ABSTRACT
Developing a protocol for somatic embryogenesis (SE) for adult mother plants of Jatropha curcas, aids to overcome problems such as harvesting time and an uneven yield, providing the opportunity to propagate proven elite genotypes. Until now, several authors have achieved SE in J. curcas using different explants, however, in none of these protocols adult plants have been used as mother plant material. Furthermore, the challenge of overcoming the morphogenesis limitations successfully and further regeneration of adult plants of J. curcas has been great. The main objective of this study was to develop a somatic embryogenesis protocol for adult plants of the oil producing J. curcas. transverse thin cell layers (tTCL) of young petioles were used as explants. Half strength Y3 and MS media were used containing three different concentrations of 2,4-dichloropherioxyacetic acid and 6-benzil-aminopurine in a factorial trial. Nine accessions of J. curcas of various ages (3-12 years.) were tested using this protocol. All accessions responded positively to callus induction and to the induction of somatic embryos, demonstrating the protocol to be genotype-independent. This study enables micropropagation of adult of proven elite plants of J. curcas via somatic embryogenesis.
Key words: Jatropha curcas, transverse thin cell layers (tTCL), somatic embryos, half strength media, regeneration.
Abbreviation:
2,4-D, 2,4-dichlorophenoxyacetic acid; BAP, 6-Benzyl-aminopurine; IAA, indole-3-acetic acid; Kinetin, 6-furfuryl aminopurine; MS, Murashige and Skoog medium; Picloram, 4-amino-3,5,6-trichloro-2-pyridinecarboxylic acid; TCL, thin cell layer; Y3, Y3 minerals medium.
In a world of an increasing demand for energy, fossil fuels are becoming more scarce and less favourable due to their CO2 emissions and impact on global warming (Rehman et al., 2019). The focus on renewable energies has identified Jatropha curcas L. as a promising plant to contribute to a more sustainable alternative. This is due to the yield and quality of the oil extracted from the seeds, having a relatively high oil content, ranging from ~30% to ~35% (Barros et al., 2015; de Oliveira et al., 2009).
Nevertheless, even though J. curcas is highly promising as a renewable energy source, some challenges have been encountered whilst attempting to exploit the crop (Mulpuri et al., 2019). The plant is still a wild type plant, that is not domesticated yet and this presents high heterogeneity between individuals within cultivars (Bahadur et al., 2012). Consequently, agronomical and harvesting processes are challenging (Mulpuri et al., 2019). In order to minimize and overcome these challenges, the selection and mass propagation of elite genotypes has become an encouraging solution (Mulpuri et al., 2019).
To achieve mass propagation, the somatic embryogenesis (SE) technique was chosen due to its high amount of plants obtained per explant, considering that, the plants regenerated from this technique are mostly non-chimeric and the populations derived are true clones (Egertsdotter et al., 2019). However, adult plants are highly recalcitrant to tissue culture (Jyoti et al., 1998).
To achieve SE in J. curcas, different explants have been used: embryo axis and cotyledons (Nindita et al., 2014); root and hypocotyl (Galaz-Ávalos et al., 2012); leaf and shoot tips (Medipally et al., 2014); young leaves from young plants (Baran Jha et al., 2007); and immature zygotic embryos (Cai et al., 2011). However, there are no reports on SE protocols using transverse Thin Cell Layers (tTCL) in adult plants of J. curcas. TCL explants are small pieces of somatic tissue that usually consist of different types of cells (parenchyma, epidermal, cambium, vascular and medullar tissues) (Van, 1980). As explained by Nhut et al. (2003), TCLs are very responsive and the morphogenic responses are dependent on different factors, also involving growth conditions (PGRs, light quality, temperature, photoperiod).
The aim of this paper was to develop an efficient protocol to induce SE in adult plants of J. curcas, to obtain successful micropropagation of elite genotypes, using transverse Thin Cell Layers of young petioles.
Nine accessions of J. curcas L. were used, with an age range of 4 to 12 years from different countries (Table 1) where the plant is widely cultivated and economically important. The reason for the wide location range and age span was to develop a robust genotype-independent micropropagation protocol, which could be applied to elite material.
Mother plants growing in two different conditions, greenhouse and field, were used. Second and third leaves were excised from apical buds in active growth (Figure 1). The leaves were rinsed with Milli-Q water, and subsequently, the petioles were separated. The excised petioles were washed in 70% alcohol for 1 min. For the plants derived from the field, 2 g of disinfectant soap was used for 2 min, as an extra step. The petioles were washed with Milli-Q water for 1 min and placed into a laminar flow chamber. Petioles were surface sterilized with a solution of 0.5% sodium hypochlorite (Sigma-Aldrich 10-14% W/W) with 0.02 % of Tween® 20 for 7 min. The field plants were additionally treated with a fungicide solution (Carbendazim 2% (Sigma-Aldrich 97%) + 2g Tween® 20), before surface sterilization with sodium hypochlorite. The petioles were then rinsed three times ( at 5, 10 and 20min) with autoclaved double distilled water. Both ends of the petioles were removed and the middle part was sectioned using a sterile scalpel to obtain the tTCLs. Subsequently, the tTCLs were used as explants to induce somatic embryogenesis (Figure 1). In addition, different types of explants were used, that is, leaf discs, petiole segments, longitudinal and transverse TCL of leaf and petiole.
Two basal media, Y3 salts and vitamins (Eeuwens, 1976) and MS salts and vitamins (Murashige and Skoog, 1962) at full and half strength, were tested. Both media were supplemented with 30 g.L-1 sucrose, 100 mg.L-1 Myo-inositol, 100 mg.L-1 arginine, 100 mg.L-1 asparagine, 100 mg.L-1 L-glutamine and jellified with 3.8 g.L-1 of Gelrite™ (DUCHEFA Biochemie B.V, Netherlands). The pH was adjusted to 5.7 ± 0.1, prior to autoclaving for 15 min at 121°C. The growth chamber conditions for all the experiments were darkness and temperature 26 ± 1°C. Light conditions were only used for the maturation of embryos.
The two basal media, were supplemented with different concentrations of the auxins 2,4-D, indole-3-acetic acid (IAA), Picloram, the cytokines 6-Benzyl-aminopurine (BAP) and Kinetin, alone and in combinations. When alone, the hormones were added in a decreasing gradient of 0.5 from 5 to 0 mg.L-1, and when in combination, in a decreasing gradient of 0.5 from 2.5 to 0 mg.L-1. 30 ml of culture medium was poured post-autoclaving into polystyrene Petri dishes (9 cm). Seven tTCLs from petioles were inoculated per Petri dish, and the Petri dish was sealed with Parafilm before being placed in a growth chamber.
After 4 weeks of initial inoculation, the explants with developed callus were transferred onto callus multiplication medium consisting of the two basal media supplemented with 0.05mg.L-1 of 2,4-D and 2 mg.L-1 BAP. The Petri dishes were sealed and placed in the growth chamber.
Two weeks after multiplication, to induce SE, calli were transferred onto regeneration media. The regeneration media consisted of the two basal media, supplemented with 2mg.L-1 of BAP. Petri dishes containing the callus were sealed with Parafilm and kept in the growth chamber.
After 2 weeks in the induction phase, the calli showing embryo structures were transferred to both basal media containing 2 mg.L-1 of BAP. The Petri dishes were kept in the growth chamber for further two weeks.
For the maturation of the embryos, they were transferred to both basal media, containing 2 mg.L-1 of BAP, and the Petri dishes were moved to a growth chamber with 26 ± 1°C with a photoperiod of 16 h light/8 h darkness and an irradiance of ± 40 µMol.m-2 s-1.
In order to overcome the recalcitrance, characteristic of adult plants of
J. curcas for micropropagation (Jyoti et al., 1998; Nhut et al., 2013), it was sought to develop a protocol to induce
SE using different types of explants from adult mother plants. Several types of explants were tested, and it was found that tTCLs of young petioles were the most suitable explants (Figure 2B). As shown in Figure 2A, tTCL of the petiole contains a small number of cells of different types, which means that this type of explant includes a new and greater number of patterns for morphogenesis compared to those present in other somatic cell systems (Gendy et al., 1996).
Contamination was not observed in the plant material from the greenhouse and in less than 10% of the plant material coming from the field. Thus, the methodology used for partial surface sterilization and disinfestation on both plant materials was efficient, allowing the in vitro establishment of the selected accessions.
In this study, the results indicate that half-strength Y3 medium supplemented with 0.5 mg.L-1 of 2,4-D and 0.5 mg.L-1 of BAP gave the best results for callus induction (100%) in all accessions and more embryogenic cells compared to the other treatments (Figure 3). The other explants either died or the cells were hyper hydrated. To induce somatic embryos, Medipally et al. (2014)used the same concentration and type of hormones as were used in this paper, but with very young plants (9-months-old) and using leaf lamina and shoot tips as explant. For this protocol, responses were obtained from adult plants using petioles. Calli showed granular/nodular aspect, were friable and of a light green/yellow colouration. The cells were rounded and obtained seven days after first inoculation (Figure 2D).
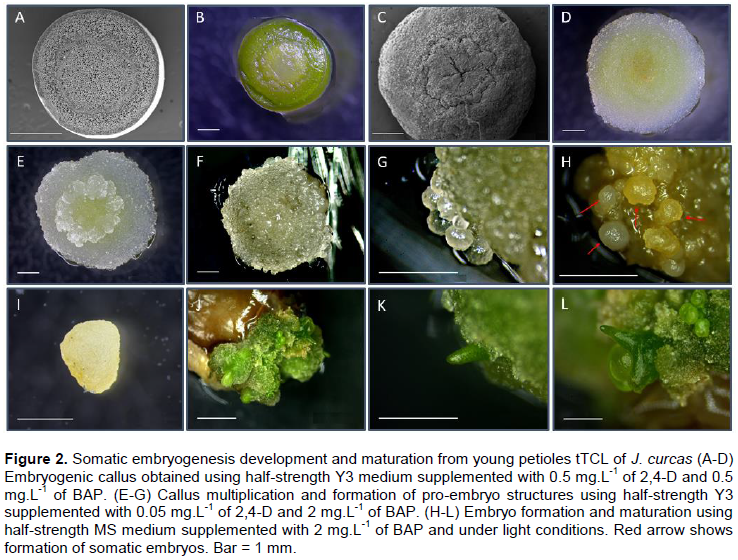
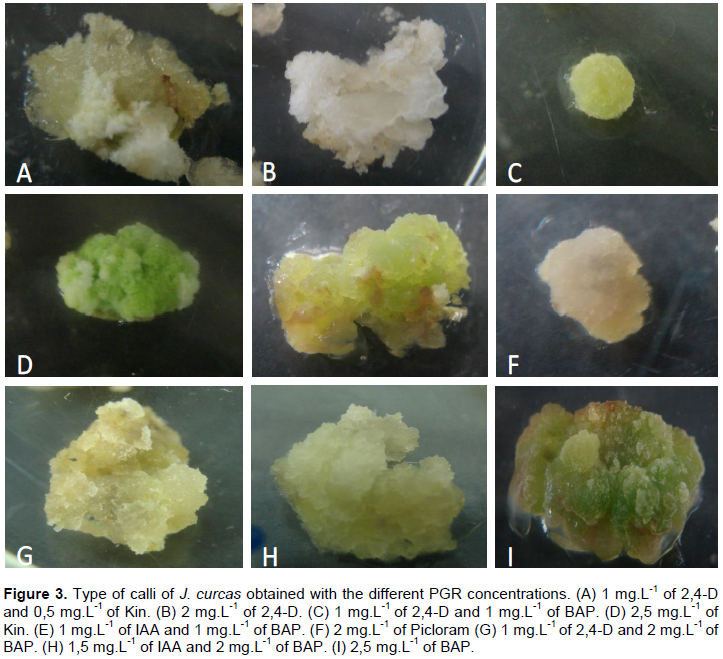
It could be observed that callus started developing on the central part of the petiole TCL and continued all over the explant (Figure 2C). Moreover, Nhut et al. (2013) described a protocol for propagating J. curcas through direct and indirect SE and Organogenesis using tTCL. However, they also performed it on very young plants and used leaves lamina, while in this study, adult plants were used given the advantage that the protocol can be used for elite genotypes. Even though in a more recent study (Galaz-Ávalos et al., 2018), SE was obtained using relatively low concentrations of exogenous hormones. The time for each stage (seed germination, plantlet preparation, individualisation of explant, embryo induction, etc.) was extremely long (≥100 days before first response was seen), whilst in this study, somatic embryos were obtained before 45 days after first inoculation and from in vivo explants.
The four-week-old calli were then transferred to multiplication media containing 0.05 mg. L-1 of 2,4-D and 2 mg.L-1 of BAP. Shortly after, callus cells started to multiply rapidly and form small clumps, mostly on the edges of the callus as well as on the central part (Figure 2E). It could be observed that the cells started growing and forming globular structures on the edges of the callus 45 days after first inoculation (Figure 2F-G), which was also recorded in carrot (Masuda et al., 1995), J. curcas (Kalimuthu et al., 2007), Coffea arabica (Bartos et al., 2018) and macaw palm (Meira et al., 2019).
For this experiment, the embryogenic cells were obtained only when both 2,4-D and BAP were present in the culture media. The importance of the interaction between auxins and cytokinins has been well studied and described previously (Litz and Gray, 1995) to improve cell organisation in morphogenetic processes (Fujimura and Komamine, 1980). Baran Jha et al. (2007) obtained embryos when the embryogenic calli were transferred onto MS supplemented with 0.5 mg.L-1 Kinetin and 0.25 mg.L-1 IBA, also proving the importance of auxin-cytokinin interaction.
Once the exogenous auxin 2,4-D was completely removed, and 2 mg.L-1 of BAP was kept in the medium, embryos started to form 55 days after inoculation (Figure 2H). Medium free of added auxins induces a reduction in the level of endogenous auxins in the explant, which has triggered somatic cells in the callus to start differentiation processes and also leads to the maturation of the embryos (Ribnicky et al., 1996). The somatic embryos in this protocol presented a white/yellow colouration and could be easily separated. (Figure 2I). Several authors have shown that callus growing in medium with 2,4-D, and thereafter, complete removal of the hormone has induced SE (Cheema, 1989; Godbole et al., 2002; Seldimirova et al., 2019). Kalimuthu et al. (2007) managed to induce direct somatic embryos from green cotyledons using 2 mg.L-1 of BAP. In this study, when the embryos were moved onto half-strength MS medium, supplemented with 2 mg.L-1 of BAP under a photoperiod of 16h/8h, the embryos started to mature and advance into further stages after 85 days after inoculation (Figure 2J-L). In accordance with previous publications, BAP showed higher responsiveness on the somatic embryos, for maturation (Agarwal and Kamal, 2004; Cai et al., 2011; Kumar et al., 1994) and germination (Payghamzadeh and Kazemitabar, 2012; Raemakers et al., 1993; Siang et al., 2012).
Up to the date of submission of this study, there has not been a report on the importance of shifting between different basal media for inducing somatic embryo-genesis. However, there are many reports describing the importance of media composition on stimulating subsequent stages of SE, as well as for the optimisation of the morphogenetic response (Kumar et al., 2008; Pinto et al., 2008; Rodríguez-Sahagún et al., 2011; Walker and Sato, 1981).
As far as we are aware, this is the first report on the successful induction of somatic embryogenesis from several accessions of adult plants of J. curcas L., using tTCL of petioles. The fast and high response from the type of explant and the developmental stage of the mother plants make this protocol a great tool for inducing somatic embryogenesis from elite genotypes of J. curcas. This protocol also provides a great opportunity for plant breeders to develop improved varieties.
The authors have not declared any conflict of interests.
This work was supported by the Norwegian Agency for Development Cooperation (NORAD) funded project “Research and Capacity Building in Clean and Renewable Bioenergy in Ethiopia” under the Norwegian Programme for Capacity Development in Higher Education and Research for Development (NORHED/ EnPe) Program, coordinated by Dr. Meseret Tesema of Hawassa University, Ethiopia and Norwegian University of Life Sciences (NMBU), Norway. The authors acknowledge a short stay in Prof. Lucia Atehortua’s lab in Colombia.
2,4-D, 2,4-dichlorophenoxyacetic acid;
BAP, 6-Benzyl-aminopurine;
IAA, indole-3-acetic acid;
Kinetin, 6-furfuryl aminopurine;
MS, Murashige and Skoog medium;
Picloram, 4-amino-3,5,6-trichloro-2-pyridinecarboxylic acid;
TCL, thin cell layer;
Y3, Y3 minerals medium.
REFERENCES
Agarwal M, Kamal R (2004). In vitro clonal propagation of Momordica charantia L. Indian Journal of Biotechnology 3:426-430.
|
|
Bahadur B, Sujatha M, Carels N (2012). Jatropha, Challenges for a New Energy Crop . Genetic Improvement and Biotechnology 2:139-142.
Crossref
|
|
|
Baran Jha T, Mukherjee P, Datta MM (2007). Somatic embryogenesis in Jatropha curcas Linn., an important biofuel plant. Plant Biotechnology Reports 1:135-140.
Crossref
|
|
|
Barros TFS, Arriel NHC, Queiroz MF, Fernandes PD, Mendonça S, Ribeiro JAA, Medeiros EP (2015). Fatty acid profiles of species of Jatropha curcas L., Jatropha mollissima (Pohl) Baill. and Jatropha gossypiifolia L. Industrial Crops and Products 73:106-108.
Crossref
|
|
|
Bartos PMC, Gomes HT, Gomes SM, Vasconcelos Filho SC, Teixeira JB, Scherwinski-Pereira JE. (2018). Histology of somatic embryogenesis in Coffea arabica L. Biologia 73:1255-1265.
Crossref
|
|
|
Cai L, Fu L, Ji L (2011). Regeneration of Jatropha curcas through efficient somatic embryogenesis and suspension culture. GM Crops 2(2):110-117.
Crossref
|
|
|
Cheema GS (1989). Somatic embryogenesis and plant regeneration from cell suspension and tissue cultures of mature himalayan popular (Populus ciliata). Plant Cell Reports 8(2):124-127.
Crossref
|
|
|
de Oliveira JS, Leite PM, de Souza LB, Mello VM., Silva EC, Rubim JC, Suarez PA (2009). Characteristics and composition of Jatropha gossypiifoliaand and Jatropha curcas L. oils and application for biodiesel production. Biomass and Bioenergy 33(3):449-453.
Crossref
|
|
|
Eeuwens C (1976). Mineral requirements for growth and callus initiation of tissue explants excised from mature coconut palms (Cocos nucifera) and cultured in vitro. Physiologia Plantarum 36(1):23-28.
Crossref
|
|
|
Egertsdotter U, Ahmad I, Clapham D (2019). Automation and Scale Up of Somatic Embryogenesis for Commercial Plant Production, With Emphasis on Conifers. Frontiers in Plant Science 10(109): 109.
Crossref
|
|
|
Fujimura T, Komamine A (1980). Mode of action of 2, 4-D and zeatin on somatic embryogenesis in a carrot cell suspension culture. Zeitschrift für Pflanzenphysiologie 99(1):1-8.
Crossref
|
|
|
Galaz-Ávalos RM, Avilez-Montalvo RN, Ucan-Uc C M, Chan-López JA, Loyola-Vargas VM (2012). Jatropha curcas una alternativa para la obtención de biodiésel sin afectar al sector alimentario. Biotecnología 16:94-114.
|
|
|
Galaz-Ávalos RM, Martínez-Sánchez HG, Loyola-Vargas VM (2018). Induction of Somatic Embryogenesis in Jatropha curcas. In: Plant Cell Culture Protocols 207-214.
Crossref
|
|
|
Gendy C, Sene M, Van Le B, Vidal J,Thanh KVT (1996). Somatic embryogenesis and plant regeneration in Sorghum bicolor (L.) Moench. Plant Cell Reports 15(12):900-904.
Crossref
|
|
|
Godbole S, Sood A, Thakur R, Sharma M, Ahuja PS (2002). Somatic embryogenesis and its conversion into plantlets in a multipurpose bamboo, Dendrocalamus hamiltonii Nees et Arn. Ex Munro. Current Science 885-889.
|
|
|
Jyoti S, Amla B, Renu S (1998). In vitro plantlet formation and micropropagation of Jatropha curcas (L.). Advances in Plant Sciences 11(2):167-169.
|
|
|
Kalimuthu K, Paulsamy S, Senthilkumar R, Sathya M (2007). In vitro propagation of the biodiesel plant Jatropha curcas L. Plant Tissue Culture and Biotechnology 17(2):137-147.
Crossref
|
|
|
Kumar JV, Kumari BR, Sujatha G, Castano E (2008). Production of plants resistant to Alternaria carthami via organogenesis and somatic embryogenesis of safflower cv. NARI-6 treated with fungal culture filtrates. Plant Cell, Tissue and Organ Culture 93(1):85-96.
Crossref
|
|
|
Kumar VD, Kirti PB, Sachan JKS, Chopra VL (1994). Plant regeneration via somatic embryogenesis in chickpea (Cicer arietinum L.). Plant Cell Reports 13(8):468-472.
Crossref
|
|
|
Litz RE, Gray DJ (1995). Somatic embryogenesis for agricultural improvement. World Journal of Microbiology and Biotechnology 11(4):416-425.
Crossref
|
|
|
Masuda H, Oohashi SI., Tokuji Y, Mizue Y (1995). Direct embryo formation from epidermal cells of carrot hypocotyls. Journal of Plant Physiology 145(4):531-534.
Crossref
|
|
|
Medipally SR, Naresh B, Manoj Kumar S, Yusoff FM, Devi P (2014). Somatic Embryogenesis from leaf and shoot tip explants of Jatropha curcas L.. Indian Journal of Science and Technology 7:1842-1846.
Crossref
|
|
|
Meira FS, Luis ZG, de Araújo Silva-Cardoso IM,Scherwinski-Pereira JE (2019). Developmental pathway of somatic embryogenesis from leaf tissues of macaw palm (Acrocomia aculeata) revealed by histological events. Flora 250:59-67.
Crossref
|
|
|
Mulpuri S, Carels N, Bahadur B (2019). Jatropha, Challenges for a New Energy Crop : A Sustainable Multipurpose Crop 3:4-23.
Crossref
|
|
|
Murashige T, Skoog F (1962). A revised medium for rapid growth and bio assays with tobacco tissue cultures. Physiologia Plantarum 15(3):473-497.
Crossref
|
|
|
Nhut DT, da Silva JAT, Van Le B, Van K.TT (2003). Thin cell layer (TCL) morphogenesis as a powerful tool in woody plant and fruit crop micropropagation and biotechnology, floral genetics and genetic transformation. Micropropagation of Woody Trees and Fruits 783-814.
Crossref
|
|
|
Nhut DT, Hai NT, Huyen PX, Huong DTQ, Hang NTT, da Silva JAT (2005). Thidiazuron induces high frequency shoot bud formation from Begonia petiole transverse thin cell layer culture. Propagation of Ornamental Plants 5(3):149-155.
|
|
|
Nhut DT, Loan NTK, Huy NP, Hai NT, Chien HX, Tuan TT, DuTX(2013). Thin Cell Layer Technology in Micropropagation of Jatropha curcas L. In Biotechnology of Neglected and Underutilized Crops, pp. 33-42.
Crossref
|
|
|
Nindita A, Purwoko BS, Efendi D, Dewi IS (2014). Somatic embryogenesis media optimization study of physic nut (Jatropha curcas) as biodiesel feedstock. Energy Procedia 47:21-28.
Crossref
|
|
|
Payghamzadeh K, Kazemitabar S (2012). The effects of BAP, IBA and genotypes on in vitro germination of immature walnut embryos. International Journal of Plant Production 4(4):309-322.
|
|
|
Pinto G, Silva S, Park YS, Neves L, Araújo C, Santos C (2008). Factors influencing somatic embryogenesis induction in Eucalyptus globulus Labill.: basal medium and anti-browning agents. Plant Cell, Tissue and Organ Culture 95(1):79-88.
Crossref
|
|
|
Raemakers C, Bessembinder J, Staritsky G, Jacobsen E, Visser R (1993). Induction, germination and shoot development of somatic embryos in cassava. Plant Cell, Tissue and Organ Culture 33(2):151-156.
Crossref
|
|
|
Rehman A, Rauf A, Ahmad M, Chandio AA, Deyuan Z (2019). The effect of carbon dioxide emission and the consumption of electrical energy, fossil fuel energy, and renewable energy, on economic performance: evidence from Pakistan. Environmental Science and Pollution Research 26(21):21760-21773.
Crossref
|
|
|
Ribnicky DM, Ilic N, Cohen JD, Cooke TJ (1996). The Effects of Exogenous Auxins on Endogenous Indole-3-Acetic Acid Metabolism (The Implications for Carrot Somatic Embryogenesis). Plant Physiology 112(2):549-558.
Crossref
|
|
|
Rodríguez-Sahagún A, Acevedo-Hernández G, Rodríguez-Domínguez JM, Rodríguez-Garay B, Cervantes-Martínez J, Castellanos-Hernández OA (2011). Effect of light quality and culture medium on somatic embryogenesis of Agave tequilana Weber var. Azul. Plant Cell, Tissue and Organ Culture 104(2):271-275.
Crossref
|
|
|
Seldimirova O, Kudoyarova G, Kruglova N, Galin I, Veselov D (2019). Somatic Embryogenesis in Wheat and Barley Calli in vitro Is Determined by the Level of Indoleacetic and Abscisic Acids. Russian Journal of Developmental Biology 50(3):124-135.
Crossref
|
|
|
Siang TC, Soong ST,Yien ATS (2012). Plant regeneration studies of Jatropha curcas using induced embryogenic callus from cotyledon explants. African Journal of Biotechnology 11(31):8022-8031.
Crossref
|
|
|
Van KTT (1980). Control of morphogenesis by inherent and exogenously applied factors. in thin cell layers [Plants, de novo buds, roots]. International Review of Cytology 11:175-194.
|
|
|
Walker K, Sato S (1981). Morphogenesis in callus tissue of Medicago sativa: the role of ammonium ion in somatic embryogenesis. Plant Cell, Tissue and Organ Culture 1(1):109-121.
Crossref
|
|