ABSTRACT
Biological extracts have recently shown a great potential for rapid biosynthesis of silver nanoparticles (AgNPs) with antibacterial activity. AgNPs were synthetized by reduction effect of secondary metabolites produced by the Nigrospora sp. fungus, from Moringa oleifera stem as a reducing agent and silver nitrate (AgNO3) (1 mM) as salt precursor. The synthesis of nanoparticles (NPs) was monitored through analysis of the UV-Vis spectroscopy absorption in the 436 to 440 nm range indicating the presence of AgNPs in the colloidal aqueous solutions. Fourier transform infrared spectroscopy (FTIR) spectra were performed to identify the compounds responsible for the bio reduction of the Ag+. The morphology and sizes of AgNPs were characterized by scanning electron microscopy (SEM), energy dispersive of X-ray spectroscopy (EDS), dynamic light scattering (DLS), and colloid stability by zeta potential measurements. The NPs obtained were spherical in shape with size in the 3 to 70 nm range. Antibacterial activity was confirmed by evaluation of their effect against Escherichia coli, Klebsiella cloacae and Staphylococcus epidermidis. The proposed green synthesis of AgNPs from secondary metabolites produced by the Nigrospora sp. fungus from M. oleifera stem can be strongly recommended as a potential method for biomedical application.
Key words: Silver nanoparticles, secondary metabolites, green chemistry, antibacterial activity.
One of the most important areas of nanotechnology is biomedicine, where metallic nanoparticles have been used for different applications such as controlled release of drugs, synthesis of new medicines, tumor cells detection, among others (Ghazwani, 2015). AgNPs are gaining particular attention due to their desirable properties like optical, magnetic, electronic, biological, catalytic and antibacterial activity. Chemical and physical methods have been used to synthetize silver nano-particles but most of these techniques are not environmentally friendly (Suarez-Cerda et al., 2015). Biological methods currently employ microorganisms, plants and their extracts (Kuppusamy et al., 2014). Many studies have proven that the plant extracts act as potential precursors for the synthesis of nanomaterials in a non-hazardous way. Plant extracts contain metabolites which act as reducing and stabilizing agents for bio-reduction of metallic NPs (Anjum and Abbasi, 2016; Kuppusamy et al., 2014). Recent reports reveal green synthesis of silver nanoparticles using primary metabolites of the plants extracts as reducing agents, for example leaf extract of Moringa (Prasad and Elumalai, 2011), extracts of Prosopis glandulosa (Abdelmoteleb et al., 2016), and of Vitex negundo plants. Antimicrobial activity against human pathogens has also been found suitable for the biosynthesis of AgNPs (Kathireswari et al., 2014). However, most of these techniques do not use secondary metabolites as reducing agents. Silver nanoparticles can acts as effective and alternative bactericide agents for combating bacterial drug resistance problems (Ahmed et al., 2015). The surface area of the NPs ensemble becomes larger as the particle size decreases, which in turn increases the total surface available for contact with bacteria, increasing the antibacterial efficiency of AgNPs (Ghazwani, 2015).
The aim of this paper was to synthesize AgNPs by the reducing effect of secondary metabolites produced by the activity of fungi and bacteria, particularly the Nigrospora sp. fungus from M. oleifera stem, in an aqueous solution of silver nitrate. The AgNPs formation was evaluated by UV-Vis, fourier transformed infrared (FTIR) spectroscopy, dynamic light scattering (DLS), scanning electron microscopy (SEM) and electron dispersive X-ray (EDS). The synthetized AgNPs exhibit antibacterial properties against different pathogenic microorganisms.
Stem and reagents/reactants
The chemicals used in this study were from Sigma (Bangalore, India) and Merck (Mumbai, India) with specifications of the American Chemical Society (ACS). M. oleifera stem samples were harvested from trees cultivated in Mexicali, Baja California, Mexico. The stem samples were previously washed with 1% v/v hypochlorite aqueous solution and distilled water to remove the dust. The sample was macerated in a mortar and dried at room temperature.
Preparation of the reductant precursors
Plant extract was prepared according to the methods of Prasad and Elumalai (2011) with some modifications. Ten grams of fresh-dried macerated M. oleifera stem in 1 L of water were heated for 20 min at 60°C to extract the active ingredients into the aqueous phase. After that, the solution was kept in incubation to permit the growing of microorganisms contained in the plant; endophytic bacteria and fungus. The main developed microorganism in the culture was the Nigrospora sp. fungus, which has been reported to produce bioactive secondary metabolites from M. oleifera stem extracts, mainly griseofulvin, dechlorogriseofulvin, 8-dihydroamulosin and mullein (Zhao et al., 2012). The culture was grown during 10 days and then the supernatant with metabolites was employed for the synthesis.
Biosynthesis of AgNPs
Working solutions with different v/v ratios of supernatant from M. oleifera stem ferments were prepared using 1 mM silver nitrate. The synthesis of AgNPs was conducted at room temperature with ratios of 1:1 (A), 1:5 (B) and 1:10 (C) between the silver nitrate and the supernatant. The reaction was carried out during 10 min. The bioreduction of Ag+ ions was observed by color change from faint yellow to yellow-brown, indicating the formation of AgNPs (Bello et al., 2015; Netala et al., 2016). The reaction mixtures were poured into a test tube and the separation was carried out using a centrifuge (10000 rpm/10 min). The supernatant was extracted with a micropipette and the pellet was suspended in 10 mL acetone and then in distilled water. This centrifugation and resuspension processes were repeated 3 to 4 times. The resulting AgNPs were air dried to evaporate excessive liquid and these were used for further characterization.
Characterization of AgNPs
The progress of reaction was followed spectrophotometrically using a Perkin-Elmer UV-Vis Lambda 25 spectrophotometer. UV-visible spectroscopy is an important technique to establish the formation and stability of NPs in solution (Dhand et al., 2016). The scan was recorded from 300 to 600 nm, showing a characteristic peak appearing within the range of 440 to 450 nm. FTIR was carried out to identify the molecules corresponding to the metabolites involved in reduction, capping and stabilization of the synthetized AgNPs (Haghighi Pak et al., 2016). AgNPs were recorded using a Perkin-Elmer Spectrum One in ATR mode in the 4000 to 500 cm-1 range. The presence of AgNPs was confirmed by EDS, and the surface characterization was performed by SEM analysis. This was done using a JEOL JSM-6010L, with an accelerating voltage of 10 kV and a STEM support. Zeta potential and hydrodynamic sizes of the synthesized NPs were determined by introducing 3 mL of sample in the Nanotrac Wave instrument.
Determination of antimicrobial activity by well-diffusion method
The antimicrobial activities of the NPs were confirmed by well diffusion method against pathogenic microorganisms (Kim et al., 2007). Pure cultures of Escherichia coli (ATCC-25922), Klebsiella cloacae (ATCC-23355) and Staphylococcus epidermidis (ATCC-12228), Manassas, VA, USA were used for antibacterial analysis. Approximately, 80 mL of trypticase soy were dispersed on sterilized Petri dishes. One hundred micro liter (about 100 CFU/mL) of each bacterium was spread uniformly onto the individual plates using sterile cotton swabs. The dried AgNPs were dissolved in distilled water and used immediately. 30 μL of different ratios of AgNPs, 1:10 (A), 1:5 (B) and 1:1 (C) were loaded to each well through a micropipette (positive control), 30 μL of AgNO3 solution and a blank was prepared without AgNPs; all sets were incubated at 37°C for 24 h. Afterwards the zone of inhibition (ZOI) was measured (Haghighi Pak et al., 2016; Lokina et al., 2014). The antimicrobial activity was realized for triplicate for each microorganism.
The collaborative action of biomolecules in the supernatant was visualized through change of the color of the solutions. The supernatant presented the usual faint yellow color of media before reacting with AgNO3. The color tones changed, turning from yellow to brown, indicating that NPs with different sizes were formed (Figure 1). This result indicates that the secondary metabolites obtained from the metabolic activity of Nigrospora sp. fungus in M. oleifera stem aqueous extracts were able to reduce the silver nitrate precursor to AgNPs.
Characterization by UV-Visible and FTIR spectroscopy
AgNPs have free electrons, which give an increment of the surface plasmon resonance (SPR) absorption band due to the interaction of electrons of metal nanoparticles with the light wave (Elumalai et al., 2015; Ghaedi et al., 2015; Gurunathan et al., 2009). A shift of the band maximum from 440 to 436 nm is observed in the UV-Vis spectra depending on the supernatant/AgNO3 ratio (Figure 2). The shift corresponds to change of color, which is due to reduction of Ag+ and formation of AgNPs. The results show that the ratio of supernatant plays an important role in the control of nanoparticle formation. The narrow peak was observed when supernatant was mixed with AgNO3 solution in a 1:10 ratio, indicating yield of nanoparticles. As the supernatant/AgNO3 ratio decreases, the absorption signal increases because more Ag+ are available for interaction with biomolecules from the supernatant, thereby a more intense color results. Also, increasing number of silver nanoparticles were formed as a result of reduction of Ag+. We can affirm that bioactive compounds in the ferment in the Nigrospora sp. fungus were responsible for the accelerated reduction and stabilization of Ag-NPs.
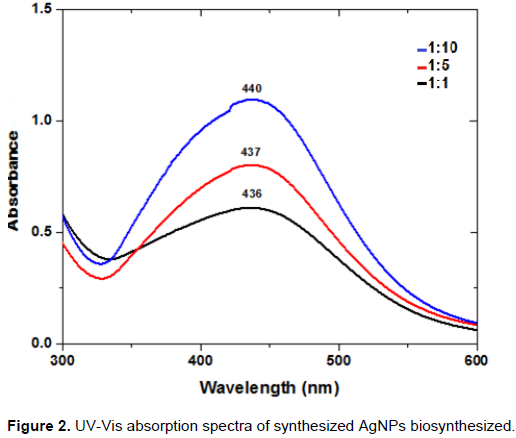
Figure 3 shows the FTIR spectrum of colloid suspension with AgNPs synthetized using M. oleifera stem ferment. The broad band at 3593 cm-1 may be related to OH- groups present in biomolecules. The band observed at 2917 to 2843 cm-1 was assigned to the aliphatic C–H group. The peak around 1624 cm-1 corresponds to C=O stretching vibration, while the peaks observed at 1541 and 1341 cm-1 correspond to secondary amine group and to symmetric bending of CH3, respectively. The peak at 1274 cm-1 is due to SO3- stretching vibration and the one at 1077 cm–1 corresponds to C=O bonds of ether, ester or phenol. Comparison with standard library reveals the presence of characteristic peaks of β-carotenes, flavonoids and tannins, which are actively involved with enzymes in the reduction and stabilization of the AgNPs.
Zeta potential
Zeta potential measurement is an important analysis because it complements the other characterizations and gives information for the solubility, physical stability, colloidal dispersity, and velocity dissolution of the NPs in the suspensions. The measured Zeta potential was ‑200 mV, indicating physical stability of nanoparticles synthetized.
Analysis of AgNPs size distribution
Samples A and B present size distributions with maximums of ~15 and 6.5%, respectively, centered at approximately 35 nm (Figure 4). The distribution maximum of sample C is ~38% at ~3 nm. In addition, the nanoparticles in sample C exhibit better uniformity compared to samples A and B seen as much narrow distribution. The average size of the AgNPs in samples A, B and C was 70, 25 and 3 nm, respectively. The obtained results demonstrate that the supernatant/AgNO3 ratio has an important role in controlling the growth and size of AgNPs during the green synthesis.
SEM and EDS analysis of AgNPs
SEM results on Figure 4 show that the nanoparticles were spherical in shape. Sample A contains particles with larger sizes due to the increment of silver nitrate. This favors the silver ions reduction, which leads to nanoclusters of AgNPs and formation of larger agglomerates in this solution. The EDS results for the three types of colloidal solutions were very similar. In Figure 5 the EDS spectrum of sample A is presented. The main peak is due to silver; the peaks of copper are result of samples preparation on a copper grid. The analysis of SEM and DLS are congruent.
Antimicrobial activity of AgNPs against pathogenic microorganism
Zone of inhibition found for E. coli (E. coli), S. epidermis (S. epidermidis) and K. cloacae (K. cloacae) were 19.5, 19 and 18 mm respectively (Figure 6). Results in Table 1 shows minimal level bacterial inhibition of AgNO3 solutions and blank (ferments alone) in comparison to obtained silver nanoparticles in this study. Results confirm that biosynthesized AgNPs presents antibacterial properties. The antibacterial activity is similar to the reference antibiotic reported (Prasad and Elumalai, 2011). The mechanism bactericide of AgNPs is not fully explored. Maybe antibacterial activity is due to the AgNPs bind of the cell membrane through covalent and ionic bonding, causing changes in its permeability which affects their respiratory functions. They penetrate bacteria, damaging structures containing sulfur and phosphorus based functional groups such as the DNA chains; it also contributes to the bactericidal effect of the Ag+ ions emerging from the surface of the AgNPs (Abdelmoteleb et al., 2016).
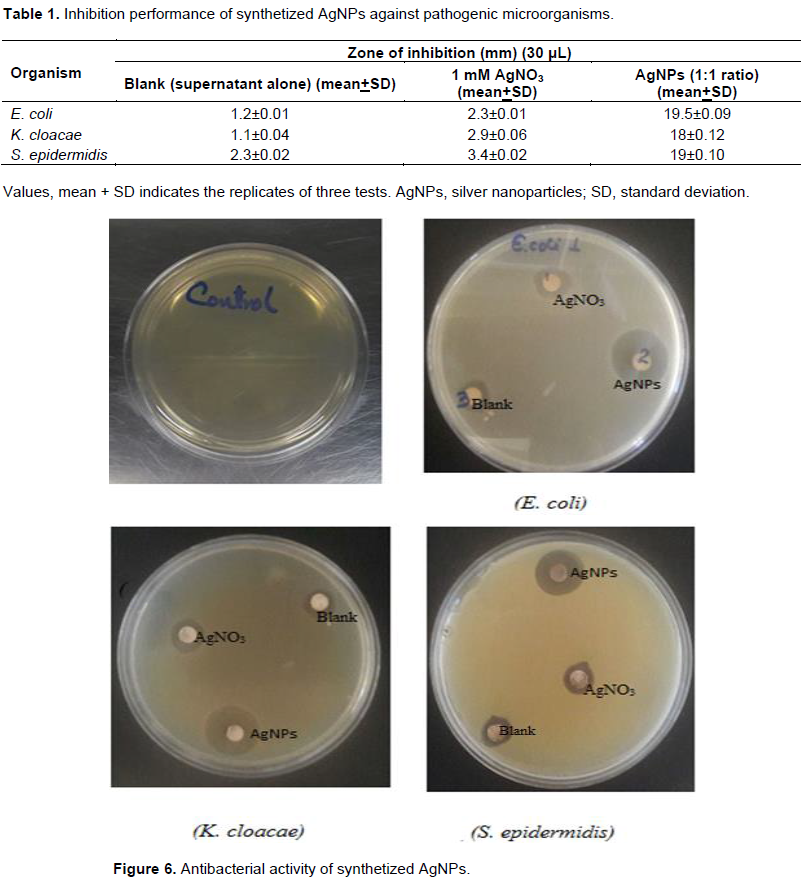
This study demonstrated the synthesis of AgNPs by the reducing effect of secondary metabolites produced by the activity of Nigrospora sp. fungus, based on a simple, safe and green method. AgNPs synthesis was achieved in a short time after mixing the supernatant with the silver nitrate solution. The NPs obtained were characterized by UV-Vis, DLS and SEM-EDS spectroscopy. The characteristic signal in the colloidal suspensions is proven to be sensitive to the detection of AgNPs because they showed a strong absorption peak because the silver nanoparticles exhibit excitation of SPR. UV-Visible analysis showed that the absorption peaks depends on the ratios employed due to the absorbance peaks that decreased as the size of the nanoparticles increased. SEM images shows that sizes of particles observed were well-defined in the 3 to 70 nm range with spherical shapes. EDS analysis of AgNPs confirmed the presence of elemental silver. AgNPs obtained showed antibacterial activity; inhibiting the growth of E. coli, K. cloacae and S. epidermidis. Results contribute to the development of new routes of synthesis utilizing secondary metabolites. Bioactive compounds by Nigrospora sp., fungus from M. oleifera stem stands as potential candidates for biosynthesis and stabilizer of AgNPs in biomedical applications.
The authors have not declared any conflict of interests.
REFERENCES
Abdelmoteleb A, Valdez-Salas B, Cece-a-Duran C, Tzintzun-Camacho O, Gutiérrez-Miceli F, Grimaldo-Juarez O, González-Mendoza D (2016). Silver nanoparticles from Prosopis glandulosa and their potential application as biocontrol of Acinetobacter calcoaceticus and Bacillus cereus. Chem. Spec. Bioavailab. 29(1):1-5.
Crossref
|
|
Ahmed MJ, Murtaza G, Mehmood A, Bhatti TM (2015). Green synthesis of silver nanoparticles using leaves extract of Skimmia laureola: characterization and antibacterial activity. Mater. Lett. 153:10-13.
Crossref
|
|
Anjum S, Abbasi BH (2016). Biomimetic synthesis of antimicrobial silver nanoparticles using in vitro-propagated plantlets of a medicinally important endangered species: Phlomis bracteosa. Int. J. Nanomed. 11:1663.
|
|
Bello OS, Adegoke KA, Akinyunni OO (2015). Preparation and characterization of a novel adsorbent from Moringa oleifera leaf. Appl. Water Sci. pp. 1-11.
Crossref
|
|
Dhand V, Soumya L, Bharadwaj S, Chakra S, Bhatt D, Sreedhar B (2016). Green synthesis of silver nanoparticles using Coffea arabica seed extract and its antibacterial activity. Mater. Sci. Eng. C 58:36-43.
Crossref
|
|
Elumalai K, Velmurugan S, Ravi S, Kathiravan V, Ashokkumar S (2015). Green synthesis of zinc oxide nanoparticles using Moringa oleifera leaf extract and evaluation of its antimicrobial activity. Spectrochim. Acta Mol. Biomol. Spectrosc. 143:158-164.
Crossref
|
|
Ghaedi M, Yousefinejad M, Safarpoor M, Khafri HZ, Purkait MK (2015). Rosmarinus officinalis leaf extract mediated green synthesis of silver nanoparticles and investigation of its antimicrobial properties. J. Ind. Eng. Chem. 31:167-172.
Crossref
|
|
Ghazwani AA (2015). Biosynthesis of silver nanoparticles by Aspergillus niger, Fusarium oxysporum and Alternaria solani. Afr. J. Biotechnol. 14(26):2170-2174.
Crossref
|
|
Gurunathan S, Kalishwaralal K, Vaidyanathan R, Venkataraman D, Pandian SR, Muniyandi J, Hariharan N, Eom SH (2009). Biosynthesis, purification and characterization of silver nanoparticles using Escherichia coli. Colloids Surf. B 74(1):328-335.
Crossref
|
|
Haghighi Pak Z, Abbaspour H, Karimi N, Fattahi A (2016). Eco-Friendly Synthesis and Antimicrobial Activity of Silver Nanoparticles Using Dracocephalum moldavica Seed Extract. J. Appl. Sci. 6(3):69.
Crossref
|
|
Kim JS, Kuk E, Yu KN, Kim JH, Park SJ, Lee HJ, Kim SH, Park YK, Park YH, Hwang CY, Kim YK (2007). Antimicrobial effects of silver nanoparticles. Nanomedicine 3(1):95-101.
Crossref
|
|
Kuppusamy P, Yusoff MM, Maniam GP, Govindan N (2014). Biosynthesis of metallic nanoparticles using plant derivatives and their new avenues in pharmacological applications–An updated report. Saudi Pharm J. 24(4):473-484.
Crossref
|
|
Lokina S, Stephen A, Kaviyarasan V, Arulvasu C, Narayanan V (2014). Cytotoxicity and antimicrobial activities of green synthesized silver nanoparticles. Eur. J. Med. Chem. 76:256-263.
Crossref
|
|
Netala VR, Bethu MS, Pushpalatha B, Baki VB, Aishwarya S, Rao JV, Tartte V (2016). Biogenesis of silver nanoparticles using endophytic fungus Pestalotiopsis microspora and evaluation of their antioxidant and anticancer activities. Int. J. Nanomed. 11:5683-5696.
Crossref
|
|
Prasad TNVKV, Elumalai EK (2011). Biofabrication of Ag nanoparticles using Moringa oleifera leaf extract and their antimicrobial activity. Asian Pac. J. Trop. Biomed. 1(6):439-442.
Crossref
|
|
Suarez-Cerda J, Alonso-Nunez G, Espinoza-Gomez H, Flores-Lopez LZ (2015). Synthesis, kinetics and photocatalytic study of "ultra-small" Ag-NPs obtained by a green chemistry method using an extract of Rosa 'Andeli' double delight petals. J. Colloid Interface Sci. 458:169-177.
Crossref
|
|
Zhao JH, Zhang YL, Wang LW, Wang JY, Zhang CL (2012). Bioactive secondary metabolites from Nigrospora sp. LLGLM003, an endophytic fungus of the medicinal plant Moringa oleifera Lam. World J. Microbiol. Biotechnol. 28(5):2107-2112.
Crossref
|