ABSTRACT
The high concentrations of salts in water, especially in semiarid regions, increase salt concentration in the soil and plant with the consequent decrease in NPK contents. Current study evaluates the influence of irrigation with water of different salinities, associated to nitrogen (N) fertilization, on NPK contents in castor bean cv. BRS Energia. An experiment was conducted for four months, in lysimeters under the field condition, in the municipality of Pombal, Paraíba - Brazil, using an Eutrophic Entisol of sandy loam texture ina randomized 5 × 4 factorial block design with three replications. Five levels of electrical conductivity of irrigation water (ECw=0.3, 1.2, 2.1, 3.0 and 3.9 dS m-1) and four doses of N (70, 100, 130 and 160 mg kg-1) were tested. The highest contents of potassium (K) in roots and leaves were obtained respectively with ECw of 0.3 and 2.2 dS m-1. The N content in leaves was not influenced by factors under analysis. Increase in N dose decreased the plant contents of phosphorus (P) and K.
Key words: Ricinus communis L., electrical conductivity, regressions.
The castor bean plant (Ricinus communis L.) of the family Euphorbiaceaeis an oleaginous plant with high socioeconomic value, featuring products and by products used in the castor oil chemical industries and in agriculture, with a possible use as a biofuel. Oil from its seeds has approximately 90% ricinoleic fatty acid with special characteristics and energy contents. It is used in industries as an ingredient in the manufacture of plastics, synthetic fibers, paints, enamels, lubricants and others (Almeida Júnior et al., 2009).
Castor bean seeds cv. BRS Energia were used as planting material. According to Silva et al. (2009), the cultivar is a vigorous genetic material, easily propagated, with a cycle of 130 days, low height (average 106 cm), with semi-indehiscent fruits, oil content in seeds averaging 48% and an average productivity of 1.800 kg ha-1.
The use of castor oil for the production of biodiesel is a hallmark in economic, environmental and social fields by increase in jobs and profits with the strengthening of agricultural economy, especially family agriculture, and the generation of opportunities due to an increase in agricultural areas in the semiarid northeastern regions in Brazil (Azevedo and Beltrão, 2007). However, precisely in this region the rainfallis very irregular and irrigation is the best method to guarantee agricultural production. Due to demands for a good water quality and the growing needs for the expansion of agricultural production worldwide, farmers have been compelled to use water with moderate to high salt contents in irrigation (Nobreet al., 2011).
High concentrations of salts in the soil may not only reduce the soil´s water potential but also may cause toxic effects in plants, such as functional disorders and damages in their metabolism (Debouba et al., 2006). The above-mentioned effects depend on many other factors such as plant species, cultivar, phenologic stage of crop, type of salts, intensity and duration of saline stress, crop management and irrigation, and edaphoclimatic conditions (Tester and Davenport, 2003). The osmotic adjustment is highly important for the development of plants in a saline medium and any flaw in this equilibrium causes damages very similar to those by drought, such as loss of turgidity and decrease in growth and production (Ashraf and Harris, 2004).
The importance of nutrition supplementation with nitrogen (N) in plants under saline stress should also be taken into account. In fact, the macronutrient N has relevant physiological functions on the formation of organic compounds, with special reference to amino-acids, proteins, coenzymes, nucleic acids, vitamins, chlorophyll and others (Chaves et al., 2011). Further, Silva et al. (2008) report that N fertilization enhances plant growth and decreases the deleterious effects of high salt concentration on the growth and production of crops. In fact, the accumulation of these solutes (glycine betaine, N-amino solutes, proline; lysine, glutamine, aspartic acid), increases the capacity of osmotic adjustments of plants to salinity and tolerance of crop to water and saline stress.
Current research assesses the effects of irrigation with water containing different salinity levels and N doses on N, P and K contents in the castor bean plant cv. BRS Energia.
The experiment was conducted under the field condition in an experimental area of Agricultural Sciences and Food Technology Center of the Federal University of Campina Grande (CCTA/UFCG) in Pombal, Paraíba - Brazil, at 6°48’16” S and 37°49’15” W, average altitude 144m, during September 2011 and January 2012 using plasticcontainers as drainage lysimeters. Five levels of electrical conductivity of irrigation water (ECw = 0.3; 1.2; 2.1; 3.0 and 3.9 dS m-1) were used combined with four N rates (70; 100; 130 and 160 mg N kg-1 of soil). These doses were based on the recommendations of Novaiset al. (1991) for assays in pots (100 mg kg-1). Experimental design consisted of randomized blocks (5x4), with three replications. The experimental units were distributed in simple rows spaced 0.90 m apart, with 0.70 m between plants in the row.
The seeds were sown in the 100 L plastic containers. After thinning, one plant per pot was kept and formed the experimental unit. Each recipient had two holes in its lower segment for drainage of excess water and plastic bottles for the collection of drained water were placed beneath the holes to estimate water consumption per plant. Recepients were filled with 2 kg of pebbles number zero which covered the bottom of the recipient. Further, 107.5 kg of Eutrophic Entisol of sandy loam texture, non-saline and non-sodic, collected at a depth between 0 - 30 cm, were placed on the pebbles. Soil, retrieved from the municipality of Pombal, PB - Brazil, was ground and sieved (<0.02 mm)and its physical and chemical attributes (Table 1) were analysed by methodology described by Claessen (1997).

Fertilization was performed for potassium and phosphorus based on the recommendations of Novais et al. (1991), using 12.0 g of potassium chloride and 162.5 g of monoammonium phosphate. In addition 2.5 kg of vermicompost (6.3 g N kg-1; 1.28 g P kg-1; 0.53 g K kg-1) was also applied to increase fertility and water retention capacity of soil.
Nitrogen was applied in split doses, one third was applied at the start of the experiment and the other two thirds in four equal applications along with irrigation water at 10-day intervals starting 25 days after sowing (DAS). In treatment N2 (equivalent to 100% recommendation), 33.34 g mono-ammonium phosphate (MAP) and 8.88 g urea were applied. Two foliar applications with Albatroz (N - 10%; P2O5 - 52%; K2O - 10%; Ca - 0.1%; Zn - 0.02%; B - 0.02%; Fe - 0.15%; Mn - 0.1%, Cu - 0.02%; Mo - 0.005%) in the proportion of 1 g L-1of water, at the start of flower emission (29 and 37 DAS), were also undertaken.
Considering the contents of N, P and K found in the vermicompost and the albatroz solution treatments received additional amounts of 6390, 21900 and 1070 mg kg-1 of N, P2O5and K2O, respectively, however, these additional amounts on top of recommended dosages of Novais et al. (1991) for nitrogen, phosphorus and potassium were not considered for analyzing the effect of N since all treatments had received the same amount of vermicompost and albatroz solution and the same being negligible compared to pre-established doses of N.
Different salinity levels were obtained by addition of NaCl in water from the local supply system and quantity was determined by employing relation C (mg L-1) = 640 × ECw (dS m-1), (Rhoades et al., 2000). After soil conditioning in the recipient, water content was raised to field capacity by capillary saturation, followed by free drainage with the respective type of water. After preparation and ECw calibration, using a portable conductivity meter, the waters were stored in plastic recipients, adequately protected in order to avoid evaporation. The subsequent irrigations occurred daily at 17.00 h and volume of water to be applied was calculated according to irrigation requirements determined by water balance, or rather, volume applied minus volume drained in the previous irrigation.
The contents of N, P and K in leaf, stem and root were assessed after 120 DAS. The plants were harvested and their respective parts separated and dried in a forced air oven at 65°C till constant weight. The plant samples were then weighed, milled and analyzed, following methodologies recommended by Silva (1999). Data were evaluated by analysis of variance with F-test at 0.05 probability using SISVAR-ESAL (Ferreira, 2003) and regression analysis were performed when effects were significant. Choice of model of regression was based on value of coefficient of determination (R2) taking into consideration a plausible biological explanation.
Analysis of variance (Table 2) showed that levels of water salinity (ECw) had a significant effect on N and P contents in stem and root tissues, whereas K contents of all organs of the castor bean plants under analysis were significantly influenced by this treatment. For N rates, there was a significant effect on N accumulation in stem and root tissues. Nitrogen rates also affected (p<0.05) P and K contents in leaf and stem tissues. The interaction between ECw versus N rates was significant for concentrations of N in the roots and P and K in the stem tissues, respectively.
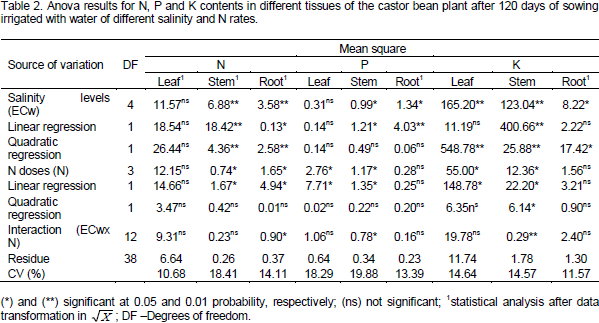
The foliar N content showed a mean value of 11.98 g kg-1 which was not affected by salinity in irrigation water, but there was a 67.71% increase in N content of stem with per unit increase (p<0.01) of ECw (Figure 1a). Results for N content in the stem corroborate with that of Al-Harbi (1995) who reported the non-dependence between N contents and salinity levels for the period in which the plant underwent stress and especially for the species and genotypes with different salt tolerance.
The N content in the roots showed a quadratic response (p < 0.05) for plants with doses of 70 and 100 mg kg-1 N (Figure 1b). However, there was no significant effect for doses of 130 and 160 mg Nkg-1, respectively, which showed average contents of 2.45 and 2.66 g Nkg-1. Decrease in stem and root N contents in plants submitted to NaCl stress may have been caused by the competition between chloride (Cl-) and nitrate (NO3-) ions transporters (Ogawa et al., 2000) and/or the inactivation of NO3- transporters due to the toxic effects of the ions (Lin et al., 2002). Salinity also causes the rupture of the root membrane and inhibits NO3-absorption (Parida and Das, 2004).
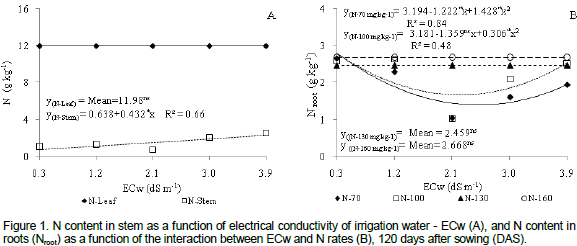
Further, saline stress interferes in enzymes similar to ammonium (NH4+), with a greater accumulation of the ion in the tissue, besides the accumulation from the degradation of proteins caused by the stress (Zhou et al., 2004). Costa et al. (2008) also reported that increase of salt concentration of the irrigation water (0.15 to 4.5 dS m-1) caused deleterious effect on N concentration in root tissues of Amaranthus spp.
Phosphorus content in leaf tissues was negatively affected by N doses (Figure 2a). There was a 5.33% decrease in P for each interval of 30% in N doses. When plants were submitted to the maximum N dose (160 mg kg-1), P content in leaves decreased by 0.9 g kg-1 (16%) when compared to those with a lower dose (70 mg kg-1). According to Malavolta et al. (1997), P is a highly mobile element in plants and reaches the leaves or the meristhematic locations by long distance transport and together with N is immediately distributed in the above ground plant material. With the aging of leaves, up to 60% of P may also be transported by the phloem to other segments of the plant, especially to new organs and developing fruit, with a decrease in P content of the leaves.
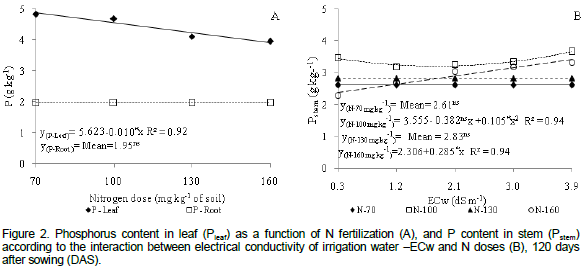
There was a quadratic response of plants with 100% N dose with regard to P contents in stem tissues (Figure 2b). High accumulation of P (3.65 g kg-1) was reported under water salinity of 3.9 dS m-1. However, N dose of 160 mg kg-1 caused a linear increase in the concentration of P in the stem, with a 12.35% increase with per unit increase in ECw. There was a 44.49% (1.02 g kg-1) increase in P amounts in plants submitted to ECw=3.9 dS m-1 when compared to plants irrigated with lower salinity level (0.3 dS m-1). It may be observed (Figure 2b) that the application of doses of 70 and 130 mg kg-1 N did not affect P content in the stem tissues of the castor bean plant but registered mean concentrations of 2.61 and 2.81 g kg-1 P, respectively.
Several studies demonstrated that the interaction between water salinity and P uptake in plants is a highly complex issue and depends on plant species, salt concentration, type of salt and salinity level of irrigation water (Grattan and Grieve, 1999). However, the accumulation of P in the stem of plants under salt stress may be a consequence of the decrease of translocation associated with a possible reduction in growth rate (Lacerdaet al., 2006). Results corroborate those observed in gliricidia (Farias et al., 2009) and cowpea (Silva et al., 2011) plants cultivated under saline stress.
Plants irrigated with ECw=2.2 dS m-1 caused a high accumulation of K contents in leaves (27.03 g kg-1) with a decrease in ion concentration above this ECw level (Figure 3a). The low uptake of K in plants under high salinity levels has been attributed to the competition between Na+ and K+ by the absorption sites of the plasmalema and to the great efflux of K+ from the roots as a direct result of osmotically induced exchanges in the permeability of the plasmalema and the substitution of Ca2+ by Na+ in the membrane (Ferreira et al., 2001).
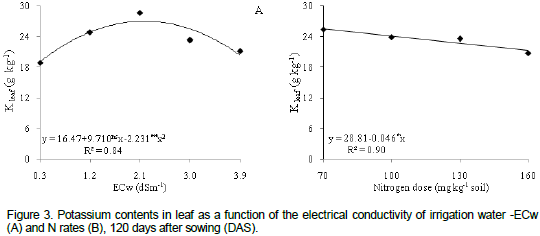
The first explanation is mainly due to the fact that the two cations (Na+ and K+) have a similar atomic radius and K+ transporters are less specific in toxic concentrations of Na (Castillo et al., 2007). Results in current research confirmed results obtained by Sousa et al. (2012) when they evaluated the influence of ECw in macro-and micronutrient contents in leaves of Jatropha curcas. Authors reported a quadratic effect and a highest K content (32.63 g kg-1) in plants under ECw=2.2 dS m-1.
With regard to the effect of N dose on the K content of leaves (Figure 4b), there was a decrease of 4.79% in the concentration of K+ with each 30% increase in the N dose, which amounts to a decrease of 14.37% of K when data of plants cultivated under the highest (160 mg kg-1) and lowest N dose (70 mg kg-1) were compared. According to Rosolem (2005), K is a strong competitor with other cations owing to the high efficiency of the plants´ absorption system. Absorption of the other cationic ions increases when K+ is absent in the solution since competition is less. The divergences in results in current study and those observed by Pacheco et al. (2008) may be explained considering that the latter studied the effects of NPK fertilization on castor bean IAC 226 and reported high contents of K+ when combined to high N doses.
The interaction between salinity and N dose interfered in the accumulation of Kin the stem tissues of the castor bean plant (Figure 4a), showing a quadratic response for all N doses. Maximum K+ contents (13.57, 11.41, 11.87 and 11.21 g kg-1) occurred when the plants underwent irrigation with ECw of 3.9, 3.3, 3.9 and 3.1 dS m-1, respectively. The lowest content of the nutrient (4.84, 6.13, 5.50 and 4.10 g Kkg-1) occurred in plants irrigated with water at ECw=0.3 dS m-1for N rates of 70, 100, 130 and160 mg kg-1, respectively. Selectivity increase of the K membrane is relevant to reduce the effect of salinity in plants since it is the main ion in osmotic adjustment and in the maintenance of cell turgor (Munns, 2002).

Potassium content was also significantly affected by the interaction of water salinity of irrigation water and N doses (Table 2). Figure 4b shows that plants with 70 and 100 mg kg-1 of N decreased quadratically, with maximum contents of K (6.89 and 7.36 g kg-1 respectively), obtained in plants irrigated with ECw of 0.3 dS m-1. There was a growing linear response with dose 160mg kg-1N which caused a 10.3% increase in K content with per unit increase in ECw (Figure 4b) or K content in plants irrigated with ECw=3.9 dS m-1 increased by 1.40 g kg-1 (37.10%) when compared to plants under ECw=0.3 dS m-1. There were no significant statistical effects for dose of130 mg kg-1 N, averaging 4.89 g kg-1 K in the roots (Figure 4b).
Decrease in K content in the root is possibly due to the direct exposure of roots to saline solution which causes changes in the integrity and selective permeability of the plasma membrane (Grattan and Grieve, 1999). Viana (2007) analyzed the combination of N and K doses in wheat, in a controlled area, and reported that K concentration in the dry matter of the roots decreased as N supply increased.
Highest potassium content in root and leaf tissues are obtained under irrigation water salinity of 0.3 and 2.2 dS m-1, respectively. The N content in leaves of castor bean is not affected by levels of salinity in irrigation water and N doses tested. The application of nitrogen doses causes decrease in leaf P and K contents.
The authors have not declared any conflict of interest.
REFERENCES
Al-Harbi AR (1995). Growth and nutrient composition of tomato and cucumber seedlings as affected by sodium chloride salinity and supplemental calcium. J. Plant Nutrit. 18(7):1403-1416.
Crossref |
|
Almeida JAB, Oliveira FA, Medeiros JF, Oliveira MKT, Linhares PCF (2009). Efeito de doses de fósforo no desenvolvimento inicial da mamoneira. Rev. Caatinga. 22(1):217-221. |
|
Ashraf M, Harris PJC (2004).Potential biochemical indicators of salinity tolerance in plants. Plant Sci. 166(1):3-16.
Crossref |
|
Azevedo DMP, Beltrão NEM (2007). O agronegócio da mamona no Brasil. 2. ed. Campina Grande, EmbrapaInformaçãoTecnológica. P. 504. |
|
Castillo EG, Tuong TP, Ismail AM, Inubushi K (2007). Response to salinity in rice: Comparative effects of osmotic and ionic stresses. Plant Prod. Sci. 10(2):159-170.
Crossref |
|
Chaves LHG, Gheyi HR, Ribeiro S (2011). Consumo de água e eficiência do uso para cultivar de mamona Paraguaçu submetida à fertilização nitrogenada. Eng. Ambient. 8(1):126‑133. |
|
Claessen MEC (1997). Manual de métodos de análise de solo. 2. ed. rev. atual. Rio de Janeiro: Embrapa-CNPS, P. 212. (Embrapa-CNPS. Documentos, 1). |
|
Costa DMA, Melo HNS, Ferreira SR, Dantas JA (2008). Conteúdo de N, P, K+, Ca2+ e Mg2+ no amaranto (Amaranthusspp) sob estresse salino e cobertura morta. Rev. Ciênc. Agron. 39(2):209-216. |
|
Debouba M, Gouia H, Suzuki A,Ghorbel MH (2006). NaCl stress effects on enzymes involved in nitrogen assimilation pathway in tomato Lycopersicon esculentum seedlings. J. Plant Physiol. 163(12):1247-1258.
Crossref |
|
Farias SGG, Santos DR, Freire ALO, Silva RB (2009). Estresse salino no crescimento inicial e nutrição mineral de gliricídia (Gliricídiasepium (Jacq.) KunthexSteud) em solução nutritiva. Rev. Bras. de Ciênc do Solo. 33(5):1499-1505.
Crossref |
|
Ferreira DF (2003). SISVAR 4,6 - programa de análise estatística.Lavras: Universidade Federal de Lavras, CD-ROM. |
|
Ferreira RG, Távora FJAF, Ferreyra HFF (2001). Distribuição da matéria seca e composição química das raízes, caule e folhas de goiabeira submetida a estresse salino. Pesq. Agropec. Bras. 36(1):79-88.
Crossref |
|
Grattan SR, Grieve CM (1999). Salinity-mineral nutrient relations in horticultural crops. Sci. Hortic. 78(1-4):127-157. |
|
Lacerda CF, Assis Júnior JO, Lemos Filho LCA, Oliveira TS de, Guimarães FVA, Gomes-Filho E, Prisco TJ, Bezerra MA (2006). Morpho-physiological responses of cowpea leaves to salt stress. Braz. J. Plant Physiol. 18(4):455-465.
Crossref |
|
|
|
Lin CC, Hsu YT, Kao CH (2002). The effect of NaCl on proline accumulation in rice leaves. J. Plant Growth Regul. 36(3):275-285.
Crossref |
|
Malavolta E, Vitti GC, Oliveira SA (1997). Avaliação do estado nutricional das plantas: Princípios e aplicações. Piracicaba: Potafos. P. 319. |
|
Munns R (2002). Comparative physiology of salt and water stress. Plant Cell Environ. 25(2):239-250.
Crossref |
|
Nobre RG, Gheyi HR, Soares FAL, Cardoso JAF (2011). Produção de girassol sob estresse salino e adubação nitrogenada. Rev. Bras. de Ciênc. do Solo. 35(3):929-937.
Crossref |
|
Novais RF, Neves JCL, Barros NF (1991). Ensaio em ambiente controlado. In: Oliveira AJ. Métodos de pesquisa em fertilidade do solo. Brasília: Embrapa-SBEA. pp. 189-253. |
|
Ogawa K, Soutome R, Hiroyama K, Hagio T, Shoji I, Nakagawa H, Komamine A (2000). Co-regulation of nitrate reductase and nitrite reductase in cultured spinach cells. J. Plant Physiol. 157(3):299-306.
Crossref |
|
Pacheco DD, Gonçalves NP, Saturnino HM, Antunes PD (2008). Teores foliares de nutrientes em mamoneiras (Ricinuscommunis L.) adubadas com doses variadas de NPK em solo de chapada da bacia do Rio Jequitinhonha. Rev. de Biol. e Ciênc. da Terra. 8(1):224-231. |
|
Parida AK, Das AB (2004). Effects of NaCI stress on nitrogen and phosphorous metabolism in a true mangrove Bruguiera parviflora grown under hydroponic culture. J. Plant Physiol. 161(8):921-928.
Crossref |
|
Rhoades JD, Kandiah AM, Marshali AM (2000).Uso de águas salinas para produção agrícola. Campina Grande: UFPB, P. 117. (Estudos da FAO – Irrigação e Drenagem, 48). |
|
Rosolem CA (2005). Interação de potássio com outros íons. In: Yamada T, Roberts TL. Potássio na agricultura brasileira. Piracicaba: Instituto da Potassa e Fosfato, pp. 239-256. |
|
Silva EC, Nogueira RJMC, Araujo FP, Melo NF, Azevedo Neto AD (2008). Physiological responses to salt stress in young umbu plants. Environ. Exper. Bot. 63(1-3):147-157.
Crossref |
|
Silva FC (1999). Manual de análises químicas de solos, plantas e fertilizantes. Brasília: Embrapa Comunicaçãoparatransferência de |
|
Silva FLB da, Lacerda CF de, Sousa GG, Neves ALR, Silva GL, Sousa CHC (2011). Interação entre salinidade e biofertilizante bovino na cultura do feijão-de-corda. Rev. Bras. Eng. Agríc. Ambient. 15(4):383:389.
Crossref |
|
Silva SMS, Gheyi HR, Beltrão NEM, Santos JW dos, Soares FAL (2009). Dotações hídricas em densidades de plantas na cultura da mamoneira cv. BRS Energia. Rev. Bras. de Ciênc. Agric. 4(3):338-348.
Crossref |
|
Sousa AEC, Lacerda CF de, Gheyi HR, Soares FAL, Uyeda CA (2012). Teores de nutrientes foliares e respostas fisiológicas em pinhão manso submetido a estresse salino e adubação fosfatada. Rev. Caatinga. 25(2):144-152. |
|
Tester M, Davenport R (2003). Na+ tolerance and Na+ transport in higher plants. Ann. Bot. 91(5):503-527.
Crossref |
|
Viana EM (2007).Interação de nitrogênio e potássio na nutrição, no teor de clorofila e na atividade da redutase do nitrato em plantas de trigo. Master’sDissertation. Escola Superior de Agricultura Luiz de Queiroz, Piracicaba. P. 96. |
|
Zhou W, Sun Q, Zhang C, Yuan Y, Zhang J, Lu B (2004). Effect of salt stress on ammonium assimilation enzymes of the roots of rice (Oryza sativa) cultivars differing in salinity resistance. Acta. Bot. Sin. 46(8):921-927. |
|