In Ivory Coast, the continuous accumulation of municipal solid waste from different sources causes an undesirable enrichment of heavy metals or metalloids in dumpsite areas (Bongoua-Devisme et al., 2018a, b). This is the case for the dumpsite soil of M’Plouessoue Park, where the soils are contaminated with heavy metals such chromium (Cr), lead (Pb) and nickel (Ni) (Bongoua- Devisme et al., 2018a, b). These potential toxic elements
(PTEs) can enter the soil and groundwater resources and, consequently, pose severe threats to the food chain, human health and soil ecosystems. Thus, it is necessary to develop a remedial approach to remove heavy metals from contaminated soils. One of the established approaches is through phytoremediation strategies.
Phytoremediation is a new technology that utilizes plant to extract heavy metals from the contaminated ground, accumulating these metals in roots, stems and branches. Many studies have been conducted in this field over the last two decades, and various phytoremediation approaches (phytostabilization, phytoimmobilization and phytoextraction) have been successfully performed (Favas et al., 2019). However, the success of this new technology depends on the plant species, which must be able to tolerate and accumulate high concentrations of metals in shoots, undergo rapid growth and show high biomass production potential (Mohd et al., 2013).
Acacia mangium Wild. is a tropical plant which has the capacity to improve soil fertility by enhancing nutrient cycling, higher nutrient availability and microbial activities (Koutika, 2019). Moreover, this plant is rapidly growing and developing very fast. They can also grow in extreme conditions. It is also plant owing to its remarkable capacity to extract metal from polluted soils. The phytoremediation potential of A. mangium was been previously reported in numerous studies (Majid et al., 2012; Mohd et al., 2013). In fact, Majid et al. (2012) demonstrated that A. mangium can accumulate 93.5 mg kg-1 of copper (Cu) and 79 mg kg-1 of zinc (Zn) in its biomass and was able to tolerate high concentration of cadmium (Cd). Mohd et al. (2013) reported that A. mangium requires 5 and 17 years to remove 79.8 kgha-1 of Zn and 47 kgha-1 of Cu, respectively.
In contrast, many works have demonstrated that the success of phytoremediation may not solely depend on the plant itself but also on the interaction of plant roots with soil microorganism and fauna and the amount of heavy metals accumulated soil, because the interaction between plants and beneficial rhizosphere micro-organisms can enhance biomass production and the tolerance of plants to heavy metals (Ma et al., 2015).
Hence, another promising approach that has recently been employed to improve plant growth is the application of soil fauna such as earthworms. Earthworms are generally described as ecosystem engineers that greatly impact the physical, chemical and biological properties of soil by affecting the availability of nutrients and organic compounds for other organisms, and significantly increasing plant production (Blouin et al., 2013).
Pontoscolex corethrurus, an endogeic earthworm is one of the most widespread peregrine earthworms which is commonly found in humid tropics (Taheri et al., 2018; De Novais et al., 2018). It shows a very broad tolerance to various environmental conditions (Taheri et al., 2018). It is used in ecotoxicological studies and has also been recommended as a bioindicator for the assessment of soil quality and ecosystem disturbances (Taheri et al., 2018). For instance, it was used for the remediation of polluted sites (Duarte et al., 2014) and the improvement of phytoextraction treatments (Jusselme et al., 2015). Moreover, many studies have demonstrated that P. corethrurus species offers a more relevant alternative than the current use of Eisenia fetida or Eisenia andrei for ecotoxicological tests (Buch et al., 2013).
Thus, it is of interest to study the conjugated actions of P. corethrurus earthworm and of a metal tolerant plant such as A. mangium in the remediation of metal-contaminated soil. The principal aims of this research were to evaluate the effects of P. corethrurus on Pb, Cr and Ni phytoremediation efficiency of A. mangium in contaminated soil.
Soil sampling and analysis
The contaminated soils were sampled from the abandoned dumping site located in M’Ploussoue Park, Bonoua, Ivory Coast, at latitude 5°16' N and longitude 3°36' W, where the potential toxic elements (PTEs) concentration exceeded environmental law screening values (Bongoua-Devisme et al., 2018a,b). Soil samples were collected at 18 different points from the surface (0-30 cm) to cover the entire study area according to the random sampling technique. Non-contaminated soil (control) situated at 5 km of contaminated site at latitude 5°15' N and longitude 3°35' W was used as a control medium. The characteristics of contaminated soil and non-contaminated soil are described in Table 1.
In each site, soil samples were air-dried and sieved to 2 mm to remove various types of wastes (paper, used batteries, electronic goods, wood, plastic paper, straws, buckets, tin cans, sacks, clothes, glass bottles, cotton wool, food wastes, leaves, fruit wastes, medicine bottles, foams, ashes, water sachet, card board and human excreta), then were mixed and homogenized to obtain a composite sample. The composite sample was transferred to the laboratory for various analyses. The composite sample was also used for a pot experiment.
Biological material
Seeds of A. mangium were obtained from the Centre National of Research Agronomy (CNRA) at Oume, Ivory Coast. Seeds were treated with concentrated sulfuric acid (95%) before pregermination, as described by Diouf et al. (2005). The treated seeds were pregerminated in a Petri dish containing 0.8% water-agar medium (w/v) and sterilized for 30 min at 110°C. Then, the Petri dish was stored at room temperature (30°C) in the dark for 72 h, after packing with aluminium paper. Before pregermination in the Petri dish, three pregerminated seedlings were transplanted into polyethylene plastic nursery bags (15 × 40 × 150 cm) filled with contaminated and non-contaminated soil sieved at 2 mm. One month after the transplantation in the plastic nursery bags, seedlings of uniform size were individually transferred into perforated pots filled with 5 kg of dry soil sieved at 2 mm.
Earthworms (P. corethrurus) were hand-collected from Felix Houphouet-Boigny University, Cocody, Abidjan, Ivory Coast, which had not been contaminated with trace metal elements. The earthworms were then kept in plastic boxes filled with water for one week to monitor their health before starting the experiment. For the treatment with earthworms, five adult earthworms (±5 g) were placed in the perforated pot after transplantation of the seedling.
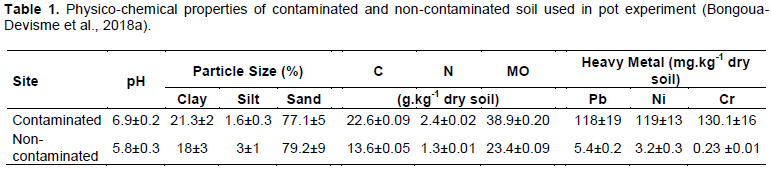
Experimental design
A greenhouse pot culture experiment was conducted at Felix Houphouet-Boigny University, Cocody, Abidjan, Ivory Coast to study the effect of the P. corethrurus earthworms on the growth and phytoremediation capacity of A. mangium. The average temperatures in the greenhouse were 26, 38 and 32°C for morning, afternoon and evening, respectively. The experiment was carried out using four treatments:
(1) non contaminated soil-non inoculated, control (C);
(2) non contaminated soil-inoculated (NcI);
(3) contaminated soil-non inoculated (CNi);
(4) Contaminated soil-Inoculated (CI).
The experiment was conducted for 90 days, and each treatment was carried out in triplicate. Before filling the pot with the soil, it was perforated to allow aeration and then covered with a perforated net to prevent the earthworms from escaping. The pots were placed in a factorial arrangement based on a completely randomized block design. The seedlings were watered daily with deionized water to maintain the moisture content at approximately 60% water-holding capacity of the soil.
Plant harvest and analysis
At the end of the experiment (90 days), plant in the pot for each treatment was removed. The rhizosphere soil (RS), which was defined as the soil that remained attached to the roots after gentle shaking (Tan et al., 2017) and the drilosphere soil (DS), which was the portion of soil influenced by earthworm secretions, burrowing and castings, were collected. Growth parameters such as shoot length, fresh weight and dry weight of the plants were measured. The height of Acacia was measured for each treatment and each replicate. Shoots (leaves and stems) were harvested, and roots were carefully removed from the soil, rinsed with tap water, and washed three times with deionized water; nodules were detached and counted. The fresh weight was determined for each plant part (shoots and roots) and then the plant part was dried at 60°C for 72 h, weighed and stored for analysis. The total dry weight of biomass (shoots + roots) of each plant per pot was determined. Rhizobial infection was evaluated by counting the number of nodules per plant. All the different soil compartments were air-dried and stored prior to the analyses. The earthworms were hand-collected, counted and weighed. The PTEs such as Ni, Cr and Pb concentrations in plant shoots (leaves and stems) and roots were dosed but for the different soil compartments (RS and DS) only their concentrations were determined in the contaminated soil treatments, using an inductively coupled plasma atomic emission spectrometer (ICP-AES).
The ability of the plant to accumulate metals from the soil and transfer metals from the roots to the shoots was estimated by the bioconcentration factor (BCF) and translocation factor (TF), respectively, as described by Majid et al. (2012). BCF is the ratio of the metal concentration in the shoots of plants to that in the soil. TF is the ratio of the metal concentration in the shoots to that in the roots of plants.
Bioconcentration factor (BCF):
Statistical analysis The data were subjected to statistical analysis using 7.1 Statistica software. Significant differences between different treatments (non contaminated soil-non inoculated, control, (C); non contaminated soil-inoculated (NcI); contaminated soil-non inoculated (CNi); contaminated soil-inoculated (CI)) in terms of height, biomass production, nodule numbers, and heavy metal contents in plant biomass, shoot tissue, root tissue and different compartments RS and DS were performed using the Student-Newman-Keuls (SNK) test at 0.05 probability level.
Plant growth performance under different treatments
Throughout the experimental period (90 days), regardless
of treatments applied, no visible heavy metal morphological toxicity symptoms, such as leaf chlorosis and root browning, appeared when Acacia was planted in contaminated soil under greenhouse conditions (Figure 1). This result revealed that Acacia is able to grow in metal-contaminated soils and is a metal-tolerant plant species, as suggested by Majid et al. (2012).
The significantly (P < 0.05) lowest height (Figure 2a), total dry weight biomass (Figure 2b) and nodule number (Figure 2c) were obtained under the contaminated soil-non inoculated (CNi) treatment, with 25.7 cm, 31 g and 5 nodules/plant, respectively (Figure 2). However, the presence of the earthworms resulted in a significant (P<0.05) increase in height (ranging from 25.7 to 51.5 cm), total dry biomass weight (ranging from 31 to 101 g) and nodule numbers (ranging from 5 to 9 nodules/plant) of the plant under CI treatments (Figure 2). Furthermore, the same increase in height (ranging from 40.5 to 55.3 cm), total dry biomass weight (ranging from 89 to 125 g) and nodule numbers (ranging from 19 to 100 nodules/plant) was noted under non-contaminated soil in the presence of earthworm (NcI) (Figure 2). This phenomenon was probably due to the action of
P. corethrurus earthworms, which can improve the condition of the contaminated soil, enhance nutrient cycling, increase soil nutrient availability, and as a result, facilitate plant growth and biomass production. Furthermore, most previous studies have justified this finding by the fact that earthworms have the potential to modify edaphic parameters such as soil structure, organic matter decomposition and indirectly facilitate the uptake of many important nutrients by plant and consequently promote plant growth. In addition, the enhancement of
Acacia growth performance can be attributed to the fact that some earthworms species can decrease the content of potential toxic elements (PTEs) in metal contaminated soil through the accumulation potential toxic elements (PTEs) in their tissues and consequently promote plant growth, as demonstrated in many studies (Lemtiri et al., 2016; Sizmur et al., 2011; Boughattas et al., 2018) in the presence of different species of earthworms (
E. fetida,
Lumbricus terrestris, P. corethrurus)
. A similar finding has been documented by Jusselme et al. (2015) who showed that the presence of
P. corethrurus could enhance the biomass of
Lantana camara L. by approximately 1.5-2-fold under Pb stress.
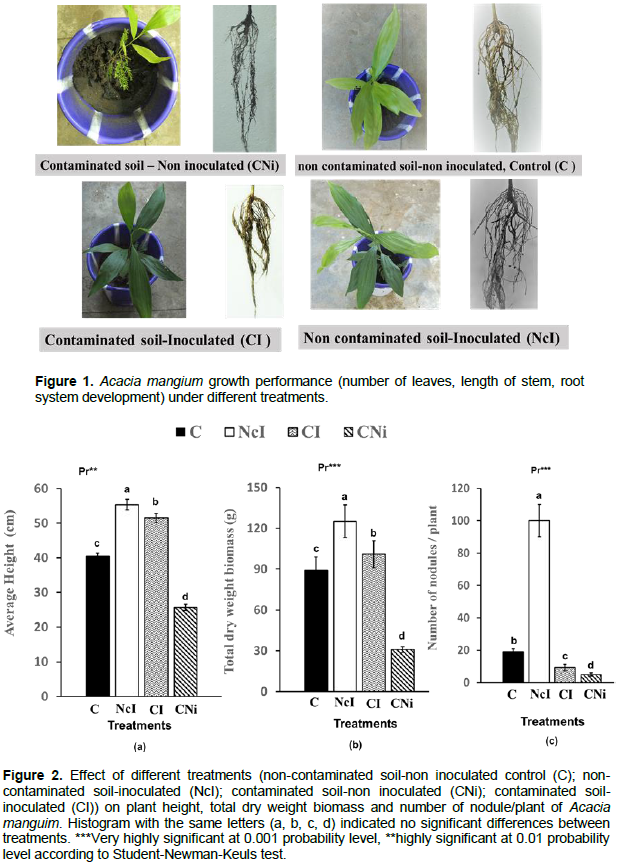
Earthworms can be used to improve phytoremediation efficiency by Acacia because of their ability to promote plant growth and biomass production and also because the morphological parameters of Acacia are not affected in contaminated soil.
Phytoremediation potential of A. mangium in contaminated soil
Cr, Ni and Pb contents are below the detection limit under non contaminated soil-non inoculated (C) and non contaminated soil-inoculated (NcI) treatments (Figure 3). In contaminated soil, the contents of Cr and Ni in plant biomass were significantly greater under inoculated (CI), with 2.4 mg.kg-1 for Cr and 2.5 mg.kg-1 for Ni, than non inoculated (CNi) treatments, with 1.33 mg.kg-1 for Cr and 2 mg.kg-1 for Ni. However, the content of Pb was lowest under inoculated (CI), with 3.4 mg.kg-1, than non inoculated (CNi) treatment, with 3.8 mg.kg-1 (Figure 3). In addition, the repartition of metal in different parts of plant tissue (root and shoot) showed that under CNi treatment, excepted for Pb content (which was 1.5-fold greater in shoot tissue (2.3 mg.kg-1) than in roots tissue (1.5 mg.kg-1)), Cr and Ni contents were very highly significant (P<0.001), 3 to 4-fold greater in roots tissue, with 1.04 mg.kg-1 for Cr and 1.6 mg.kg-1 for Ni, than in shoot tissue, with 0.3 mg.kg-1 for Cr and 0.44 mg.kg-1 for Ni (Figure 4). The results indicated that in the absence of earthworm, Acacia preferentially uptake Cr and Ni in its roots and Pb in its shoots (Figure 4). Moreover, the translocation factors ([metal]shoot/[metal]root), indicator of the effectiveness of the plant to translocate metals from roots to shoots of Acacia species, was TF < 1 for Cr and Ni, and TF > 1 for Pb under CNi treatment but under CI treatment, whatever metal dosed TF < 1 (Table 2). This emphasizes that Acacia may possess metal exclusion strategy, which probably depend to the nature of the metal. The bioconcentration factors (BCF) ([metal]plant biomass/[metal]soil) were BCF <0.1 under CNi and CI treatments (Table 2), which were indicated that Acacia are not hyperaccumulator plant as demonstrated by Cipriani et al. (2013). The findings did not differ from those of various studies indicating that Acacia is able to tolerate and uptake heavy metal in its tissues and therefore could be suitable for phytostabilization of metal-contaminated sites (Majid et al., 2012; Mohd et al., 2013). Ang et al. (2010) observed a higher accumulation of Pb in the shoots of A. mangium compared with the roots as the present finding. Furthermore, Cr, Ni and Pb phytoextraction efficiency (PEE) of A. mangium was 2, 16.9 and 9.8%, respectively, under CNi treatment (Table 2) and varied according to the nature of metal, which could be attributed to the form of the metal in the soil rhizosphere. Thus, it appeared that, according to the nature of the heavy metal in contaminated soil, Acacia could have different phytoremediation processes (phytoimmobilization and phytoextraction) when it was none inoculated with P. corethrurus. But this phytoremediation process of Acacia seems to depend on the nature and the mobile form of metal in the rhizosphere soil.
However, under inoculated treatment (CI), Cr, Ni and Pb contents were very highly significant (P<0.001), 2 to 10-fold greater in roots tissue, with 1.6 mg.kg-1 for Cr, 1.9 mg.kg-1 for Ni and 3.1 mg.kg-1 for Pb, than in shoot tissue, with 0.8, 0.63 and 0.3 mg.kg-1, respectively (Figure 4). In addition, under CI treatment, PEE >10%, irrespective of the heavy metal dosed (Table 2), with 10.4% for Cr, 48.5% for Ni and 70% for Pb. This finding indicated that the inoculation of A. mangium with earthworm significantly increase the uptake and transport of Cr, Ni and Pb from the soil environment to A. mangium biomass particularly in its roots tissue, and also its phytoextraction efficiency (PEE). This increase of potential toxic elements in Acacia biomass may have been caused by the earthworm activities, as suggested previous studies (Sizmur et al., 2011), which demonstrated an increase in the bioavailability of metal in the presence of earthworm. Earthworms influence metal bioavailability in soil through the mixing and comminution of soils and by the humic materials and detritus contained in the earthworm gut (Sizmur et al., 2011). Furthermore, similar effect has been noted by Wang et al. (2006) which reported that Pheretima species could enhance zinc (Zn) accumulation in ryegrass. Moreover, the sequestration of Cr, Ni and Pb into the root parts of A. mangium in the presence of P. corethrurus earthworms probably could be related to the plant physiological attributes, such as root structure and function and also to the fractional distribution of heavy metals in metal contaminated soil, as well as their phytoavailability. Furthermore, in the presence of P. corethrurus earthworms, the phytoimmobilization potential of uninoculated A. mangium for Cr and Ni was not modified, whereas for Pb, the phytoextraction potential of uninoculated A. mangium was modified into phytoimmobilization potential.
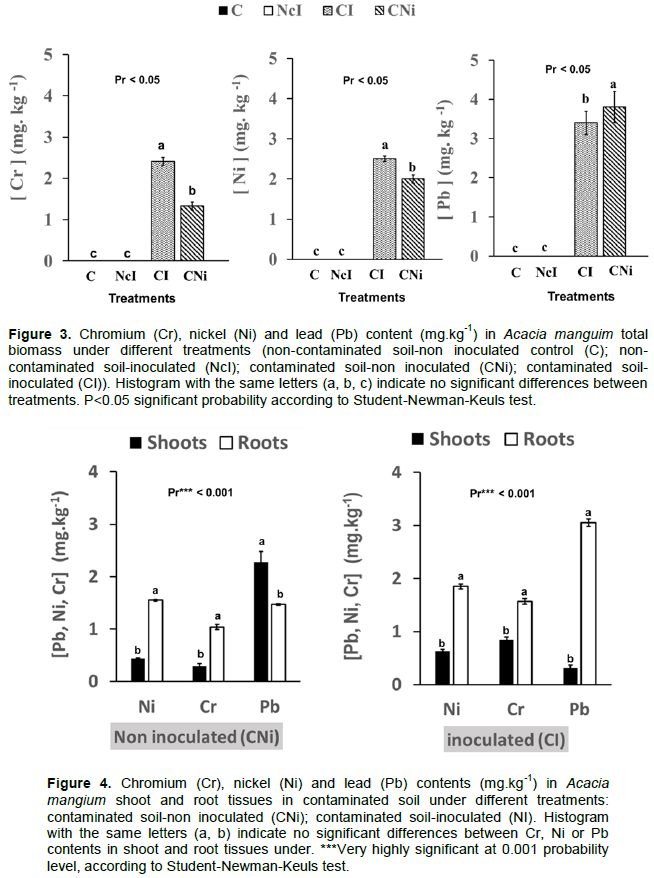
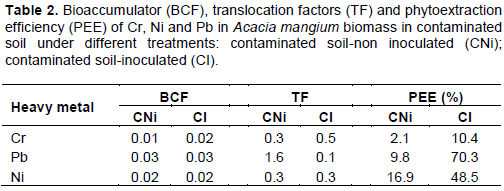
The repartition of Cr, Ni and Pb contents in the different soil compartments such as rhizosphere soil (RS) and drilosphere soil (DS) compartments, under earthworm treatment, indicated that only the content of Cr (29 mgkg-1 dry soil) was significantly (P <0.05) higher in the rhizosphere soil (RS) than in drilosphere soil (DS) compartments with 18 mgkg-1 Cr dry soil (Table 3). The highest content of Cr and Pb in the RS than in the DS compartment under earthworm treatment and the highest content of Cr (1.5 mgkg-1) and Pb (3.1 mgkg-1) in plant roots suggested that Cr and Pb mobilized by earthworm in their structures (burrows and casts) were transferred to RS compartment, which acted as sinks for these element (Brown et al., 2004), and subsequently transferred to root tissue. In contrast, despite the highest content of Ni in the DS compartment (5.6 mgkg-1 Ni dry soil) under earthworm treatment, the content of Ni was higher in the root parts than in the shoot part, which suggested that Ni mobilized in the DS compartment in their structures (burrows and casts) was stored in these structure, and temporarily transferred to RS compartment and subsequently transferred to root tissue. In addition, despite the significant accumulation of metal in RS (4.7-28.6 mg.kg-1) and DS (4.7-17.8 mg.kg-1) soil compart-ments, the content of metal in Acacia tissue was below 4 mg.kg-1. Thus, it appears that DS soil compartment was used by the plant as a sink of metal element but their content in plant tissue seems to be regulated by the plant itself, contrary to Kaur et al. (2018) studies, which demonstrated that Cd content in Brassica juncea was regulated by earthworms in the drilosphere.
This work suggested that A. mangium could have different phytoremediation process which was probably related to the form in which metal can be absorbed by the plant and also to the function of the rhizosphere.
P. corethrurus earthworm and A. mangium interaction on phytoremediation process
Previous studies have reported the interactive role of earthworms in improving plant growth in non-contaminated soils (Braga et al., 2016). In addition to this, the benefit effect of earthworms in the remediation of metal contaminated soil has been very well demonstrated in numerous research (Boughattas et al., 2017, 2018; Lv et al., 2016; Lemtiri et al., 2016), but only few studies have been conducted to assess their role in improving plant metal uptake during phytoremediation in contaminated soils (Kaur et al., 2017).
The findings of the present study have shown that the inoculation of Acacia with P. corethrurus resulted in a highly to very highly significant increase (P<0.01) in plant height, total dry weight and metal concentration in plant biomass compared with the uninoculated treatment (Table 4). Thus, Acacia appeared to exhibit rapid growth and high biomass production when earthworms were present.
This increase can been due to the interactive action between A. mangium and P. corethrurus. In fact, the character specific of the plant (Acacia) used, which is a leguminous and have the capacity to form symbiotic association with rhizosphere microorganism, such as N-fixing bacteria and arbuscular mycorrhizal fungi, and consequently influence positively plant P nutrition and growth, and then soil microbial activities (Jusselme et al., 2015), can improve the uptake of metal in contaminated soil. For Kabas et al. (2017), the increase of plant growth and metal uptake by Acacia may be due to the secretion of organic acids from root exudation. They demonstrated that some species such as Acacia spp. secrete different types and quantities of organic acids into the rhizosphere, which may enhance the mobility of metals and their uptake by roots, explaining the immobilization of Cr, Ni and Pb in plant biomass. In addition, the higher content of Cr and Pb in RS and Ni in DS than in plant biomass could be related to the physiological character of Acacia spp., which here seems to exclude a metal in its shoot tissue (Kabas et al., 2017; Majid et al., 2012; Mohd et al., 2013; Cipriani et al., 2013).
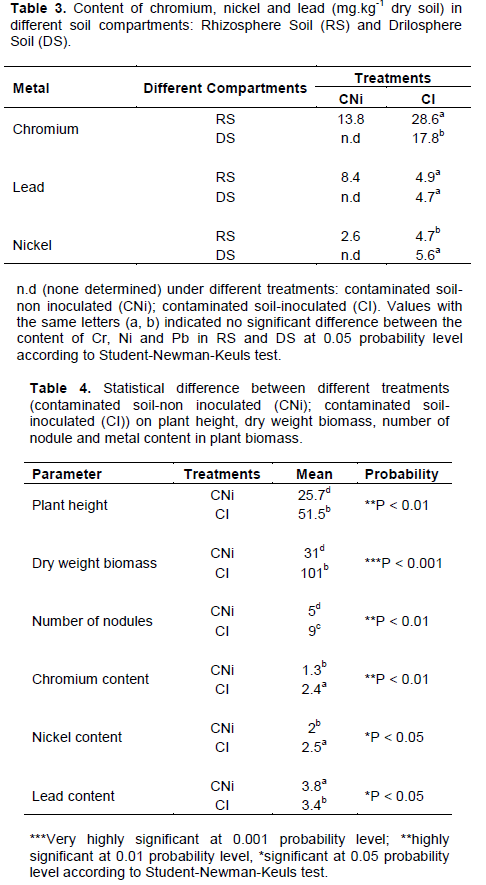
The higher significant (P<0.05) content of Pb in shoot tissue under CNi treatment, that was modified under CI, could be attributed to the form of the metal in the soil rhizosphere, which can change in the presence of earthworm. De Novais et al. (2018) justified this by the fact that the main source of organic matter used by P. corethrurus comes from the rhizosphere. So, by decomposing different types of root exudates and organic acids secreted by A. mangium into the rhizosphere, P. corethrurus can probably reduce the mobile form of metal while increase its stable form in the rhizosphere (Lemtiri et al., 2016, Boughattas et al., 2017). Boughattas et al., (2018) justified the decrease of Pb concentration in Acacia biomass under earthworm treatment by the fact that earthworm can reduced the amount of Pb associated with the soluble and exchangeable fraction.
These results suggest that, although earthworms have the potential to improve the efficiency of plant phytoremediation in metal-contaminated soils, its effectiveness depends on the nature of the plant, its behavior towards metals, rhizosphere function, and metal speciation in different soil compartments involved in the phytoremediation process.
In the presence of earthworms, the present results revealed the beneficial effects of P. corethrurus earthworms on A. mangium growth and its Pb, Ni and Cr uptake. However, the work further demonstrated that the presence of P. corethrurus earthworm affected the phytoremediation potential of A. mangium in different manners. In the control treatment, uninoculated A. mangium preferentially promoted the phytoextraction process for Pb and the phytoimmobilization process for Cr and Ni, qualifying it as a metal-tolerant plant. Furthermore, in the presence of P. corethrurus, A. mangium promoted the phytoimmobilization process for Ni, Cr and Pb. The experiments highlight the importance of biological soil parameters on the phytoremediation efficiency. It appears that earthworms have the potential to enhance the phytoremediation efficiency of plants in metal-contaminated soil but its effectiveness depends on the nature of the plant, its behavior towards metals, rhizosphere function, and metal speciation in different soil compartments involved in the phytoremediation process.