ABSTRACT
In shallow lakes, waves from different sources play a major role in sediment transport and the release of associated nutrients. This study was conducted to analyze the impacts of waves generated by wind or vessels, on nutrient concentrations and microbial diversity in China’s third largest lake, Taihu. The study area is fixed at 100 m length of sailing vessel and 10 m width from where water samples were collected, near the Taihu Laboratory for Lake Ecosystem Research (TLLER) Station, at Meiliang Bay. The average flow velocity changed during passages of the vessel from -0.1 to + 0.1 m/s with high-speed vessel compering by the time before and after passages for one hour. The significant wave height ranged from 0.002 to 0.698 m. Turbidity ranged from 29.5 to 65.5 NTU. The concentration of Chl-a (μgL-1) and nutrients total nitrogen (TN), total phosphorus (TP), ammonium (NH4+) and phosphate (PO4) were resuspended in the three depths of the water column, as a response to the turbulence caused by waves-waves collision and rivers waves. Microbial amount concentration in the two layers changed over time. It increased in the surface from 5.03 to 8.3 ng µl-1 and decreased in the bottom from 5.03 µl-1 to 2.93 ng µl-1 over a 45 min period. This study points to the importance of sediment resuspension as a factor influencing nutrient availability and microbial community structure, and as such it plays an important role in the eutrophication dynamics of Lake Taihu.
Key words: Destructive interference, wave, vessels, hydrodynamics, nutrients resuspension, microbial diversity.
Water quality is affected by natural and anthropogenic factors. Natural factors include drainage density, lake interchange coefficient, temperature and precipitation within the watershed, lake morphology, water depth, wind and waves. The anthropogenic factors include industrial effluent, agricultural runoff, and effluent from wastewater treatment facilities (Epele et al., 2018; Wang et al., 2009; Xian et al., 2007; Zhou et al., 1996). Algal blooms are indicative of accelerating eutrophication and resultant of poor water quality (Li et al., 2017; Qin et al., 2010; Stumpf et al., 2012). In Lake Taihu, China, poor water quality has become a serious threat to lake, reservoir and other freshwater ecological and economic status and sustainability (Li et al., 2017; Qin et al., 2010). Sediments
are a major internal source of nutrients in shallow lakes like Taihu, where sediment resuspension resulting from currents, waves, dredging, and ship-induced waves can be a source of nutrient enrichment (Gabel et al., 2012; Garrad and Hey, 1987; Maynord, 2005; Qin et al., 2007; Schneider et al., 2007). Microorganisms play a major role in the degradation of organic matter, and the cycling of nutrient elements in freshwater lake sediments (Junier et al., 2007). The lake microbial community is influenced by fluctuating environmental factors, including conductivity, nutrients and organic matter. An increase of organic matter content in the sediment promotes bacterial activity and can influence diversity of prokaryotic populations (Edlund et al., 2006; Fry et al., 2006; Muylaert et al., 2002; Nelson et al., 2007). Excessive nutrient inputs can increase the growth rate of some microorganisms while also affecting microbial habitats and diversity (Weisse, 1991). Studies have shown that the vertical variation of general microbial groups is related to sediment dynamics (Koizumi et al., 2004; Wilms et al., 2006). It is important to know how the partial and temporal distribution of microorganisms responds to environmental drivers accordingly therefore this study: 1) examined impacts of waves generated by wind and vessel on nutrient loads in each layer of water column, 2) measured microbial abundance in water layers, and 3) examined the relationships between microbial abundance and sediment resuspension.
Study area
Lake Taihu is a large freshwater lake located in the lower Yangtze River Delta between 30° 56′– 31° 33′ N and 119° 53′–120° 36′ E. It has a surface area of 2338 km2 and a mean depth of 1.9 m. Meiliang Bay is a semi-enclosed bay with a surface area of 129.3 km2, 1.9 m average depth and it is located in the northern part of Lake Taihu (Gao et al., 2017; Li et al., 2017; Liu et al., 2014). Meiliang Bay is extremely eutrophic, as indicated by cyanobacterial blooms during summer leading to severe water quality problem (Gao et al., 2017; Li et al., 2017). Meiliang Bay has average annual wind speed that varies from 3.5 to 5.0 m/s, with dominant summer winds from the southeast and dominant winter winds from the northwest (Gao et al., 2017; Li et al., 2017; Wu et al., 2013)(Figure 1).
Field observations and instrumentation
Field observations were made on 26 May 2016 in Meiliang Bay Taihu Laboratory for Lake Ecosystem Research, Chinese Academy of Sciences (TLLER). Wind speeds and wind direction, were monitored by a PH-â…¡ handheld weather station and PHWD wind direction transducer; the frequency was set at 5 min. The wind monitoring instruments were positioned at 10 m above the water surface. The data collection interval was 5 min. The data were calibrated with the automatic wind speed and direction recorder (10 m above the water surface, 10 min monitoring interval) located at the TLLER. Wave parameters, including wave height, wave energy, and wave period were measured by Red Blue Reader (RBR) duo T.D wave situated at 50 cm below the water surface. The acoustic Doppler velocimeter (ADV) is the most important instrument to determine and obtain a good understanding for suspended sediment transport. In our field study, we used Acoustic Doppler Velocimeter (ADV) 100 Hz and optical backscatter sensor (OBS-A3) instruments, which have capability to measure the field velocities and suspended sediment concentration (SSC) in the bed layer of Lake Taihu. During the field observation, the vessel moved from one point to another and returned to the beginning several times over a time frame of 60 min. The goal was to understand the precision of effect wave induced by wind (vessel). Water samples were collected during the experiment. The sampling site was located in Meiliang Bay (31°25′7.41″N, 120°12′46.90″E (Figure 1).
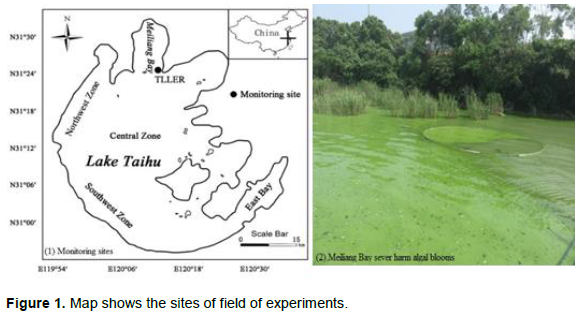
Sample collection techniques
Water samples were collected near the TLLER (311240N, 1201130E) in Meiliang Bay on 26 of May 2016. Samples were collected at different depths; 5 cm above the bottom layer, which is considered descriptive of the water column because of the shallow, middle layer (1 m above the bottom) and near surface 1.8 m above the bottom. One type of sterile bottles (20 samples) was dispensed for DNA extraction and the other 40 samples were collected for water quality from the same site--Meiliang bay and in-lake monitoring stations. Water quality samples were transported to the laboratory for filtration to obtain suspended particulate matter (SPM) content by filtering only 250 mL of water through cellulose acetate membranes (0.45 μm), followed by analyses directly including total phosphorus (TP); total nitrogen (TN); sediments solid; chlorophyll-a (Chl-a) phosphate (PO4) and ammonium (NH4+). These nutrient measurements were according to the Chinese standard methodology for Lake Eutrophication Surveys (Jin and Tu, 1990). This technique is similar to the American standard methods (APHA, 1998), for those parameters (James et al., 2009)while the DNA samples were filtered by using 0.2 µm polycarbonate membrane for DNA extraction in Meiliang Bay. Then the total DNA was removed using the procedure illustrated by Tang et al. (2009) and Zhou et al. (1996). Finally, DNA was measured using 454 pyrosequencing of 16S rRNA genes by using polymerase chain reaction (PCR). The first time sample was collected at zero time, the second time samples was after passages of vessel after 10 min, the third time samples was 20 min, the fourth time samples was 45 min. The vessel moved for 1 h continuously from one point to another point, 100 m distances far away from site of collection the samples.
Data analysis
Acoustic method measures high-frequency suspended sediment concentration (SSC). To obtain high-frequency SSC, we combined Acoustic Doppler Velocimeter (ADV Ocean, SonTek Inc.) and Optical Backscatter Sensor (OBS) turbidity meter, and bottle samples. The first step was to use bottle samples to calibrate with OBS turbidity, and then SSCs was measured by OBS (one datum every 3 min) arithmetic mean value of 3-min echo intensity (EI) data measured by ADV (which frequency was 10 data per second) and obtained logarithmic relationship between EI and SSC. Finally, high-frequency simultaneous SSC and water vertical flow velocity were obtained by ADV according to Gao et al. (2017) and Voulgaris and Meyers (2004). Wind speed and direction were measured at the meteorological station every 5 min at 5 m above water surface. The wind speed was scaled using the logarithmic profile law Equation 1:
Wave characteristics
On 26 May, 2016, the significant wave height ranged from minimum value of 0.002 to maximum value of 0.612 m; the wave energy increased after time experiment from 0.03 to 0.09 NdBar^2 m^-3 and maximum wave height ranged from 0.005 to 0.023 m; the wind speed ranged from 0.5 to 2.5 (m/s) (Figures 2 and 3).
Frequency distribution of wind directions
Major wind directions can have impact on the upper and lower velocities of flow area study and inverse wave flow during strong wind speed. For this determination, wind directions were separated into 14 sides North; East South East; North North East; East; South South East; North East; South West; South; West South West; West North West; West; North West; East North East and North North West. The flow directions were also converted into the same 14 directions to compare them with wind directions (Figure 4).
Wind directions data are presented as dominate north direction with frequencies N (0.166); ESE (0.05); NNE (0.05) E (0.1); SSE (0.0166); NE (0.016); SW (0.066); S (0.016); WSW (0.016); WNW (0.1); W (0.016); NW (0.066); ENE (0.016); NNW (0.083) (Figure 4a). The blue color demonstrates the ranges of wind directions while the white represents the degree of the opposite flow directions which were considered before the experiments. It can be perceived from the frequency statistics that the quadrant directed to the north side creates the highest percent (16.66%) of total frequencies, followed by NNW (8.33%), WNW (10%) and East (10%) of total wind directions (Figure 4b). Thus, it is important to consider them as prevailing wind directions for impact assessments on the flow before vessel passage. Each flow direction was deliberated opposite if it was 90° more than the direction of that precise wind as shown in Figure 4.
Average flow velocities distributions
Table 1 shows that there was a significant strong correlation determination by positive value of P (value < 0.5). The average flow velocities changed during passages of vessel compared to the time before passages of vessel; during the time passages of vessel, the curve shows that the flow average velocities had value that ranged from -0.1 to + 0.1 m/s with high-speed vessel as sweep and ejection events. The varying curve of the flow velocities looks similar and has kelvin wave shape especially after 16 min averages seconds recording measured by Acoustic Doppler velocity (ADV) near distance fixed. The average flow velocities curve gives a clear event of ejection and sweep processes (Figure 5). While, the statistical data of the average velocities during passages and after 5 h passages vessel show that there is no significant correlation determination by P (value > 0.5) with values ranging from minimum value of -0.11 to maximum value of 0.06 (Figure 6; Table 2).
Turbidity and SSC
Nutrients are released from sediment resuspension processes, which encouraged algal growth in the open shallow lakes. The result shows positive relationship between SSC and turbidity (R2=0.538; P<0.5) during passages of vessel (Figure 7).
Wind speed ranged from a minimum of 1.5 to maximum of 2.5 ms-1. Water temperature and pH measurements averaged as 14.3°C and 9.17. Correspondingly, turbidity ranged from 29.5 NTU at 11:00 to a maximum of 50.00 NTU at 12:00 (Figure 8a). Concentrations of TP increased from a minimum of 0.5 mg-1 at 11: 00, to a maximum of 0.14 at 14:00. The data showed a positive correlation between wind speed and turbidity R2= (0. 95, P<0.05) and Figure 8b shows correlation between wind speed and total phosphorus (TP) concentrations [R 2 = 0.7698, P (value < 0.5)].
Nutrients loads
Figure 9 shows the average of chlorophyll-a (μgL-1) and nutrient concentrations during the experiment at three depths; bottom, middle and surface layers. The results demonstrated that the concentration of Chl-a (μgL-1) decreased at bottom from 21.5 to 12.5 μgL-1, middle layer (45.1-22.9 μgL-1) and surface layer (50.2-34.4 μgL-1). TN increased at each layer; bottom (1.8-2.7 mgL-1), middle layer (1.7-2.5 mgL-1) and surface layer (1.7 -2.1 mgL-1), while TP increased in the middle layer (0.09-0.12 mgL-1) and modestly decreased in the bottom (0.8-0.09 mgL-1), and surface layer (0.09-0.11 mgL-1). NH4 increased in the bottom (0.14-0.19 mgL-1), middle (0.13-0.18 mgL-1) and surface (0.14-0.18 mgL-1). PO4 decreased in the bottom layer (0.02-0.03 mgL-1), middle layer (0.01-0.02 mgL-1) and surface layer (0.01- 0.06 mgL-1). SS also increased in each layers (7.93-11.67, 7.8-9.07, 10.93-11.4 mgL-1).
Wave-effect and diversity
Lake Taihu has suffered from severe eutrophication and harmful algal blooms for several decades (Abdul et al., 2017; Li et al., 2017; Paerl and Huisman, 2008). There are multiple sources of pollution, including urban runoff, industrial sewage, and agricultural runoff, and Meiliang Bay is the main drinking water source of Wuxi City (Epele et al., 2018; Li et al., 2017; Zheng et al., 2015). This Bay is the most pollution part and growing different microbial diversity and harmful algal blooms in the Lake. Table 3 shows changes in the distribution of microbial diversity in the two layers during the experiment, with the abundance concentration of total DNA in the bottom layer at 5.03 ng/µl-1 and 8.3ng/µl-1 in the surface layer. Ten minutes after start of the experiment the concentrations in surface and bottom layers decreased to 3.52 and 5.50 ng/µl-1 respectively.
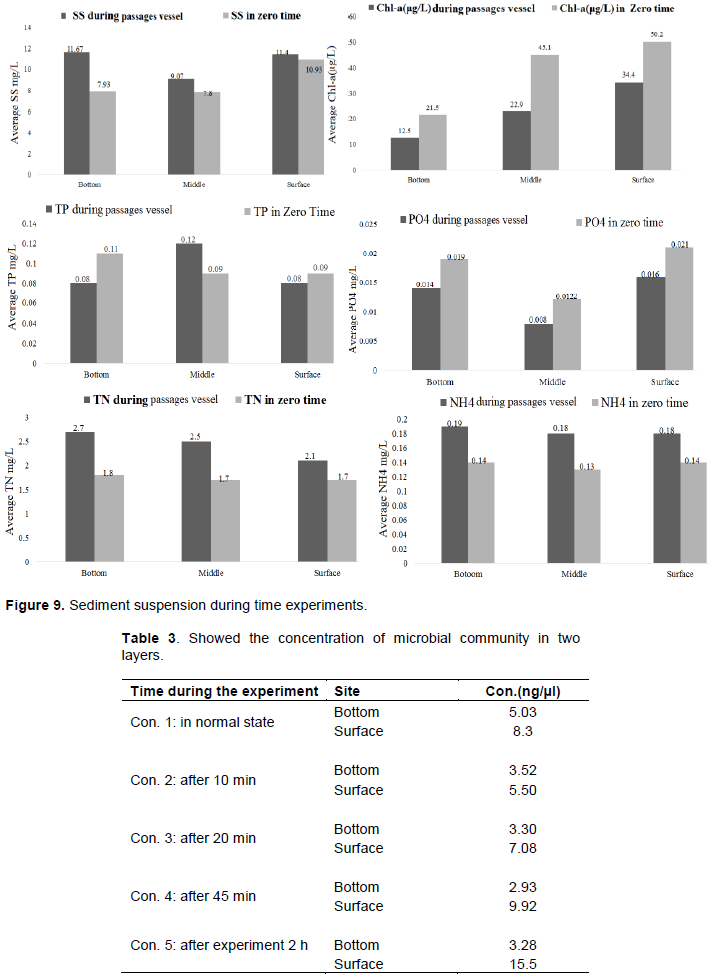
After 20 min, the concentration increased slightly at the surface, to 7. 08 ng/µl-1, and decreased at the bottom to 3.30 ng/µl-1. The concentration at the bottom decreased to 2.93 and increased at the surface to 9.92 ng/µl-1 after 45 min passages vessel. Two hours after the experiment, the concentration increased at the bottom to 3.28 ng/µl-1 and increased to 15.5 ng/µl-1 at the surface.
Wave characteristics and hydrodynamic disturbances
The waves in Lake Taihu are produced by surface strong wind disturbance, and their characteristics essentially depend on wind speed, wind fetch, water depth and size of the vessel. In large shallow lakes, open water area waves are generated when the wind blows across the lake surface (Huang and Liu, 2009); wind energy was absorbed by the surface waves and turbulence, and then degenerated by bottom friction (Green and Coco, 2014; Li et al., 2017). The strong wind can cause algal blooms and other microbial communities to be completely mixed throughout the water column. In addition, the main factor inducing the distributions of wind speed and wave parameters is wind direction (You et al., 2007).
The direction of wind during experiment varied considerably, but dominated from the north. Other study analyzed the directions in Lake Taihu and found the predominant winds were southeast, during summer and the dominant wind speed range was 1.7-6.0 m/s (You et al., 2007). Li et al. (2017)confirmed that the wave heights created by southeast winds were considerably more than wave heights produced by northwest winds during the two periods (5/25/2014 and 5/27/2014), even with similar wind speeds. This is mostly due to varying wind fetch lengths and wind energies. The association between wind speed and wave height at the site showed that the wind speed and wave height were closely related. The waves generated by vessels have raised concerns about their effects on the water body, such as bank erosion, and damage to aquatic plants (Erfurt-Cooper 2009; Gabel et al., 2017). Also, Gabel et al. (2012)demonstrated that ship-induced waves can affect the physical characteristics of lake and river shorelines. Pettibone et al. (1996)found that the waves from a ship cause resuspension of bacteria-colonized bed sediments. The resuspension events occur frequently, internal nutrient loading and high concentrations of suspended particulate matter which can negatively impact in the water quality (Hartmann and Ronneberger, 1996; Li et al., 2017).
In the present study, when the vessel moved forward in the length area of 100 m and 10 m width from site collected the data, and returned to the starting point, it generated progressive waves. The progressive waves were created by the forward movement and the waves created by dominant north wind from the opposite direction collided. The analyses of wind and wave characteristics at steady time and during the experiment showed little to no significant change. This is possibly because both waves have the same amplitude and energy and caused phenomena called destructive interference. The significant wave height ranged from minimum value of 0.002 to maximum value of 0.612 m, the wave energy increased during time experiment from 0.03 to 0.09 NdBar^2 m^-3 and the wind speed ranged from 0.5 to 2.1(m/s).
This findings show the likelihood of wave-wave to collision caused by the waves from the vessel and waves from north winds in the opposite direction. However, the presence of Southeast and Southwest winds caused reverse waves after hitting the solid stage of the site monitoring the Lakeshore and led to exerting an equal downward force on the end of the string. This new force creates a wave pulse that propagates from right to left with the same speed and amplitude as incident wave, but with opposite polarity (upside down) of strong reflectional waves according to Newton's third law (Brown, 1989; Svendsen and Madsen, 1981). The same effort and strength that led to impact on the water surface of the study area lead to generate small waves downwards causing resuspension of sediments and increased the microbial biomass at the time of experiment. Also, the dominant winds from the north directed towards the lakeshore in Meiliang bay caused the same inverse waves, which could be the most important reason for sediment resuspension in this bay (Figures 5 and 6).
Dynamic nutrients loads
The increased turbidity and the positive correlation between wind speed, turbidity R2= (0. 97, P<0.05) may be due to the impact of waves generated by wind (vessel). The wind speed during experiment did not exceed the critical point 3.5 m/s that gave key factor for the resuspension to occur in the water body during weak wind speed and nutrients released. This may consider the cause of eutrophication phenomena and make algal bloom grew in the lake maybe by waves generated by vessels. The mixing of sediments resuspension into the water column does lead to nutrient release, the recurrence and period of resuspension events, as well as relation bioavailability of nutrients (TP, TN, NH4, PO4); therefore, factor in determining the magnitude of water quality impacts from waves.
The slope of relationship (TP=3.98x+55.4, R 2 = 0.7698, P (value < 0.5) (Figure 9) during May 26, 201, had weak wind occurrence, suggesting its potential to provide accurate prediction of the size of change in TP due to sediment resuspension in Meiliang Bay. The enrichment of water column by TP from the sediments could promote nitrogen limitation if a significant proportion of mobilized TP is bioavailable. Furthermore, a large flux of bioavailable phosphorus could maintain eutrophic conditions, in spite of large reductions in external phosphorus loading. On the other hand, if resuspended nutrients continue to exist largely as particle-bound and inaccessible to the biota, the effect on water quality could be comparatively small. Our assessments of resuspension are similar to other shallow eutrophic lake which were determined by Anthony and Downing (2003). Our results in Taihu Lake therefore show that resuspension of the bottom area may be influenced by waves. Frequent wave influence may be by strong wind, vessels, and disturbance of sediments. Efforts should be taken to research more about waves induced by wind, vessels to minimize influence of them, distinguish their impact exactly from the separate wave, which can further minimize the resuspension possibility in the shallow lake.
Many organisms were found in the sediments in higher concentrations than in the water column (Burton et al., 1987). Some microorganisms such as, Sediment-bound enteric bacteria may become resuspended by a verity of perturbations including dredging, storm events, boats and ships traffic (Garrad and Hey, 1987; Pettibone et al., 1996; Yousef et al., 1980). The concentration of some indicator microorganisms increase of 40-50 times in waters below dredging operation as compared to upstream waters (Grimes, 1980; Pettibone et al., 1996). The results indicated (Table 1) that there was a slight decrease of the microbial abundance concentration in the bottom layer especially during time passages of vessel 45 min and increased in the surface layer. This result may be because momentum wave shear stress and wave-wave collided the waves generated by vessel or SE, SW wind. This collide induced turbulence which increased the dissolved solids, soluble reactive phosphorus, turbidity and resuspending the microbial community associated with bottom sediments in the water bodies. Sediments may be resuspended as flocculated particles and sediment gathers can be a dominant form of sediment transport in freshwater. Generation of a wave makes resuspension in the layers (Perkins et al., 2014; Van Donsel and Geldreich, 1971).
In addition, slight increase of microbial abundance in surface layer also provides the suspension process in the lake. Moving the vessel generated waves, resuspending bacteria-colonized bed sediments. The microbes in different layers may also move by motility or a momentum change because the average velocity hydrodynamics change during the experiment. At the beginning of experiment, microbes move from the bed to water column, leading to increased microbial abundance in the others layers Table 1; and at the bottom layer, the wave’s energy transmission to sediments particles attached. This leads to a different transport dynamic of sediments thus changing their mode of transport and deposition processes. The vessels had an impact on the physical characteristic of lake and river shorelines (Gabel et al., 2012;Garrad and Hey, 1987). Vessels induced waves and currents are effects on sediment resuspension and shoreline erosion and it began decades ago (Gabel et al., 2017; Garrad and Hey, 1987; Mason and Bryant, 1975). As a result of flow velocities changed and nutrients concentrations during passages of vessel induced waves and currents. This led to increase effect of shear stresses and sediments particles suspended fallowing by nutrients released which is the reason for supplying algal bloom for growth in a huge quantity. Increased wave energy, turbidity and changing the velocities during the time passages boat are similar with the results of (Gabel et al., 2012; Gao et al., 2017; Garrad and Hey, 1987)(Figures 5, 6). This led to alterations of the bottom morphology, sediment grain sizes and bank collapses (Gabel et al., 2017; Garrad and Hey, 1987; Nanson et al., 1994).
The vessel generated waves and the shear stress from those waves motivated the bottom sediment partials and liberation nutrients and maybe this shear stress is momentum mechanisms according to the turbulent burst that happened, ejections and sweeps during passages of vessel. The results are given a start point for more concentration of the mechanisms happened during momentous events on the bottom layer. In Lake Taihu there were a lot of vessels traveling in the lake and maybe the amount eroded materials depends on the characteristics of the vessels and its waves such as, travelling speed, distance to shore Lake, significant wave height, wave length. Also the characteristics of the location water depths are different in the lake, steepness of shoreline, sediment characteristics, grain size and cohesively, therefore leading to different erosion or suspension rates in the lake. May be in Meiliang bay the lakeshore was eroded from different waves generated by vessels and north winds.
Nutrient concentrations during the experiment at three depths
The average Chl-a μgL-1 and nutrient concentrations during the experiment at the three depths showed changes, with the concentration of Chl-a μgL-1 decreasing at the bottom. Wind-induced waves, especially strong winds, participate in 95% of sediment resuspension and nutrient release and are important for understanding how hydrodynamics affect eutrophication. The water depth at sites of data collection was only a few meters because Lake Taihu is a shallow lake, hence causing long-period waves move to the bottom layer and infiltrate the sediment water boundary that may bring about sediment resuspension and nutrient liberation (Jin and Tu, 1990; Li et al., 2017). Our results appear similar to Carper and Bachmann (1984)which showed that the resuspension of sediments in the lake by waves action and moments shear stress resulted in increased turbidity, thereby circling sediment nutrients back into the water column.
Field observations, analysis of the water column characteristics, effects of vessels and wind-generated waves on SS and microbial abundance were determined in this study. Examinations of nutrient loads in water layers suggest that both waves generated by wind or vessels can be responsible, by increasing resuspension and turbidity in the water column. In the lake, the variances of induced wave such as wind, boats and ships passages may differentially impact the concentration of suspended solid. Our results indicate that waves have a significant impact on suspended sediment concentration. Management strategies in Lake Taihu have thus far concentrated on the mitigation of algal blooms by nutrient load reductions. Future studies should focus on field observations of vessel waves shear stress, their effects on sediment resuspension and nutrient cycling, and their roles in eutrophication and algal bloom the changes in the hydrodynamic of the shallow Lake.
The authors have not declared any conflict of interests.
The research was supported by National key research and development plan (2016YFC0401703), and Chinese National Science Foundation (5177090079, 51579071, 51379061, 41323001, 51539003); National Science Funds for Creative Research Groups of China (No. 51421006); the program of Dual Innovative Talents Plan and Innovative Research Team in Jiangsu Province; the Priority Academic Program Development of Jiangsu Higher Education Institutions, and the Fundamental Research Funds for the Central Universities.
REFERENCES
American Public Health Association (APHA) (1998). American Public Health Association. Standard methods for the examination of water and wastewater. 20th ed. American Public Health Association, Washington, DC.
|
|
Abdul J, Yiping L, Wei D, Wencai W, Jianwei W, Xiaomeng G, Hafiz O, Sarwar K, Kumud A (2017). The role of wind field induced flow velocities in destratification and hypoxia reduction at Meiling Bay of large shallow Lake Taihu, China. Environmental Pollution 10.1016/j.envpol.2017.09.0950269-7491/© 2017 Published by Elsevier Ltd.
|
|
|
Anthony JL, Downing JA (2003). Physical impacts of wind and boat traffic on Clear Lake, Iowa, USA. Lake and Reservoir Management 19(1):1-14.
Crossref
|
|
|
Brown DE (1989). Students' concept of force: the importance of understanding Newton's third law. Physics Education 24(6):353.
Crossref
|
|
|
Burton G, Gunnison D, Lanza GR (1987). Survival of pathogenic bacteria in various freshwater sediments. Applied and Environmental Microbiology 53(4):633-638.
|
|
|
Carper GL, Bachmann RW (1984). Wind resuspension of sediments in a prairie lake. Canadian Journal of Fisheries and Aquatic Sciences 41(12):1763-1767.
Crossref
|
|
|
Donelan MA, Dobson FW, Smith SD, Anderson RJ (1993). On the dependence of sea surface roughness on wave development. Journal of Physical Oceanography 23(9):2143-2149.
Crossref
|
|
|
Edlund A, Soule T, Sjöling S, Jansson JK (2006). Microbial community structure in polluted Baltic Sea sediments. Environmental Microbiology 8(2):223-232.
Crossref
|
|
|
Epele LB, Manzo LM, Grech MG, Macchi P, Claverie AÑ, Lagomarsino L, Miserendino ML (2018). Disentangling natural and anthropogenic influences on Patagonian pond water quality. Science of the Total Environment 613(Supplement C):866-876.
Crossref
|
|
|
Erfurt-Cooper P (2009). European waterways as a source of leisure and recreation. River Tourism pp. 95-116.
Crossref
|
|
|
Fry JC, Webster G, Cragg BA, Weightman AJ, Parkes RJ (2006). Analysis of DGGE profiles to explore the relationship between prokaryotic community composition and biogeochemical processes in deep subseafloor sediments from the Peru Margin. FEMS Microbiology Ecology 58(1):86-98.
Crossref
|
|
|
Gabel F, Garcia XF, Schnauder I, Pusch MT (2012). Effects of shipâ€induced waves on littoral benthic invertebrates. Freshwater Biology 57(12):2425-2435.
Crossref
|
|
|
Gabel F, Lorenz S, Stoll S (2017). Effects of ship-induced waves on aquatic ecosystems. Science of The Total Environment 601:926-939.
Crossref
|
|
|
Gao X, Li Y, Tang C, Acharya K, Du W, Wang J, Luo L, Li H, Dai S, Yu Z (2017). Using ADV for suspended sediment concentration and settling velocity measurements in large shallow lakes. Environmental Science and Pollution Research 24(3):2675-2684.
Crossref
|
|
|
Garrad P, Hey R (1987). Boat traffic, sediment resuspension and turbidity in a broadland river. Journal of Hydrology 95(3-4):289-297.
Crossref
|
|
|
Green MO, Coco G (2014). Review of waveâ€driven sediment resuspension and transport in estuaries. Reviews of Geophysics 52(1):77-117.
Crossref
|
|
|
Grimes D (1980). Bacteriological water quality effects of hydraulically dredging contaminated upper Mississippi River bottom sediment. Applied and Environmental Microbiology 39(4):782-789.
|
|
|
Hartmann M, Ronneberger D (1996). Ejections, Bursts and Sweeps in Stochastic Signals. Advances in Turbulence VI:107-110.
Crossref
|
|
|
Huang P, Liu Z (2009). The effect of wave-reduction engineering on sediment resuspension in a large, shallow, eutrophic lake (Lake Taihu). Ecological Engineering 35(11):1619-1623.
Crossref
|
|
|
James RT, Karl HE, Zhu GW, Qin BQ (2009). Comparative analysis of nutrients, chlorophyll and transparency in two large shallow lakes (Lake Taihu, PR China and Lake Okeechobee, USA). Hydrobiologia 627:211-231.
Crossref
|
|
|
Jin X, Tu Q (1990). The standard methods for observation and analysis in lake eutrophication. Chinese Environmental Science Press, Beijing 240.
|
|
|
Junier P, Witzel KP, Hadas O (2007). Genetic diversity of cyanobacterial communities in Lake Kinneret (Israel) using 16S rRNA gene, psbA and ntcA sequence analyses. Aquatic Microbial Ecology 49(3):233-241.
Crossref
|
|
|
Koizumi Y, Takii S, Fukui M (2004). Depth-related change in archaeal community structure in a freshwater lake sediment as determined with denaturing gradient gel electrophoresis of amplified 16S rRNA genes and reversely transcribed rRNA fragments. FEMS Microbiology Ecology 48(2):285-292.
Crossref
|
|
|
Li Y, Tang C, Wang J, Acharya K, Du W, Gao X, Luo L, Li H, Dai S, Mercy J, Yu Z, Pan B (2017). Effect of wave-current interactions on sediment resuspension in large shallow Lake Taihu, China. Environmental Science and Pollution Research 24(4):4029-4039.
Crossref
|
|
|
Liu J, Wang P, Wang C, Qian J, Hon J, Hu B (2014). The macrobenthic community and its relationship to the contents of heavy metals in the surface sediments of Taihu Lake, China. Fresenius Environmental Bulletin 23(7A):1697-1707.
|
|
|
Mason C, Bryant R (1975). Changes in the ecology of the Norfolk Broads. Freshwater Biology 5(3):257-270.
Crossref
|
|
|
Maynord ST (2005). Wave height from planing and semiâ€planing small boats. River Research and Applications 21(1):1-17.
Crossref
|
|
|
Muylaert K, Van der Gucht K, Vloemans N, De Meester L, Gillis M, Vyverman W (2002). Relationship between bacterial community composition and bottom-up versus top-down variables in four eutrophic shallow lakes. Applied and Environmental Microbiology 68(10):4740-4750.
Crossref
|
|
|
Nanson GC, Von Krusenstierna A, Bryant EA, Renilson MR (1994). Experimental measurements of riverâ€bank erosion caused by boatâ€generated waves on the gordon river, Tasmania. River Research and Applications 9(1):1-14.
Crossref
|
|
|
Nelson DM, Ohene-Adjei S, Hu FS, Cann IK, Mackie RI (2007). Bacterial diversity and distribution in the Holocene sediments of a northern temperate lake. Microbial Ecology 54(2):252-263.
Crossref
|
|
|
Paerl HW, Huisman J (2008). Blooms like it hot. Science 320(5872):57-58.
Crossref
|
|
|
Perkins TL, Clements K, Baas JH, Jago CF, Jones DL, Malham SK, McDonald JE (2014). Sediment composition influences spatial variation in the abundance of human pathogen indicator bacteria within an estuarine environment. PloS One 9(11):e112951.
Crossref
|
|
|
Pettibone GW, Irvine KN, Monahan KM (1996). Impact of a ship passage on bacteria levels and suspended sediment characteristics in the Buffalo River, New York. Water research 30(10):2517-2521.
Crossref
|
|
|
Qin B, Xu P, Wu Q, Luo L, Zhang Y (2007). Environmental issues of lake Taihu, China. Hydrobiologia 581(1):3-14.
Crossref
|
|
|
Qin B, Zhu G, Gao G, Zhang Y, Li W, Paerl HW, Carmichael WW (2010). A drinking water crisis in Lake Taihu, China: linkage to climatic variability and lake management. Environmental management 45(1):105-112.
Crossref
|
|
|
Schneider AR, Porter ET, Baker JE (2007). Polychlorinated biphenyl release from resuspended Hudson River sediment. Environmental science & technology 41(4):1097-1103.
Crossref
|
|
|
Stumpf RP, Wynne TT, Baker DB, Fahnenstiel GL (2012). Interannual variability of cyanobacterial blooms in Lake Erie. PloS One 7(8):e42444.
Crossref
|
|
|
Svendsen I, Madsen P (1981). The dynamics of wave induced ship motions in shallow water. Ocean Engineering 8(5):443-479.
Crossref
|
|
|
Tang X, Gao G, Zhu L, Chao J, Qin B (2009). DNA extraction procedure affects organic-aggregate-attached bacterial community profiles from a shallow eutrophic lake. Canadian Journal of Microbiology 55(6):776-782.
Crossref
|
|
|
Van Donsel D, Geldreich E (1971). Relationships of salmonellae to fecal coliforms in bottom sediments. Water research 5(11):1079IN31081-10801087.
Crossref
|
|
|
Voulgaris G, Meyers ST (2004). Temporal variability of hydrodynamics, sediment concentration and sediment settling velocity in a tidal creek. Continental Shelf Research 24(15):1659-1683.
Crossref
|
|
|
Wang L, Cai Y, Fang L (2009). Pollution in Taihu Lake China: causal chain and policy options analyses. Frontiers of Earth Science in China 3(4):437-444.
Crossref
|
|
|
Weisse T (1991). The Microbial Food Web and its Sensitivity to Eutrophication and Contaminant Enrichment: A Crossâ€system Overview. International Review of Hydrobiology 76(3):327-337.
Crossref
|
|
|
Wilms R, Köpke B, Sass H, Chang TS, Cypionka H, Engelen B (2006). Deep biosphereâ€related bacteria within the subsurface of tidal flat sediments. Environmental Microbiology 8(4):709-719.
Crossref
|
|
|
Wu TF, Qin BQ, Zhu GW, Zhu MY, Wei L, Luan CM (2013). Modeling of turbidity dynamics caused by wind-induced waves and current in the Taihu Lake. International Journal of Sediment Research 28(2):139-148.
Crossref
|
|
|
Xian G, Crane M, Su J (2007). An analysis of urban development and its environmental impact on the Tampa Bay watershed. Journal of Environmental Management 85(4):965-976.
Crossref
|
|
|
You B, Wang T, Fan C, Zhu L, Zhong J, Li B, Yin H, Hu C (2007). Quantitative simulative method of sediment resuspension in Lake Taihu. Journal of Lake Sciences 19:611-617.
Crossref
|
|
|
Yousef YA, McLellon WM, Zebuth HH (1980). Changes in phosphorus concentrations due to mixing by motorboats in shallow lakes. Water Research 14(7):841-852.
Crossref
|
|
|
Zheng SS, Wang PF, Chao W, Jun H (2015). Sediment resuspension under action of wind in Taihu Lake, China. International Journal of Sediment Research 30(1):48-62.
Crossref
|
|
|
Zhou J, Bruns MA, Tiedje JM (1996). DNA recovery from soils of diverse composition. Applied and Environmental Microbiology 62(2):316-322.
|
|