ABSTRACT
Agronomic biofortification of staple food crops with micronutrients important for human nutrition, such as selenium (Se), is currently being advocated to address widespread deficiencies in the diets of populations in sub-Saharan Africa. Previous research has shown that there is likely to be widespread dietary Se deficiency in Malawi due to low concentration of Se in edible parts of the staple crops, such as maize (Zea mays L), on low-pH soils, but that this could be addressed through agronomic biofortification using Se-enriched fertilisers. Farmers often intercrop maize with legumes such as groundnut (Arachis hypogaea) and soybean (Glycine max). Therefore, a field study during the 2012/2013 cropping season examined the effect of foliar application of Se on its concentration in grains and stover of maize, soybean and groundnut grown as intercrops or sole crops at three sites in Malawi. Mean Se concentrations were highest in soybean seed, followed by groundnut seed and maize grain, both in plots with added Se and without. Application of 10 g ha-1 of Se increased Se concentration in maize grain by 8-fold, in groundnut seed by 9-fold and in soybean seed by 18-fold; thus universal adoption could increase estimated average dietary Se supply in Malawi from between 21 and 31 µg cap-1 d-1 to between 68 and 78 µg cap-1 d-1.
Key words: Selenium, intercropping, food security, hidden hunger, biofortification, fertilizers, mineral micronutrient deficiencies.
SeleniumThe element selenium (Se) is an essential element in the nutrition ofnutrient for humans and livestock. A total of 25 selenoproteins have been identified in humans including iodothyronine deiodinases, thioredoxin reductases, glutathione peroxidases and a range of other selenoproteins, with critical roles in thyroid functioning, cell proliferation, antioxidant defence and the immune response (Fairweather-Tait et al., 2011). Chronic and extremely low levels of Se intake leading to concentrations in blood plasma levels of <20-40 µg L-1 are thought to be a major underlying cause of Keshan disease (a cardiomyopathy) and Kashin-Beck disease (an osteoarthropathy). Moderate deficiencies (blood plasma <100 µg L-1) resulting from low dietary intakes or malabsorption and high losses of Se (e.g. due to infection such as HIV) can cause immune dysfunction and increased viral pathogenicity and low Se intakes have been associated with certain cardiovascular disorders and cancers (Beck, 2007; Fairweather-Tait et al., 2011).
Suboptimal dietary Se intake is likely to be widespread in Malawi due to limited phytoavailability of Se in the predominant low-pH soils, and narrow food choices including limited animal-source products (Eick et al., 2009; Chilimba et al., 2011; Gibson et al., 2011). Through analysis of composite diet samples, Hurst et al. (2013) found median intake of Se for adult women in rural Malawi of just 6.8 µg cap-1 d-1 (median absolute deviation, MAD=2.9, range=1.1-62.3, n=56) in an area of low-pH soils but 55.3 µg cap-1 d-1 (MAD=25.9, range=5.8-192, n=58) in an area of calcareous soils with pH >6.5. This compares to the adult female estimated average requirement (EAR) of 45 µg d-1 (IOM, 2000). The EAR is the average daily nutrient intake that meets the requirements of 50 percent of apparently healthy individuals in a particular age and sex group.
Chilimba et al. (2012) reported a linear response in maize grain and stover Se concentration to selenium application using a variety of application methods and rates (R2 > 0.90). For each g ha-1 of Se applied, the Se concentration increased by 11-29 and 3-21 µg kg-1 in grain and stover, respectively. Annual application of 5 g ha−1 of Se to maize crops grown on low-pH soils in Malawi would raise average dietary Se supply by 26-37 µg person-1 d-1, greatly reducing risks of dietary Se deficiency. Agronomic biofortification via Se-enriched fertilisers might therefore be a cost-effective way to address widespread Se deficiency in Malawi, and could follow the policy-precedent of Finland which has successfully increased dietary Se intake through Se biofortification of major crops since 1984 (Eurola et al., 2004; Eurola, 2005; Lyons et al., 2005; Broadley et al., 2006; White and Broadley, 2009; Broadley et al., 2010; Alfthan, 2013).
Current knowledge suggests that Se recovery by crops is inefficient and applied Se is likely to be rapidly leached as soluble selenate (SeVIO42-), adsorbed as selenite (SeIVO32-; pK2 = 7.3) or immobilised into organic forms (Mayland et al., 1991; Fordyce, 2013; Gabos et al., 2014). For example, Sager and Hoesch (2006) reported that between 0.7 and 4.7% of applied Se was transferred to barley grain. Chilimba et al., (2012) observed recovery rates in maize grain of 6.5 and 10.8% after applying 10 g Se ha−1 at two contrasting sites in Malawi, but in the subsequent residual year < 0.1% of the original Se application was recovered in the crop. These studies were conducted using sole crops, whereas many smallholder farmers in Malawi intercrop maize with other species including legumes and root and tuber crops. It is likely that Se recovery by different crops will differ and suspected that intercropping systems may also influence Se recovery by individual species. The objectives of this study were (i) to determine Se concentration in grains and stover of crops grown in sole and intercropping systems with, and without, application of Se-enriched fertiliser, and (ii) to evaluate the effectiveness of different cropping systems in recovering applied Se.
Experiments were conducted during the 2012/13 growing season at three research stations of the Malawi Ministry of Agriculture and Food Security: Chitedze Research Station, Zombwe Extension Planning Area and Lunyangwa Research Station (Table 1). The experimental treatments consisted of five cropping systems: monocrop maize, monocrop groundnuts, monocrop soybean, intercrop maize/groundnuts and intercrop maize/soybean. There were two application rates of Se: 0 and 10 g ha-1. The experimental plots were laid out in a randomised complete block design with three replicates. The gross plots contained four ridges of 30 cm height, spaced 75 cm apart and 5 m long. The net plot data were collected comprised 4 m lengths of the two central ridges.
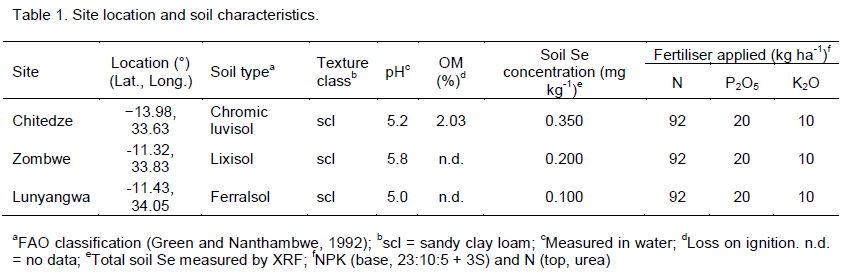
Maize (variety SC627) was used with four seeds per planting station at 75 cm intervals along the ridges, thinned to three plants per planting station after emergence. Soybean (variety Ocepara-4) was used with one seed per planting station at 2.5 cm intervals along the ridges. Rhizobia inoculation was achieved by preparing a mixture of 200 ml of 5% sugar solution and one 50 g sachet of rhizobium inoculant to form a slurry which was poured over the soybean seed and mixed until all seeds were evenly covered; planting of the seed was undertaken on the same day. Groundnut (variety CG7) was used with one seed per planting station at 15 cm intervals along the ridges. Planting spacing for intercrops was identical to the corresponding sole crop. Seeds of the intercropped species were planted between the maize planting stations.
The Malawi national fertiliser recommendation was used with base application of N, P2O5 and K2O (46, 20 and 10 kg ha-1) soon after seed emergence using a 23:10:5 +3S fertiliser and a top dressing of urea at 46kg N ha-1 applied two weeks after basal dressing (Table 1). Sodium selenate (Na2SeO4(aq)) solution containing 15.0 mg L-1 of Se was applied to the gross plot area at early stem extension stage (~‘knee high’). To ensure even application to the crop, the Na2SeO4(aq) was applied as a high-volume drench (667 ha-1 of water) using a knapsack sprayer, with the operator wearing personal protective equipment of overalls, boots, face-shield and nitrile gloves (Broadley et al., 2010). A 16 L Berthoud Vermorel 2000Pro knapsack tank (Exel GSA, Villefanche-sur-Saône, France) was connected to a 1 m boom, housing three Lurmark 110°, flat-fan spray nozzles (Hypro EU Ltd, Longstanton, Cambridge, UK), spaced equally, with a spray-swath of 1.5 m. A coarse nozzle type “08 white” was used (1180 ml nozzle-1 min-1; British Crop Protection Council, 2001) to minimise potential for aerosol drift. Ergonomically acceptable drench rates were calibrated to treat four replicate plots from a single tank at appropriate walking speed with two passes.
Maize and legumes were harvested and weighted to determine biomass and grain yield when the crop was mature and had dried in the field to a moisture content of approximately 15%. Sub-samples of biomass and grain were collected and dried in drying ovens at Lunyangwa and Chitedze research stations at 65 °C for 24 h in preparation for elemental analyses including Se. grainWhole grains and stover were milled in a kitchen blender before shipping to the UK. Samples (∼0.4 g dry weight, DW) were digested with microwave heating for 45 min at a controlled pressure of 2 MPa in 3.0 ml of 70% trace analysis grade (TAG) HNO3, 2.0 ml H2O2 and 3.0 ml Milli-Q water (Fisher Scientific UK Ltd, Loughborough, Leicestershire, UK). The microwave system comprised a Multiwave 3000 platform with a 48-vessel 48MF50 rotor (Anton Paar GmbH, Graz, Austria). Samples were digested in vessels comprising perfluoroalkoxy (PFA) liner material and polyethylethylketone (PEEK) pressure jackets (Anton Paar GmbH). Digested samples were diluted to 20 ml (30% HNO3) with Milli-Q water (18.2 MΩ cm) and stored at room temperature pending elemental analysis. Immediately prior to analysis, samples were diluted 1-in-10 with Milli-Q water. Selenium (78Se) analysis was undertaken by ICP-MS (X-SeriesII, Thermo Fisher Scientific Inc., Waltham, MA, USA) using a hydrogen reaction cell. Samples were introduced from an autosampler (Cetac ASX-520, Omaha, NE, USA) with 4 × 60-place sample racks, at 1 ml min−1 through a concentric glass venturi nebuliser and Peltier-cooled (3°C) spray chamber (Thermo Fisher Scientific Inc.). Internal standards introduced to the sample stream via a T-piece included Ge and Rh (10 µg L−1) in 2% TAG HNO3 and 2% methanol to enhance Se ionization in the plasma. An external wheat flour standard (NIST 1567a; National Institute of Standards and Technology, Gaithersburg, MD, USA) was used as reference material. Each digestion batch (n=48) included two blank digestions and two certified reference samples; final Se concentrations were converted to mg kg−1 DW.
Statistical analysis was conducted in GenStat (V.16.1.10916, VSN International, Hemel Hempstead, UK). ANOVA was performed to determine the influence of Se application, crop-type, site and intercropping versus monocropping on Se concentrations in plant tissues. Data were not transformed prior to ANOVA on the basis of visual inspection and a Shapiro-Wilk test for normality. The efficiency of recovery (RSe, %) of exogenous Se for each crop component was calculated as:
Where: Y = fresh-weight yield of the crop component (kg ha-1); M = moisture content (proportion) of the crop component; CSe = Se concentration in crop component (mg kg-1 DW) with exogenous Se application; C0 = Se concentration in the crop component (mg kg-1 DW) without exogenous Se application; FSe = rate of exogenous Se application (mg ha-1).
Recovery efficiency of plots was calculated as the sum of crop components. For calculation of rates of recovery, maize grain moisture content was assumed to be 15%, the national standard for moisture level at harvest. USDA moisture content data (USDA-ARS, 2013) was used for groundnut (6.91%, “Peanuts, virginia, raw”) and soybean (8.54%, “Soybeans, mature seeds, raw”). Moisture content of 15% was assumed for stover of all crop types.
Some samples were excluded from Se analysis due to low yield; termite damage contributed to missing samples of soybean biomass. Yield data was combined across sites to form average yields for each crop. Three samples (two soybean seed and one groundnut seed from plots without Se application) were identified as outliers, defined as Se concentration in excess of three standard deviations from the mean. These samples were presumed to be contaminated, either in the field or post-harvest, so were excluded from statistical analysis. The influence of exogenous Se application, crop-type, site and intercropping versus monocropping was tested using ANOVA (unbalanced design; Table 1).
Application of Se at 10 g ha-1 significantly increased Se concentration in crops p<0.001; Tables 2 and 3). There were also significant differences in Se concentration between crop types (p<0.001 but not between sites (p=0.242; Tables 2 and 3). The ANOVA detected a significant influence of intercropping on Se concentration at the 95% level (p=0.038), with crops from intercropped plots having higher mean Se concentration. This might have been due to the denser and more varied canopy cover of intercrop versus monocrop stands which could have spread the arrival of exogenous Se to the root zone, effectively slowing the rate of Se and deposition to the soil surface and thereby increasing opportunity for root-uptake prior to Se being leached, immobilised into organic forms or strongly adsorbed as selenite following reduction in the soil. Greater foliar interception may also have increased the amount of foliar absorption through the leaf epidermis. However, further investigation for each crop-type at both Se application rates using two -sample t-tests did not find significant differences at the 95% level between samples that were intercropped versus those that were monocropped. Sample sizes may have been insufficient.
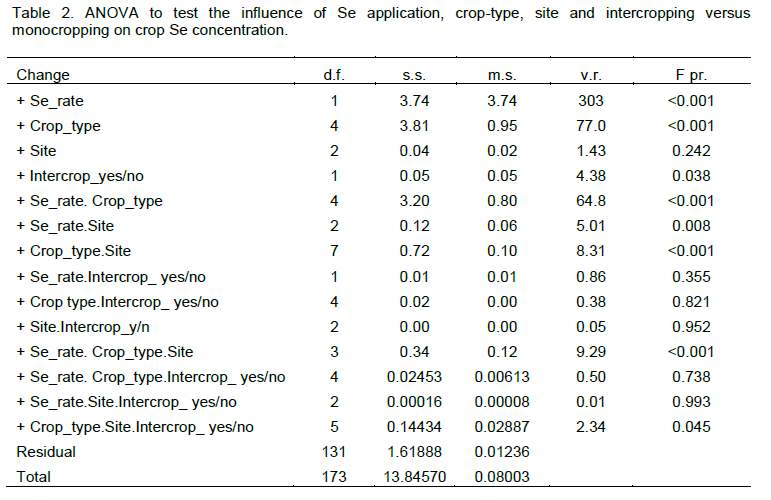
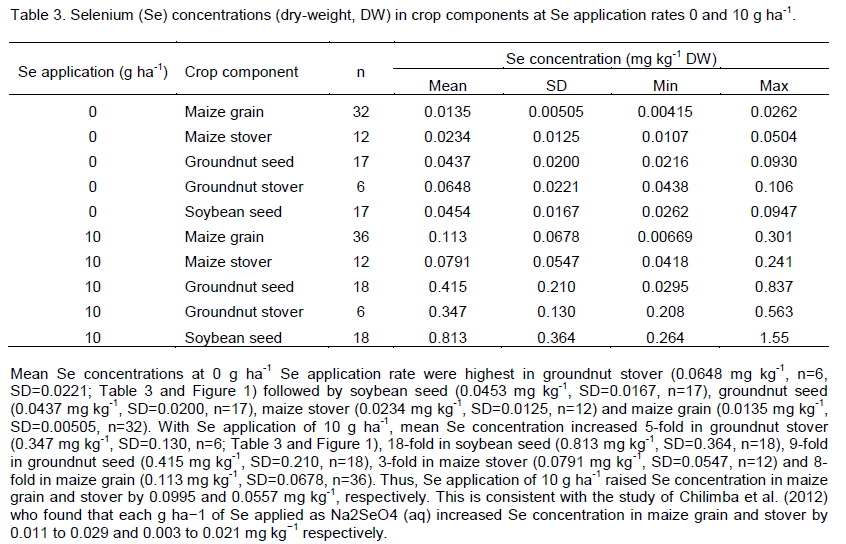
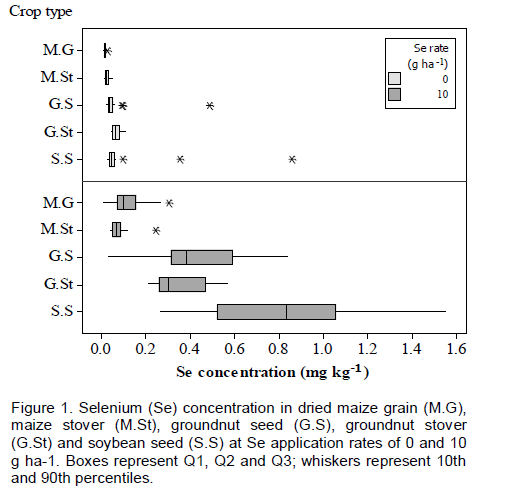
Application of Se did not significantly affect yield of any crop. However, mean maize stover yield in intercropped plots (2,640 kg ha-1, SD=1550, n=18) was less than sole-cropped maize (4,210 kg ha-1, SD=1730, n=26) (t(24)=2.30, p=0.030), mean maize grain yield in intercropped plots (1,940 kg ha-1, SD=1050, n=18) was less than that in sole-cropped maize (3,120 kg ha-1, SD=1260, n=24) (t(22)=2.14, p=0.044), and mean groundnut seed yield in intercropped plots (551 kg ha-1, SD=89.5, n=6) was less than that in sole-cropped groundnuts (908 kg ha-1, SD=242, n=11) (t(9)=3.63, p=0.006). There were no significant differences in yields of soybean seed or groundnut stover due to intercropping. Soybean stover yield could not be tested due to insufficient samples. Negative yield effects of intercropping are likely to be due to competition for water and nutrients and shading effects (Natarajan and Willey, 1986; Yunusa, 1989). However, when the edible components of intercrop plots were combined, mean yields (2,630 kg ha-1, SD=974, n=18) were greater than those of sole crops (1,850 kg ha-1, SD=1500, n=16), although the difference was only marginally significant (t(32)=-1.81, p=0.080).
The cropping system with the highest proportional recovery (RSe) of exogenous Se was sole groundnut (14.7%; Table 4), followed by maize/groundnut intercrop (12.7%) and maize monocrop (4.6%). Soybean seed recovered 7.4% of exogenous Se when monocropped and 10.5% when intercropped with maize. Due to missing data for soybean stover it was not possible to calculate Se recovery in sole soybean or soybean/maize intercrop treatments. Uptake of added Se is generally greater in legumes than cereals (Bisbjerg and Gissel-Nielsen, 1969) and this might be related to protein content as the most common form of Se in plants is generally Se-methionine (Tapiero et al., 2003). With Se fertiliser applied, the cropping system that yielded the most Se in the edible parts (i.e. seed fraction) of the crop was maize/soybean intercrop (1,300 mg ha-1), followed by soybean sole crop (785 mg ha-1), maize/groundnut intercrop (400 mg ha-1), groundnut sole crop (351 mg ha-1) and finally maize sole crop (300 mg ha-1).
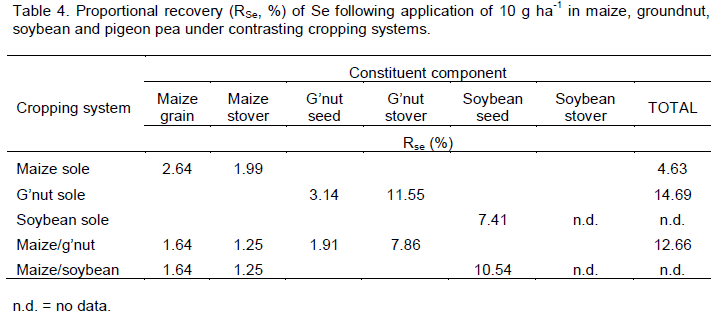
The average amounts of maize, groundnut and soybean (fresh-weight) available for consumption in Malawi are 365, 14 and 7 g cap-1 d-1 according to food balance sheets from the United Nations Food and Agriculture Organization (FAO, 2012). Using composition data for crops at 0 g ha-1 Se application, the estimated supply of Se from maize, groundnuts and soybean (that is, 4.2, 0.6 and 0.2903 µg cap-1 d-1, respectively) appears to contribute little towards dietary Se requirements considering an EAR for adult women of 45 µg cap-1 d-1 (IOM, 2000). With universal coverage of Se biofortification at 10 g ha-1, maize, groundnuts and soybean would supply an average of 35.1, 5.4 and 5.2 µg cap-1 d-1 of Se, respectively. Average dietary supply of Se from sources other than maize, groundnut and soybean is likely to range between 15 and 25 µg cap-1 d-1 in Malawi (Donovan et al., 1992; Eick et al., 2009). Average dietary Se intake would therefore range between 61 and 71 µg cap-1 d-1 which is likely to be optimum for most people (Fairweather-Tait et al., 2011), and provides minimal risk of overdose based on a current safe upper limit of 400 µg Se person-1 d-1 (IOM, 2000). There may be further benefits of Se biofortification on livestock health since cattle and goats are commonly fed on maize stover residue during the dry season. For example, >6 mg d-1 of supplemental Se is required to optimise serum Se status of dairy cattle (Gerloff, 1992). Feed containing >0.1 mg kg-1 Se will protect against Se deficiency disorders (Girling, 1984); this compares to concentrations of Se in maize stover of 0.079 and 0.023 mg kg-1 with and without Se application, respectively.
Risks of negative environmental or health impacts due to Se toxicity are minimal with agronomic biofortification at 10 g ha-1 yr-1 of Se. Selenium toxicity can occur over some sedimentary rocks, e.g. the black shale and sandstone deposits of the Great Plains in the USA, where concentrations of total Se in the soil are high (1-10 mg kg-1) and the soil environment alkaline (Muth and Allaway, 1963). However, Oldfield (1999) report that soils with up to 20 mg kg-1 total Se did not cause problems to vegetation and livestock in humid lateritic soils in Hawaii. Application of Se at 10 g ha-1 yr-1 is equivalent to 0.0036 mg kg-1 topsoil, assuming 2.8 t ha-1 topsoil, and it would take >5 k yr to reach a concentration of 20 mg kg-1 Se in the topsoil.
Dietary Se deficiency appears to be widespread in Malawi, on the basis of crop, soil, diet composite, blood and urine surveys (Eick et al., 2009; Chilimba et al., 2011; Gibson et al., 2011; Hurst et al., 2013). Effective biofortification of the staple grain maize with Se through sodium selenate application has been demonstrated previously (Chilimba et al., 2012). This study measured the effect of sodium selenate application on Se concentration in maize, groundnut and soybean in sole- and intercrop systems at three sites with low-pH soils. Foliar application of 10 g ha-1 of Se in the form of a sodium selenate liquid drench was effective in increasing Se concentration in maize grain by 8-fold, groundnut seed by 9-fold and soybean seed by 18-fold. Considering all grain and stover components combined, recovery efficiency of exogenous Se was greater in groundnuts as sole crop and maize/groundnut intercrop compared to maize sole-crop. Considering only the edible portion of grain, maize-soybean intercrop provided the highest yield of Se (1,310 mg ha-1). Universal adoption of Se-enriched fertiliser would lead to a 150-225% increase in estimated average dietary supply of Se, from between 21 and 31 µg cap-1 d-1 to between 68 and 78 µg cap-1 d-1. Further research is now required to validate this estimate at wide scales and to monitor impact on human health and nutrition.
The authors have not declared any conflict of interest.
Funding and support for this study was provided by Malawi Government Ministry of Agriculture and Food Security and the University of Nottingham, UK. Funding for EJMJ’s PhD Studentship is provided by the University of Nottingham and British Geological Survey. ADCC conceived and designed the experiment. EJMJ and SDY analysed the samples. EJMJ conducted data analyses. All authors were involved in drafting and reviewing the manuscript.
REFERENCES
Alfthan G (2013). Effects of nationwide addition of selenium to fertilizers on foods and animal and human health in Finland, in: Banuelos, G.S., Zhi-Qing, L, Xuebin Y (Eds.), Selenium in the Environment and Human Health. CRC Press, Florida pp. 111–112.
Crossref |
|
Beck MA (2007). Selenium and vitamin E status: Impact on viral pathogenicity. J. Nutr. 137:1338–1340. PMid:17449602 |
|
Bisbjerg B, Gissel-Nielsen G (1969). The uptake of applied selenium by agricultural plants. I: The influence of soil type and plant species. Plant Soil 31:287–298.
Crossref |
|
Broadley MR, Alcock J, Alford J, Cartwright P, Fairweather-Tait SJ, Foot I, Hart DJ, Hurst R, Knott P, McGrath SP, Meacham MC, Norman K, Mowat H, Scott P, Stroud JL, Tovey M, Tucker M, White PJ, Young SD, Zhao FJ (2010). Selenium biofortification of high-yielding winter wheat (Triticum aestivum L.) by liquid or granular Se fertilisation. Plant Soil 332:5–18.
Crossref |
|
Broadley MR, White PJ, Bryson RJ, Meacham MC, Bowen HC, Johnson SE, Hawkesford MJ, McGrath SP, Zhao FJ, Breward N, Harriman M, Tucker M (2006). Biofortification of UK food crops with selenium (Se). Proc. Nutr. Soc. 65:169-181.
Crossref |
|
Chilimba ADC, Young SD, Black CR, Rogerson KB, Ander EL, Watts M, Lammel J, Broadley MR (2011). Maize grain and soil surveys reveal suboptimal dietary selenium intake is widespread in Malawi. Sci. Rep. 1:72.
Crossref |
|
Chilimba ADC, Young SD, Black CR, Meacham MC, Lammel J, Broadley MR (2012). Agronomic biofortification of maize with selenium (Se) in Malawi. Field Crop Res. 125:118–128.
Crossref |
|
Donovan UM, Gibson RS, Ferguson EL, Ounpuu S, Heywood P (1992). Selenium intakes of children from Malawi and Papua New Guinea consuming plant-based diets. J. Trace Elem. Electrolytes Health Dis. 6:39–43. |
|
Eick F, Maleta K, Govasmark E, Duttaroy AK, Bjune AG (2009). Food intake of selenium and sulphur amino acids in tuberculosis patients and healthy adults in Malawi. Int. J. Tuberc. Lung Dis. 13:1313–1315. |
|
Eurola M (2005). Proceedings: Twenty Years of Selenium Fertilization, September 8-9, 2005, Helsinki, MTT Agrifood Research, Finland. |
|
Eurola M, Hietaniemi V, Kontturi M, Tuuri H, Kangas A, Niskanen M, Saastamoinen M (2004). Selenium content of Finnish oats in 1997–1999: effect of cultivars and cultivation techniques. Agric. Food Sci. 13:46–53.
Crossref |
|
Fairweather-Tait SJ, Bao Y, Broadley MR, Collings R, Ford D, Hesketh J, Hurst R (2011). Selenium in human health and disease. Antioxid. Redox Sign. 14:1337–1383.
Crossref |
|
Food and Agriculture Organization (FAO) (2012). Food Balance Sheet data. http://faostat.fao.org [accessed March 2012] |
|
Fordyce FM (2013). Selenium Deficiency and Toxicity in the Environment. In: Selinus et al. (Eds) Essentials of Medical Geology: Revised Edition, Natural Environment Research Council, UK pp. 375–416.
Crossref |
|
Gabos MB, Goldberg S, Alleoni LRF (2014). Modeling selenium (IV and VI) adsorption envelopes in selected tropical soils using the constant capacitance model. Environ. Toxicol. Chem. 33:2197–2207.
Crossref |
|
Gerloff BJ (1992). Effect of selenium supplementation on dairy cattle. J. Anim. Sci. 70:3934–3940. |
|
Gibson RS, Bailey KB, Ampong Romano AB, Thomson CD (2011). Plasma selenium concentrations in pregnant women in two countries with contrasting soil selenium levels. J. Trace Elem. Med. Biol. 25:230–235.
Crossref |
|
Girling CA (1984). Selenium in agriculture and the environment. Agric. Ecosyst. Environ. 11:37–65.
Crossref |
|
Green R, Nanthambwe S (1992). Land resources appraisal of the Agricultural Development Divisions. Ministry of Agriculture/United Nations Development Programme/Food and Agriculture Organization MLW/85/011. Field Document No. 32, Lilongwe, Malawi. |
|
Hurst R, Siyame EWP, Young SD, Chilimba ADC, Joy EJM, Black CR, Ander EL, Watts MJ, Chilima B, Gondwe J, Kang'ombe D, Stein AJ, Fairweather-Tait SJ, Gibson RS, Kalimbira AA, Broadley MR (2013). Soil-type influences human selenium status and underlies widespread selenium deficiency risks in Malawi. Sci. Rep. 3:1425.
Crossref |
|
Institute of Medicine (IOM) (2000). Dietary Reference Intakes for Vitamin C, Vitamin E, Selenium, and Carotenoids. National Academies Press, Washington D.C. |
|
Lyons GH, Ortiz-Monasterio I, Stangoulis JCR, Graham RD (2005). Selenium concentration in wheat grain: Is there sufficient genotypic variation to use in breeding? Plant Soil 269:269–380.
Crossref |
|
Mayland HF, Gough LP, Stewart KC (1991). Selenium mobility in soils and its absorption, translocation, and metabolism in plants. In: Severson RC, Fisher JR, Scott E, Gough LP (Eds.), Proceeding of the 1990 Billings land reclamation symposium on selenium in arid and semiarid environments, Western United States, US Geol. Sur. Circ., 1064(1991):57–65. |
|
Muth OH, Allaway WH (1963). The relationship of white muscle disease to the distribution of naturally occurring selenium. J. Am. Vet. Med. Assoc. 142:1379–1384. PMid:13936909 |
|
Natarajan M, Willey RW (1986). The effects of water stress on yield advantages of intercropping systems. Field Crop Res. 13:117–131.
Crossref |
|
Oldfield JE (1999). 'Selenium World Atlas.' Selenium-Tellurium Development Association, Grimbergen, Belgium. |
|
Sager M, Hoesch J (2006). Selenium uptake in cereals grown in lower Austria. J. Cent. Eur. Agric. 7:71–77. |
|
Tapiero H, Townsend DM, Tew KD (2003). The antioxidant role of selenium and seleno-compounds. Biomed. Pharmacother. 57:134-144.
Crossref |
|
US Department of Agriculture, Agricultural Research Service (USDA-ARS) (2013). National Nutrient Database for Standard Reference, Release 26.
View
|
|
White PJ, Broadley MR (2009). Biofortification of crops with seven mineral elements often lacking in human diets - iron, zinc, copper, calcium, magnesium, selenium and iodine. New Phytol. 182:49–84.
Crossref |
|
Yunusa IAM (1989). Effects of planting density and plant arrangement pattern on growth and yields of maize (Zea mays L.) and soya bean (Glycine max (L.) Merr.) grown in mixtures. J. Agric. Sci. 112:1–8.
Crossref |