ABSTRACT
The seeds were quasi-statically loaded to determine rupture force, toughness, yield force, rupture stress, modulus of stiffness and elasticity. Compression tests were carried out on seeds at moisture content of 15.76, 19.81 and 34.43% (wb), seed orientation in both the longitudinal and transverse axis at a loading rate of 25 mm/min. Values of the rupture force, toughness, rupture stress, yield force and modulus of stiffness of the seeds decreased linearly from 362.04 to 168.82 N, 1.783 to 0.623 J, 7.4 to 3.15 N/mm2, 213.42 to 89.68 N and 39.6 to 21.97 N in transverse axis and from 276.64 to 195.26 N, 1.355 to 0.641 J, 16.12 to 6.23 N/mm2, 211.58 to 124.72 N and 38.74 to 24.77 N in longitudinal axis with increase in moisture content from 15.76 to 34.43 (%wb). Values of modulus of elasticity determined using hertz theory of parallel plate contact increased from 1.529 to 3.52 MPa with increase in moisture content from 15.76 to 19.81 (%wb) but decreased to 1.563 MPa with increase in moisture content. Highest value for rupture force (362.04 N) and toughness (1.783 J) of the seeds were obtained at a moisture content of 15.76% wb. The seeds are more flexible in longitudinal orientation and the rupture force requires less energy under longitudinal loading than transverse loading.
Key words: African oil bean seeds, mechanical properties, compressive tests, moisture content, loading orientation.
African oil bean seed (Pentaclethra macrophylla Benth) belongs to the Leguminosae family and the sub-family of Mimosoideae (Keay, 1989) with no known varietal characterization. It is a tropical tree crop found mostly in the southern and middle belt regions of Nigeria and in other coastal parts of West and Central Africa. The seed is planted or retained along the edges of home gardens and farms mainly for its seed from which edible oil can be extracted (Oboh, 2007). African oil bean seed has been cultivated in Nigeria since 1937 (Ladipo, 1984) and for many years in other West African countries where its seed is relished as a food. Some parts of the plant also have medicinal values.
The seeds of the African oil bean are edible when boiled and fermented especially among the eastern part of Nigeria. The seed when cooked, processed and fermented is called ‘ugba’ (in Igbo language of Nigeria) and is used for the preparation of many delicious delicacies like African salad, soups and sausages for eating with different staples (Enujiugha, 2003). The seed is a source of edible oil; it contains more than 52% oil in its cotyledons (Enujiugha and Agbede, 2000). Their edible seeds require tedious but careful processing and fermentation before they can be eaten as food supplement. The seeds are eaten alone with other ingredients like stockfish, garden egg, sliced tapioca or they can be mixed with vegetables popularly known as “African salad” among the Eastern part of Nigeria (Okafor and Fernandez, 1987).
Mechanical properties of agricultural materials affects their processing, handling storage and consumption. These properties are important in the design of planters, harvesters and in postharvest operations such as cleaning, conveying and storage (Balasubramanian et al., 2012; Asoegwu et al., 2006). Presently, all the post harvest handling and processing practices are done manually and it is necessary to design tools, equipment and machines for the above processes (Asoegwu et al., 2006). Design of these equipments without putting these properties into consideration may yield poor results (Polat et al., 2007).
Some physical properties of the African oil bean seed were studied by (Asoegwu et al., 2006) but no information appears to have been reported on the mechanical properties of the seeds and their relationship with moisture content.
The objective of this research is to study the effects of moisture content and loading orientation on some mechanical properties of the African oil bean seeds.
Sample preparation
Samples of the African oil bean seeds were obtained from a local
market in Ojoo, Ibadan. The seeds were manually cleaned to remove defective ones and other foreign materials before conducting the tests as presented in Plate 1.
The initial moisture content of the African oil bean was determined using the moisture analyzer and it was found to be 8.25% wb. The samples were divided into three parts and a method for adjusting the seeds moisture content without damage in its morphology was developed to prepare samples for subsequent tests. The seeds were oven dried at 105°C for 16 h until there was no change in its mass. The desired moisture levels were obtained by soaking each of the divided samples in water for different time intervals. In this method, the initial moisture content (8.25% wb) was used with the value of quantity of water absorbed by the seed using the mass balance equation, moisture content value obtained are 15.76, 19.81 and 34.43%.
The conditioned seed were put in cellophane bags and stored in a refrigerator at 5°C for one week to have uniform moisture distribution throughout samples. The required seed quantity was taken out of the refrigerator and allowed to equilibrate to room temperature before each test (Kara et al., 2010).
Compression tests
Quasi-static compression tests were performed with an Instron Universal Testing Machine (Model M500) with a 25 KN compression load cell and Integrator. Tests were conducted at room temperature of 30°C at the five moisture level. In this study a total of 90 seeds were compressed. The three perpendicular dimensions of the seeds were measured with a micrometer and recorded before each test. The individual seeds were loaded between parallel plates of the machine and compressed until rupture occurred as is denoted by a rupture point in the force deformation curve. To determine the effect of the orientation of loading, the grain was positioned horizontally with the major axis of the grain being normal to the direction of loading, or lengthwise as shown in Figure 1. For vertical loading the major axis of the grain was parallel to the direction of loading as shown in Figure 1b. The deformation (strain) was taken as the change in the original dimension of the grain. The energy required for causing rupture (failure) in the grain was determined by calculating the area under the force-deformation curve up to grain rupture. The results and the force-deformation curves obtained at each loading orientation and moisture level were analyzed for the following:
a. Rupture force
b. Yield force
c. Rupture stress
d. Toughness
e. Modulus of Stiffness
f. Modulus of elasticity
The rupture force was taken as the point on the force-deformation curve, at which the compressed shell became completely broken and torn with the kernel exposed and the rupture stress was obtained as the ratio of rupture force and cross sectional area of the seeds.
The modulus of stiffness was taken as the ratio of the average maximum force to the average maximum deformation of the shell; it was calculated from the force-deformation data of the shell following the method employed by Aviara and Ajikashile (2010).
The modulus of elasticity, which is defined by Hertz’s expression for modulus of elasticity, was calculated using a method employed by Adgidzi et al. (2006). The values of Forces ‘F’ and Deformation ‘D’ for each shell were gotten from the force-deformation data. The value of Poisson ratio was taken to be 0.35 (Mohesnin, 1970):
Where: E is the modulus of elasticity,

is the poisson ratio (0.35), D is the deformation, R and R
1 are radius of curvature of seed samples, F is the rupture force, H is the minor diameter and L is the major diameter.
Rupture force (F)
The rupture force was taken as the point on the force-deformation curve, at which the compressed shell became completely broken and turned with the kernel exposed. Tested and calculation values are plotted and Figure 2 shows that as moisture content reduced, rupture force increased. The average force required to initiate seed rupture decreased from 362.04 to 168.82 N and 276.64 to 195.26 N in the transverse and longitudinal axis with an increase in moisture content from 15.76 to 34.43 (%wb) respectively.
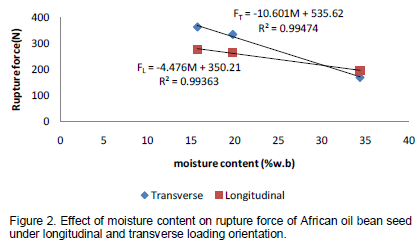
A similar trend was reported by Fathollahzedah and Rajabipour (2008) and Kiani Deh Kiani et al. (2008) in the compression of red beans grains and barberry respectively and they both attributed this to the fact that as the moisture content reduced, the tissue of the nuts became tougher. Saiedired et al (2007) in their work on the mechanical properties of Cummin seeds also observed that the force required to initiate seed rupture decreased from 58.2 to 28.8 N as moisture content increased from 5.7 to 15 (%db) and they also attributed this to the fact that at higher moisture content, the seeds were softer and required less force. The relationship between rupture force, loading orientation and moisture content may be expressed by the following regression equations:
FT = -10.601M - 535.62 (R2 = 0.9947) (4)
FL = -4.476M + 350.21 (R2 = 0.9936) (5)
Toughness (E)
Toughness was taken as the energy absorbed by the shell at which the shell got completely broken. Values obtained as shown in Figure 3 showed that as moisture content reduces the value for toughness increased. The toughness averagely decreased from 1.783 to 0.623 J and 1.355 to 0.641 J in the transverse and longitudinal orientations respectively with the toughness being more under transverse orientation. A similar trend was observed in the compression of Hemp seed by Taheri-Garavand et al. (2010) and also by Maghsoudi et al. (2012) in the determination of the rupture energy of the Badami variety of unsplit Pitaschio nut and they attributed this to the hardness of the shell at lower moisture content making it absorb more energy at lower moisture contents during compression.
The relationship between toughness, loading orientation and moisture content may be expressed by the following regression equations:
ET = -0.063M + 2.7976 (R2 = 0.9983) (6)
EL = -0.0197M + 1.3011 (R2 = 0.7107) (7)
Rupture stress (ST)
The rupture stress decreased from 7.4 to 3.15 N/mm2 and from 16.12 to 6.23 N/mm2 as moisture content increases from 15.76 to 34.43 (%wb) in the transverse and longitudinal orientations respectively; the rupture stress was higher under transverse loading than longitudinal loading. Similar trends were reported by Fadeyibi and Osunde (2012) and Fadeyibi (2012) in the compression of Rubber seed, and Haddad et al. (2001) and Kang et al. (1992) for wheat kernels in their works on the mechanical properties of wheat kernels. Figure 4 shows the linear relationship between the rupture stress, loading orientation and moisture content.
ST = -0.5139M + 23.812 (R2 = 0.9912) (8)
SL = -0.195M + 9.6295 (R2 = 0.792) (9)
Yield force (Y)
The yield force was taken as the point on the force-deformation curve, at which the visible failure of the nutshell became initiated and the shell just began to tear. The variation of the yield force of African oil bean seed with moisture content when subjected to compression in the transverse and longitudinal orientation as shown in Figure 5 shows that the yield force averagely decreased from 213.42 to 89.68 N and 211.58 to 124.72 N in the transverse and longitudinal direction respectively. This indicates that the minimum force needed in the compressive cracking of African oil bean shell to initiate the failure of the shell at the macroscopic level is moisture dependent. A similar trend was observed by Aviara and Ajikashile (2011) and they attributed this to the fact that at higher moisture content, the seeds were softer and required less force. Also Tavakoli et al. (2009a) and Tavakoli et al. (2009b), attributed the same effect to the elastic behavior of Agricultural material at lower ranges of moisture content. The relationship between the yield force, loading orientation and moisture content may be expressed by the following regression equations:
YT = -3.1416M + 244.9 (R2 = 0.9982) (10)
YL = -4.8252M + 292.09 (R2 = 0.9881) (11)
Modulus of stiffness (M)
The variation of the modulus of elasticity of the African oil bean seed with moisture content when subjected to compression in the transverse and longitudinal orientation as shown in Figure 6 shows that the modulus of stiffness decreased as moisture content increased. There was an average decrease from 39.60 to 21.97 N and 38.74 to 24.77 N in the transverse and longitudinal direction respectively. Aviara and Ajikashile (2011) reported a similar trend in the compression of Conophor nut. The relationship between the yield force, loading orientation and moisture content may be expressed by the following regression equations:
MT = -0.805M + 48.69 (R2 = 0.7812) (12)
ML = -0.6438M + 46.189 (R2 = 0.8023) (13)
Modulus of elasticity
The variation of the modulus of elasticity of African oil bean seed with moisture content when subjected to compression in the transverse and longitudinal orientation shows that it behaved differently under different loading orientations. The modulus of elasticity averagely increased from 1.529 to 3.528×106 Pa as moisture content increased from 15.76 to 19.81 (w.b) but decreased to 1.563×106 Pa with further increase in moisture content in the transverse axis as presented in Table 1. However, in the longitudinal axis, there was a reverse trend. The modulus of elasticity decreased from 5.253 to 1.405×106 Pa as moisture content increased from 15.76 to 19.81 (w.b) but increased to 2.194×106 Pa) with further increase in moisture content. A similar trend was observed by Adgidzi et al. (2006), that the modulus of elasticity of most soft biomaterials increases with increase in strain (Mohsenin, 1980). It means that for the same material, there will be different values of elasticity depending on the point at which it was calculated.
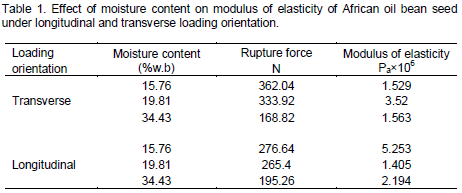
The study of some mechanical properties of the African oil bean seed at various moisture contents and loading orientation suggested the following conclusions:
1. The force required to initiate seed rupture decreased with an increase in moisture content. The rupture force was higher under transverse loading than at longitudinal loading.
2. The rupture energy decreased with an increase in moisture content. The rupture energy was higher under transverse loading than at longitudinal loading. The African oil bean seeds are more flexible in the horizontal loading direction and the rupture under horizontal loading demanded less energy than under vertical loading. This may be due to decreasing contact area of seed with loading.
3. The yield force decreased as moisture content increased from 15.76 to 34.43 (%wb) in the transverse and longitudinal axes respectively. This indicates that the minimum force needed in the compressive cracking of African oil bean shell to initiate the failure of the shell at the macroscopic level is moisture dependent.
4. The rupture stress and modulus of stiffness of the African oil bean seed decreased as moisture content increased.
5. The modulus of elasticity of the African oil bean seeds behaved differently under different loading orientations.
The authors have not declared any conflict of interest.
REFERENCES
Adgidzi D, Akande DB, Dakogol FA (2006). Determination of rheological properties of shea nuts (Butyrospermum paradoxum). J. Agric. Engineer. Technol. 14:18-28. |
|
Asoegwu S, Ohanyere S, Kanu O, Iwueke C (2006). Physical properties of African oil bean seed (Pentaclethra macrophylla Benth). Agricultural Engineering International: CIGR Ejournal. Manuscript FP 05 006. P. 8. |
|
Aviara NA, Ajikashile JO (2011). Effect of moisture content and loading orientation on some strength properties of conophor (Tetracarpidium conophorum) Nut. Agric. Eng. Res. J. 1(1):04-11. |
|
Balasubramanian S, Singh KK, Kumar R (2011). Physical properties of coriander seeds at different moisture content. Int. Agro-phys. 26:419-422. |
|
Enujiugha VN (2003). Nutrient changes during the fermentation of African oil bean (Pentaclethra macrophylla Benth) seeds. Pak. J. Nutr. 2(5):320–323.
Crossref |
|
Enujiugha VN, Agbede JO (2000). Nutritional and anti-nutritional characteristics of African oil bean (Pentaclethra macrophylla Benth) seeds. Appl. Trop. Agric. 5:11-14. |
|
Fadeyibi A (2012). Influence of moisture content on some engineering properties of rubber seed. Department of Agricultural and Bioresources Engineering, fedral University of Technology, Minna, Nigeria. An unpublished Master of Engineering Dissertation pp. 33-38 |
|
Fadeyibi A, Osunde ZD (2012). Mechanical behaviour of rubber seed under compressive loading. Curr. Trends Technol. Sci. 1:2. |
|
Fathollahzadeh H, Rajabipour A (2008). Some mechanical properties of barberry. Int. Agro-phys. 22:299-302. |
|
Haddad Y, Benet JC, Delenne JY, Merme A, Abecassis J (2001). Rheological behaviour of wheat endosperm-proposal for classification based on rheological characteristics of endosperm test samples. J. Cereal Sci. 34:105–113.
Crossref |
|
Kang YS, Spillman CS, Steele JS, Chung DS (1992). Mechanical properties of Wheat. Trans. ASAE. 38(2):573–578.
Crossref |
|
Kara M, Bastaban S, Öztürk I, Kalkan F, Yildiz C (2010). Moisture-dependent frictional and aerodynamic properties of safflower seeds. Int. Agro-phys. 26:203-205 |
|
Keay RWJ (1989). Nigerian Trees. Claredon Press, UK. P. 281. |
|
Kiani Deh Kiani M, Minaei S, Maghsoudi H, Ghasemi VM (2008). Moisture dependent physical properties of red bean (Phaseolus vulgaris L.) grains. Int. Agrophys. 22231-237. |
|
Ladipo DO (1984). Seed problems in fuelwood plantations in Nigeria. Paper prepared for the international symposium on seed quality of tropical and subtropical Species. Bangkok. |
|
Maghsoudi H, Khoshtaghaza MH, Minaei S, Zaki DH (2012). Fracture resistance of Unsplit Pistachio (Pistacia vera L.) nuts against splitting force, under compressive loading. J. Agric. Sci. Tech. 14:299-310. |
|
Mohsenin NN (1980). Physical properties of plant and animal materials. Gordon and Breach Science Publishers. |
|
Oboh G (2007). Pentaclethra macrophylla Benth. In: van der Vossen, H.A.M. & Mkamilo, G.S. (Editors). PROTA 14: Vegetable oils/Oléagineux. [CD-Rom]. PROTA, Wageningen, Netherlands. |
|
Okafor JC, Fernandez EC (1987). Compound farms of southeast Nigeria. A predominant agroforestry homegarden system with crops and small livestock. Agrofor. Syst. 5(2):153–168.
Crossref |
|
Polat R, Atay U, Saglam C (2006). Some physical and aerodynamic properties of soybean. J. Agron. 5(1):74-78.
Crossref |
|
Saiedirad MH, Tabatabaeefar A, Borghei A, Mirsalehi M, Badii F, Ghasemi VM (2008). Effects of moisture content, seed size, loading rate and seed orientation on force and energy required for fracturing cumin seed (Cuminum cyminum Linn.) under quasi-static loading. J. Food Engineer. 86(1):565–572.
Crossref |
|
Taheri-Garavand A Nassiri A, Gharibzahedi SMT (2010). Physical and mechanical properties of Hemp seed. Int. Agrophys. 26:211-215. |
|
Tavakoli H, Mohtasebi SS, Jafari A (2009a). Physical and mechanical properties of wheat straw as influenced by moisture content. Int. Agrophys. 23:175-181. |
|
Tavakoli H, Rajabipour A, Mohtasebi SS (2009b). Moisture-dependent of some engineering properties of soybean grains. Agricultural Engineering International: CIGR Ejournal. Manuscript 1110. 11:54-58. |