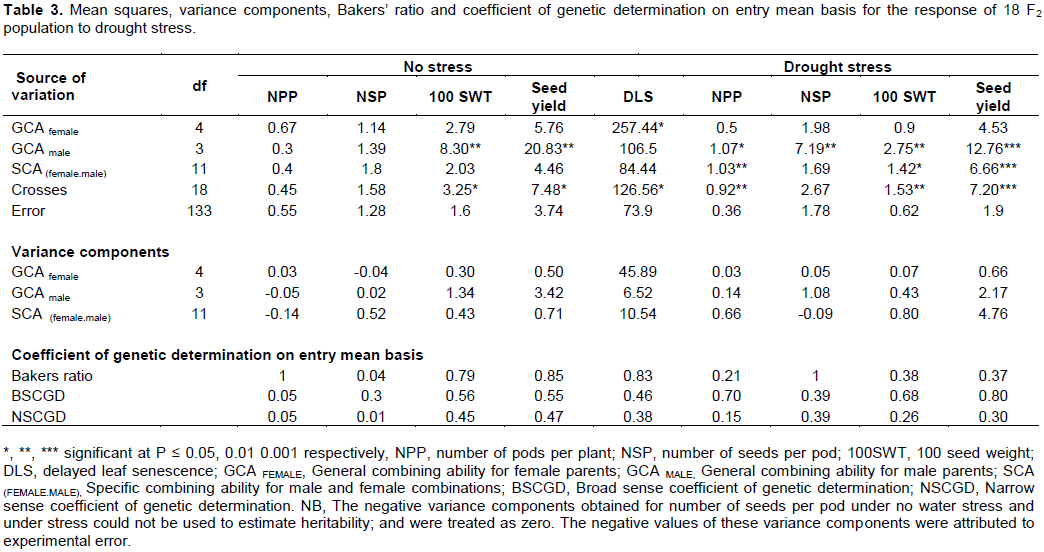
The estimates of narrow sense coefficient of genetic determination was very low (0.01-0.05) for number of pods per plant and number of seeds per pod. The estimates of broad sense coefficient of genetic determination were moderately high to high (0.68-0.8) for number of pods per plant, 100 seed weight and seed yield but moderate (0.39-0.46) for delayed leaf senescence and number of seeds per pod. The estimates of narrow sense coefficient of genetic determination was low to moderate (0.15-0.39) for all the traits.
Estimates of general and specific combining ability effects
Female parent SECOW 5T showed desirable significant positive GCA effect for seed yield (Table 4). SECOW 2W showed average positive GCA effects for number of pods per plant, 100 seed weight and average GCA effects for seed yield and number of seeds per pod. However, SECOW 2W showed undesirable significant positive GCA effect for delayed leaf senescence. SECOW 4W showed desirable significant positive GCA effect for number of seeds per pod and average effects for number of pods per plant, 100 seed weight, seed yield and a negative average GCA effect for delayed leaf senescence. SECOW 3B and SECOW 1T showed undesirable significant negative GCA effects for number of pods, 100 seed weight and seed yield. However, SECOW 1T showed the highest desirable significant negative GCA effect for delayed leaf senescence. The male parent IT 93K-452-1 and IT98K-205-8 showed desirable significant positive GCA effects for seed yield, number of pods per plant, number of seeds per pod, 100 seed weight and a desirable average GCA effect and significant negative GCA effect for delayed leaf senescence respectively (Table 4). Hence, they were good combiners. IT99K-573-1 showed undesirable significant negative GCA effects for seed yield, number of pods per plant, number of seeds per pod, 100 seed weight. IT99K-573-1 also showed a positive undesirable GCA effect on delayed leaf senescence, hence, it was a poor combiner.SECOW 5T x IT98K-205-8 and SECOW 1T x IT98K-205-8 showed desirable significant positive Specific combining ability effects for seed yield, number of pods per plant, number of seeds per pod, 100 seed weight and a desirable significant negative SCA effects for delayed leaf senescence (Table5). SECOW 4W x IT 98K-205-8 showed desirable positive SCA effects for yield and its components except for number of seeds per pod and a desirable significant negative SCA effects for delayed leaf senescence while SECOW 3B x IT98K-205-8 showed desirable SCA effects for yield and its components and an average negative SCA effect for delayed leaf senescence. The crosses SECOW 3B x IT 93K-452-1, SECOW 3B x IT99K-573-1 and SECOW 3B x IT89KD-288 showed desirable negative SCA effects for delayed leaf senescence. The cross SECOW 1T x IT93K-452-1 showed undesirable positive SCA effect on delayed leaf senescence (Stay green trait). SECOW 5T x IT93K-452-1 exhibited a significant non-desirable negative SCA effect for seed yield (Table 5).
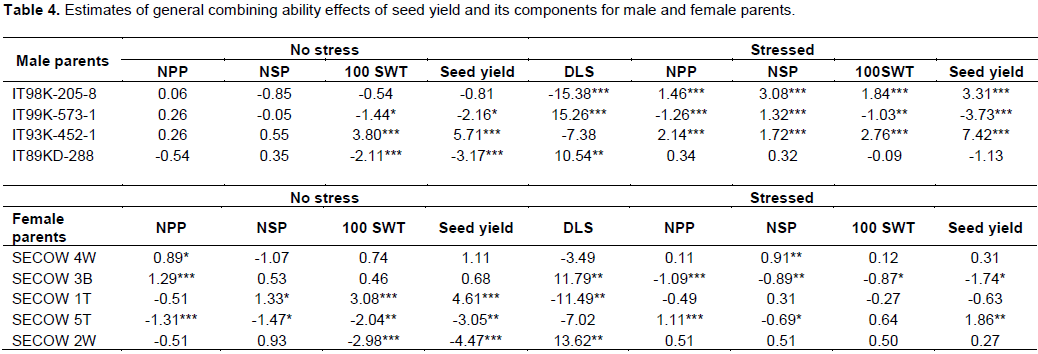

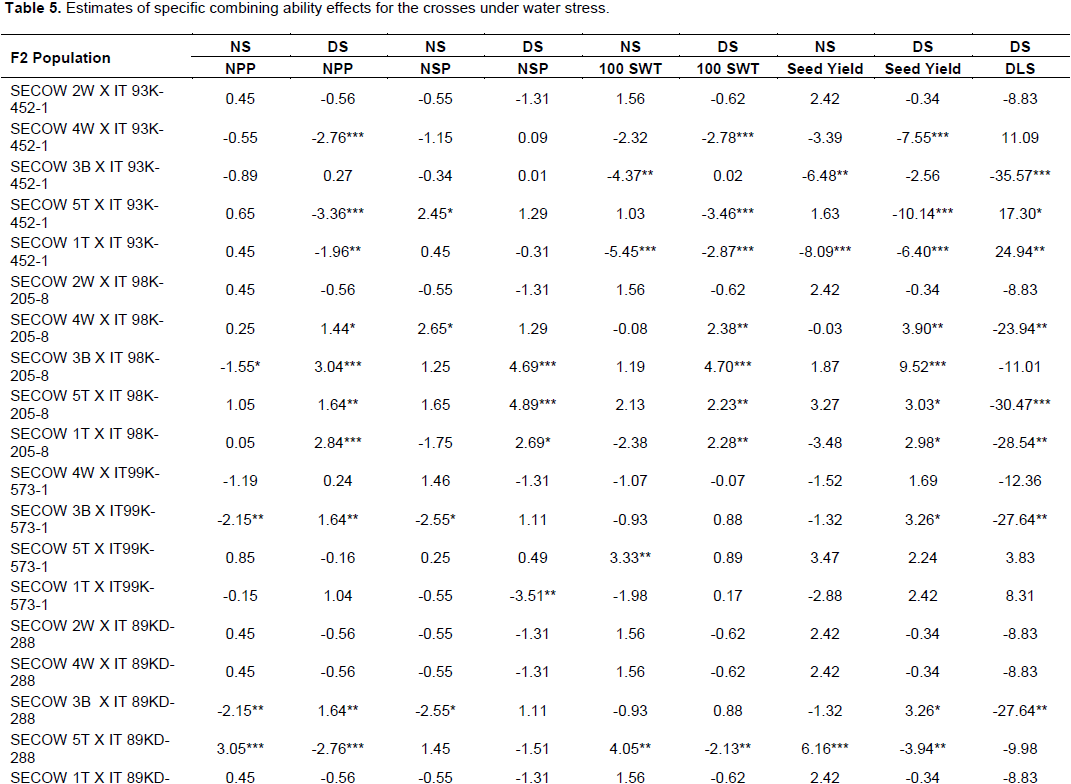

Genetic variation
The overall goal for all plant breeding programs is achieving yield gains and this is dependent on having genetic diversity for the trait under selection with a higher heritability (Falconer and Mackay, 1996). The analysis of variance showed that genotypes were significantly different in most traits evaluated under water deficit conditions, an indication of the existence of genetic variability among parents and their progenies for drought tolerance, favoring selection. The mean performance of most crosses in seed yield, number of seeds per pod, 100 seed weight was higher than most parental genotypes except for delayed leaf senescence, an indication of the presence of transgressive segregants (Table 2), which may have been as a result of recombination of additive alleles (complementary gene action) or interaction between two alleles of two different genes due to a wider genetic distance between genotypes of their parents (Marame et al., 2009). The presence of transgressive segregants suggests polygenic inheritance and breeding strategies such as backcrossing, multiple crossing,heterosis and pedigree breeding methods with recurrent selection could facilitate the simultaneous exploitation of these favorable alleles.
The average seed yield, number of pods per plant, number of seeds per pod and 100 seed weight was higher under no moisture stress than under moisture stress conditions for both parents and progenies (Table 2), indicating that moisture stress significantly reduced seed yield and its components of the parents and progenies. The intensity of the drought stress as shown by the drought Intensity Index value of 0.56 was sufficient to reduce the yield and its components of the cowpea genotypes. DII values above 0.7 are considered as severe drought stress (Ramirez-Vallejo and Kelly, 1998). Shirinzadeh et al. (2010) selected Maize and dry bean cultivars as sources of drought tolerance in the field with DII values ranging between 0.51 and 0.69, so the drought stress intensity in this study was sufficient to separate drought tolerant cultivars from drought sensitive cultivars.
Heritability and combining ability
General combining ability (gca) refers to the average performance of a parent in hybrid combinations and specific combining ability (sca) is the performance of a parent relatively better or worse than expected on the basis of the average performance of the other parents involved (Griffings, 1956). The significant mean squares of GCA male and specific combining ability (SCA) for number of pods per plant, 100 seed weight and seed yield, indicated that both additive and non-additive genetic factors were important in the genetic control of these traits. However, the additive gene effects were relatively more important than the non-additive for the traits number of seeds per pod and delayed leaf senescence as the estimates of Baker’s ratio and narrow sense coefficient of genetic determination were high. This implies that in this set of crosses, there would be a fairly high predictability of progeny performance from the parents’ GCA effects for these traits. Besides, since additive genetic variance is the variance of breeding values and the main determinant of response to selection (Falconer, 1996), these traits will be easy to improve by simple selection methods such as mass selection and pedigree method. Similar findings were reported by Chiulele (2010).The non-additive geneaction due to dominance and/or epistasis, as shown by significant SCA mean squares contributed to the total genetic variation observed in number of pods per plant, 100 seed weight and grain yield. Gupta et al. (1993) reported that the presence of non-additive genetic effects lowers progress expected from early generation selection. The Baker’s ratio was moderate for grain yield and 100 seed weight, low for number of pods per plant indicating the predominance of non-additive genetic control in the genetics of these traits. Variation observed among plants is due to the combined action of genetic and environmental factors (Baker, 1978). Heritability is a measure of the proportion of variance observed among plants that is due to genetic differences and is expressed in a broad or narrow sense way. The broad sense heritability is responsible for providing the proportion of genetic variance present in the phenotypic or total variance (Lobato et al., 2014). The broad sense coefficient of genetic determination was high for grain yield, 100 seed weight and number of pods per plant. Higher Broad sense coefficient of genetic determination estimates may be caused by greater additive genetic variance, lower environmental variance or minor inter-actions between genotype and environment (Acquaah, 2007). Similar results on high broad sense heritability estimates for grain yield.
100 seed weight and number of pods per plant were reported by Lobato et al. (2014). Though, broad-sense coefficient of genetic determination estimates were very high (> 0.5) for grain yield, number of pods per plant and 100 seed weight traits, narrow-sense coefficient of determination values were moderate to low (< 40%). Since narrow-sense coefficient of genetic determination estimates shows the proportion of a trait that is transmitted from parents to their progenies, the low narrow sense coefficient of genetic determination estimates for these traits signifies the presence of dominance effect (Abney et al., 2001) and suggests a low gene transmission to the progenies. Hence, a lower response to selection is expected in early generations as opposed to later advanced generations. Improvement for these traits therefore requires a recurrent selection procedure that allows for favorable gene recombination in later generations before a final selection is made. Similar results on moderate to low narrow sense heritability estimates for grain yield, 100 seed weight and number of pods per plant were reported by Alidu et al. (2013). However, these results contradicted with Chiulele (2010) findings, possibly due to the differences in the population studied, multilocationality of the study as Chiulele’s study was conducted across locations while this study was done in one location.
Combining ability effects
The GCA estimates provide information on the concentration of predominantly additive gene effects and are useful in identifying parents to be used in breeding programs (Cruz et al., 2004). Dabholkar (1992) reported that parents with significant GCA effects in the desired direction for a character of interest are the best for hybridization. Female parent SECOW 5T showed positive significant GCA effects for seed yield and its components, negative significant GCA effects for delayed leaf senescence in a desirable direction under water stress suggesting that this cultivar would contribute to increasing seed yield, number of pods per plant, number of seeds per pod and 100 seed weight under moisture stress conditions. As such SECOW 5T can be used in a breeding program as a source of drought tolerance. Similarly, male parents IT93K-452-1 and IT 98K-205-8 showed desirable significant positive GCA effects for seed yield and its components and negative significant GCA effects for delayed leaf senescence. These male parents can be utilized in breeding for drought tolerance as good parents. Crossing between two good general combiners governed by additive x additive gene actions may elicit transgressive segregants in the advanced generations for the traits, thereby producing hybrids with good specific combining ability (Daniel et al., 2006).
Cruz et al. (2004) reported that selection for favorable estimates of SCA should prioritize cross combinations that involve at least one parent which has shown favorable effect of GCA. The F2 crosses SECOW 5T X IT 98K -205-8, SECOW IT X IT 98K-205-8 and SECOW 3B X IT 98K-205-8 showed desirable positive significant SCA effects for seed yield and its components, implying that these crosses performed higher than what was predicted based on their parents’ GCA effects. The average seed yield of SECOW 3B x IT98K-205-8, SECOW 5T x IT98K-205-8 and SECOW 4W x IT98K-205-8 under moisture stress conditions was above the mean seed yield of all the crosses. The dominance of these crosses may be due to complementary and duplicate gene actions (Falconer, 1989). As such these crosses are expected to produce desirable segregants and could be exploited in cowpea varietal improvement programs. In effect, a large and positive SCA effects for a trait suggests the possibility for transgressive segregation for the trait in later generation of selfing (Ojo, 2003).
This study elucidated the genetic control of drought adaptation traits in selected cowpea parental genotypes. Both additive and non-additive genetic effects were responsible for the inheritance of drought adaptation traits. However, non-additive genetic effects were more important than additive genetic effects implying that the performance of progenies was better in specific crossing combinations and could not be predicted for a wide range of crosses. Therefore, improvement of drought adaptation traits through selection of crosses with high positive SCA effects and advancing them to later generation would be effective. The good combiners for seed yield, number of pods per plant, number of seeds per pod, 100 seed weight and delayed leaf senescence were SECOW 5T, IT 93K-452-1 and IT 98K-205-8. These parents are, therefore, recommended as sources of drought tolerance for breeding programs. The F2 families of the crosses: SECOW 3B x IT98K-205-8, SECOW 5T x IT98K-205-8, SECOW 4W x IT98K-205-8 and SECOW 1T x IT98K-205-8 were promising combinations that showed desirable positive significant SCA effects for seed yield, 100 seed weight and number of pods per plant. These crosses should be advanced for selection in later generations.
The authors have not declared any conflict of interests.
REFERENCES
Abney M, Mcpeek MS, Ober C (2001). Broad and narrow heritabilities of quantitative traits in a founder population. Am. J. Human Genet. 68:1302-1307.
Crossref
|
|
Acquaah G (2007). Principles of plant genetics and breeding. Blackwell, Oxford.
|
|
|
Agbicodo EM, Fatokun CA, Muranaka S, Visser RGF, Linden van der CG (2009). Breeding drought tolerant cowpea: constraints, accomplishments, and future prospects. Euphytica 167(3):353-370.
Crossref
|
|
|
Alidu M, Atokple I, Akromah R (2013). Genetic Analysis of Vegetative Stage Drought Tolerance in Cowpea. Gen. J. Agri. Sci. 3(6):481-496.
|
|
|
Baker RJ (1978). Issues in diallel analysis. Crop Sci. 18:533-536
Crossref
|
|
|
Bigirimana P (2011). Uganda: The 2010-2011 Integrated Rainfall Variability Impacts,Needs assessment & drought risk management strategy. Office of the Prime Minister.
|
|
|
Chiulele RM (2010). Breeding Cowpea for Improved Drought Tolerance in Mozambique. PhD Thesis, University of Kwazulu Natal.
|
|
|
Cruz CD, Regazzi AD, Carneiro PCS (2004). Modelos biométricos aplicados ao melhoramento genético. Viçosa: Universidade Federal de Viçosa. P 480.
|
|
|
Dabholkar AR (1992). Elements of Biometrical Genetics. College of Agriculture (1st ed), Idore 452001 (M.P), Ashot Kumat Mittal, Publishing Company, New Delhi 110059:74-76.
|
|
|
Daniel IO, Oloyede HT, Adeniji OT, Ojo DK, Adegbite AE (2006). Genetic analysis of earliness and yield in elite parental lines and hybrids of tropical maize (Zea mays L.). J. Genet. Breed. 60:289-96.
|
|
|
Fageria NK, Baligar VC, Clark RB (2007). Physiology of Crop Production. International book distributing company. India
|
|
|
Falconer DS, Mackay TFC (1996). Introduction to quantitative genetics (4th ed). Pearson Prentice Hall, Harlow, England.
|
|
|
Falconer DS (1989). Introduction to quantitative genetics(3rd ed). Longman, New York.
|
|
|
Fischer RA, Maurer R (1978). Drought resistance in spring wheat cultivars. Grain yield responses. Austr. J. Agric. Res. 29:897-912.
Crossref
|
|
|
Griffings B (1956). Concept of general and specific combining ability in relation to diallel crossing systems. Austr. J. Biol. Sci. 9:463-493.
Crossref
|
|
|
Gupta VP, Kapila RK, Sood VK, Rana ND (1993). Predicting transgression on the basis of combining ability and heterosis in soybean. Crop Improv. J. 20:143-150.
|
|
|
Lobato AKS, Vidigal MCG, Filho PSV, Ramos VMS, Poletine JP, Andrade CAB (2014). Genetic parameters of grain production and its components in common bean under drought stress. Aust. J. Crop Sci. 8(8):1152-1159.
|
|
|
Marame F, Desalegne L, Fininsa C, Sigvald R (2009). Genetic analysis for some plant and fruit traits, and its implication for a breeding program of hot pepper. Hereditas 146:131-140.
Crossref
|
|
|
Muchero W, Ehlers JD, Roberts PA (2008). Seedling Stage Drought-Induced Phenotypes and Drought-Responsive Genes in Diverse Cowpea Genotypes. Crop Sci. 48(2): 541.
Crossref
|
|
|
Ojo DK (2003). Heritability and combining ability of seedling emergence, grain yield and related characteristics in six tropical Soyabean (Glycine max (L.) Merr.) cultivars. Niger. J. Genet.18:22-28.
|
|
|
Ozimati A, Rubaihayo PR, Gibson P, Edema R, Kayondo IS, Ntare BR, Okello DK (2014). Inheritance of resistance to kernel infection by Aspergillusflavus and aflatoxin accumulation in groundnut. Afr. Crop Sci. J. 2(1):51-59.
|
|
|
Ramirez-Vallejo P, Kelly JD (1988). Traits related to drought resistance in common bean. Euphytica 99:127-136.
Crossref
|
|
|
Romanus KG, Hussein S, Mashela WP (2008). Combining ability analysis and association of yield and yield components among selected cowpea lines. Euphytica 162:205-210.
Crossref
|
|
|
Shirinzadeh AR, Zarghami AV, Azghandi M, Shiri, Mirabdulbaghi (2010). Evaluation of drought tolerance in mid- and late mature corn hybrids using stress tolerance indices. Asian. J. Plant Sci. 9:67-73.
Crossref
|
|
|
Umar UU, Ado SG, Aba DA, Bugaje SM (2014). Estimates of combining ability and gene action in Maize under water stress and non- stress conditions. J. Biol. Agric. Health care 4(25):247-253.
|
|