ABSTRACT
Microbial communities of six samples from Escravos and Meren oil production facility in Nigeria were examined after biocide treatment using cultural and molecular approaches. Sulfate reducing bacteria (SRB) count and activity were the highest in skimmer pit samples (1N1) which was affiliated with Euryarchchaeota (44%) and Proteobacteria (39%). Treatment of Meren injection water (1N2) with biocides significantly reduced SRB population (102 cell/ml). Sample 1N3 (produced water discharge area) and 1N5 (treated produced water) were dominated by 70.79 and 52% Euryarchaeota, respectively. 1N4 (partially treated produced water) was dominated by Euryarchaeota (51.78%) and Proteobacteria (38%), while 1N6 (produced oil) was dominated by Proteobacteria (28%) and Euryarchaeota (62.6%). Metabolic activities in 1N1, 1N4 and 1N5 were dominated by sulfate reduction and methanogenesis; however, that of 1N2 was dominated only by sulfate reduction, while 1N3 and 1N6 were dominated by methanogenesis. Anaerobic incubation of 1N1 samples gave relatively higher corrosion rates (0.065 mm/year) while 1N2, 1N3 and 1N5 gave moderate corrosion rates (0.018 to 0.037 mm/year). This investigation in a Nigerian oil facility have substantially expanded our knowledge on the extent of microbial diversity in these fields after routine biocide treatment and have also shown the abundance and complexity of microbial communities in these fields that have potential to initiate souring and corrosion despite regular biocide treatment.
Key words: Sulfate reduction, methanogenesis, corrosion, souring, microbial diversity.
Nigeria presently ranks about the 6th position in the world in terms of crude oil production with a maximum daily production capacity of 2.5 million barrels per day and it is also the world’s 10th largest oil reserves estimated at about 25 billion barrels (OTC, 2002). Known in the energy circle as a “Gas province with a little pool of oil”, the country’s gas reserve is put at over 166 trillion standard cubic feet and the current gas production is put at 2 billion standard cubic feet (OTC, 2002).
The constant demand for oil has driven more interest in the study of petroleum reservoir microbial diversity as microbial activity in an oil reservoir can have significant implications on oil quality and recovery (Head et al., 2003). For example, microbial activity can have costly negative effects such as hydrocarbon degradation, clogging, souring and corrosion of pipelines (Magot et al., 2000; Nimati et al., 2001; Li and Hendry, 2008; Voordouw, 2011) or beneficial effects on the rate or extent of oil recovery and productivity (Lake, 1989; Youssef et al., 2009). In the recent years, an assessment of microbial diversity and habitat conditions within a petroleum reservoir is being increasingly recognized as an important component of reservoir management (Li et al., 2012). A comprehensive assessment of the diversity, metabolic processes and habitat conditions for petroleum microorganisms is therefore of practical importance for assessing the economic potentials of oil fields. It will also help in the understanding of how in-situ biotic factors may affect oil production operations (Pham et al., 2009). A study by Van der Kraan et al. (2009) have also indicated how the presence of specific microbial communities could present information on some characteristics of oil reservoir such as temperature, acidity, salinity, and redox-potential, etc.
In Nigeria, Meren field is located south east of Lagos, Nigeria with an estimated oil reserves of 1.8 billion barrels, out of which about 750,000 barrels has been produced as at 2002 (Lumley et al., 2000). The current production capacity is about 85,000 barrels per day. Sea water picked up to 25 ft below sea level is treated with continuous injection of sodium hypochlorite with residual chlorine maintained at 1.5 mg/L. Apart from continuous chlorination, two other biocides (amine and quaternary ammonium compound and a gluteraldehyde) have been applied alternatively for 6 h weekly at a concentration of 200 pm. Even with the biocide application, it has been reported that total elimination of sulfate reducing bacteria (SRB) from Meren field is difficult (Adetoba, 1985; Lumley et al., 2000). SRB are mostly anaerobes and they have the ability to reduce sulfate ions in injection water to sulfide ion which can result to souring and pitting corrosion (Voordouw, 2011; Hubert et al., 2005). The insoluble sulfide formed can also cause plugging in filters and formation (Hubert et al., 2005). Meren sea water is a semi-closed system so both aerobic and anaerobic microorganisms co-exists and the de-aerated sea water provides an almost oxygen free environment for sulfate reducing bacteria (SRB) to thrive.
Escravos processing facility on the other hand is a sharp contrast from Meren. The facility is located nearshore in the Niger Delta, about 100 km south east of Lagos, Nigeria. It is comprised of a tankfarm where escravos crude oil is stored and loaded, a processing facility for raw crude coming from Abiteye, Okan and Olero creek (with a capacity of about 70,000 barrels of crude/day) and a gas to liquid facility (EGTL) that processes about 325 million cubic feet per day of natural gas (http://www.chevron.com). Within the Escravos facility is an oily waste storage pit (Skimmer pit) where oily waste that drains from the facility is stored temporarily before treatment and disposal. With an average temperature of about 60°C, it is expected that skimmer pit microbal flora will be dominated by moderate thermophiles. There is no sea water reinjection at Escravos facility as it is done at Meren but the same routine of biocide application is applied at Escravos as it is done at Meren. Produced water is mechanically treated to knock down the hydrocarbon level below 50 ppm before being disposed at nearby Escravos river. Considerable concentrations of SRB has been detected in Escravos produced water and the receiving sea water (Okoro et al., 1999, 2010) despite regular biocide treatment.
In characterization of microbial communities, culture independent 16S rRNA gene pyrosequencing approach which has been proven to be very efficient and reliable as opposed to the culture dependent methods which greatly underestimates the microbial diversity associated with an environment (Pham et al., 2009; Voordouw et al., 1996, Orphan et al., 2000; Grawbowski et al., 2003; Li et al., 2007; Nazina et al., 2007; Dahle et al., 2008; Okoro et al., 2014). Culture independent 16S rRNA gene based surveys are extremely valuable in providing an overall view of the community composition in a specific ecosystem regardless of the metabolic activities of the community members (Youssef et al., 2009; Gittel et al., 2009). These studies also provide preliminary information on the relative abundance of different groups of organisms within the ecosystem and how this could be used in monitoring temporal and spartial changes within the ecosystem.
Unfortunately, information on microbial diversity and habitat conditions in Nigerian oil fields is scantly in literature and this has provided strong incentives for a detailed study of microbes present in Nigerian oil fields and in production operations. To the best of our knowledge, there has been no recorded study on the microbial communities of these fields that persist after routine biocide treatments and with potential to induce souring and corrosion. The main objective of the present study therefore is to characterize the microbial communities associated with corrosion and souring in Chevron’s Meren offshore field and Escravos production and processing facility after routine biocide treatment. The present study will therefore provide some useful information that can be used to mitigate potential cases of souring, plugging, corrosion and fouling after biocide treatment and can also provide information on the efficacy of biocides currently used in these facilities to mitigate souring and corrosion.
In the present study, samples were collected from two Nigerian oil production facilities (Meren and Escravos facility) after routine biocide treatment and analyzed both chemically and microbiologically.
Site and process description
Six samples, 1N1, 1N2, 1N3, 1N4, 1N5 and 1N6 from Escravos and Meren oil production facilities (Table 1) were collected in sterile 500 ml Nalgene sample bottles, filled to the brim to exclude air and shipped to the University of Calgary, Alberta Canada for further analysis within one week of collection. From Escravos facility, samples were collected from skimmer pit which is a temporary storage pit for oily wastes arising from hot water flushing and cleaning of the oil facility. Its temperature is usually elevated ranging from 45 to 60°C. Another point of sample collection at Escravos is the point of produced water discharge where sea water mixes with produced water. At Meren offshore facility, both treated and partially treated produced water samples and samples from wellhead were collected. Injection sea water which was picked from 25 ft below sea level, filtered and treated with biocides were also collected. On arrival at the University of Calgary, samples were transferred to Coy anaerobic hood with an atmosphere of90% (v/v) N2 and 10% (v/v) CO2.
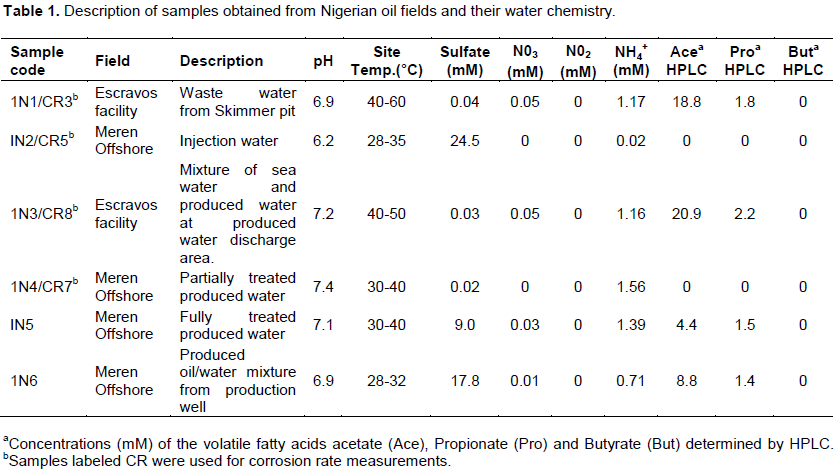
Chemical analysis
The pH of the samples was measured using an Orion pH meter. Aqueous sulfide was analyzed by using the diamine method (Trüper and Schlegel, 1964) and NH4+ by the indophenol method (APHA, 1992). Sulfate, NO3-, NO2- and the volatile fatty acids (VFA) acetate, propionate and butyrate were analyzed by high-performance liquid chromatography (HPLC), as described previously (Grigoryan et al., 2008). For analysis of inorganic anions, 100 µL of sample was combined with 400 µL HPLC anion buffer, while for analysis of VFA, 300 µL of the sample were combined with 20 µL 1 M phosphoric acid.
Measurement of microbial activities and most probable numbers
The activities of SRB, as well as of heterotrophic nitrate-reducing bacteria and sulfide-oxidizing nitrate-reducing bacteria (hNRB and soNRB) were measured in Coleville synthetic brine (CSB-K) medium (Nemati et al., 2001). Medium was anaerobically dispensed in 70 ml aliquots in 125 ml serum bottles with a gas phase of 90% N2 and 10% CO2, and closed with sterile butyl rubber stoppers. Medium was amended with 40 mM lactate and 20 mM sulfate or 3 mM VFA and 20 mM sulfate for measurement of SRB activity, with 3 mM VFA and 10 mM nitrate for measurement of hNRB activity, and with 5 mM sulfide and 10 mM nitrate for measurement of soNRB activity. The 3.5 ml of each sample was injected into the medium in the bottle and those were incubated at 37°C on shaker. Using a sterile syringe needle, 1 ml was taken periodically to determine the sulfide, sulfate, nitrate and nitrite concentrations. Microbial activities were calculated as 100/t1/2 units/day, where t1/2 is the time (days) needed to reduce half of the sulfate (SRB activity) or nitrate concentration (hNRB and soNRB activities), or oxidize half of the sulfide concentration (so-NRB). The most probable number (MPN) of lactate-utilizing SRB was determined, using vials with 9 ml of anaerobic API RP-38 broth. These were inoculated with 1 ml of sample and with 10-fold dilutions derived thereof. Formation of a black iron sulfide precipitate was used to score the presence of SRB. The vials were incubated at 37°C for 30 days after which the MPN was determined. For acid-producing bacteria (APB), ZPRA-5 phenol red-dextrose medium with a salinity of 5000 ppm was used. Change in colour from orange to yellow showed the presence of acid producers.
Iron corrosion testing
Iron coupons (2×1×0.1 cm3) were cut from ASTM A366 carbon steel with 0.015% (w/w) carbon (ASTM international designation A1008/A) and cleaned according to a standard protocol (NACE RP0775-2005) in which the coupons were polished with 400 grit sandpaper and then placed in a dibutyl-thiourea-HCL solution for 2 min. The coupons were then neutralized in a saturated bicarbonate solution for 2 min, rinsed with dH2O and then with acetone and finally dried in a stream of air. The coupons were weighed 3 times and the average weight was recorded as the starting weight. Duplicate coupons in small plastic holders to prevent them from contacting the glass wall or each other were placed in 120 ml serum bottles containing 70 ml of sample under anaerobic conditions (headspace of 90% v/v N2 and 10% CO2; N2-CO2). One set contained unfiltered sample, whereas another set contained sample filtered through 0.2 µm acrodisc syringe filters. Serum bottles with 70 ml of filtered Milli-Q purified water were used as a control. The samples were incubated at 32°C while being shaken at 60 rpm. Samples (1 ml) were withdrawn weekly for chemical analysis with a sterile syringe flushed with N2-CO2. After the incubation period, the coupons were cleaned and dried according to the NACE standard protocol and weighed again. The corrosion rate (CR) was determined from the metal weight loss (∆W in g) as:
CR = 87,600 × âˆ†W/(A × D × T) mm/year
Where A, D and T represent the coupon area (4.6 cm2), the density of the steel (7.85 g/cm3) and the incubation time (h), respectively. (Okoro et al., 2014).
DNA extraction, amplification, sequencing and bioinformatic analysis
Genomic DNA was extracted from 40 ml of samples 1N1 to 1N6 using the MP Biomedical FastDNA kit.. Extracted DNA (2 ng μL−1) was then amplified through 25 PCR cycles. The PCR products were purified and subjected to a second round of 10 PCR cycles with pyrosequencing primers 454T-RA-X (which is barcoded) and 454T-FB, which have 926f and 1392r (Park et. al., 2011) as their 3’ ends. PCR product quality was verified on an 0.7% agarose gel and PCR products were purified with a QIAquick PCR Purification Kit (Qiagen) following which their concentrations were determined on a Qubit Fluorometer (Invitrogen), using a Quant-iT dsDNA HS Assay Kit (Invitrogen). Detailed procedures have been described (Park et al., 2011). PCR products (typically 20 μL of 5 ng μL−1) were sent to the Genome Quebec and McGill University Innovation Centre for pyrosequencing with an FLX Instrument, using a GS FLX Titanium Series Kit XLR70 (Roche Diagnostics Corporation). Data analysis was conducted with Phoenix 2, a 16S rRNA data analysis pipeline, developed in house (Park et al., 2011; Soh et al., 2013). High quality sequences that remained following quality control and chimeric sequence removal were clustered into operational taxonomic units at 3% distance by using the average linkage algorithm (Schloss and Westcott, 2011). A taxonomic consensus of all representative sequences from each of these was derived from the recurring species within 5% of the best bitscore from a BLAST search against the SSU Reference data set SILVA102 (Pruesse et al., 2007). Amplicon libaries were clustered into a Newick-formatted tree using the UPGMA algorithm with the distance between libraries calculated with the thetaYC coefficient (Yue and Clayton, 2005) as a measurement of their similarity in the Mothur software package (Schloss et al., 2009). The Newick format of the sample relation tree was visualized using Dendroscope (Huson et al., 2007). The entire set of the raw reads is available from the Sequence Read Archive at the National Center for Biotechnology Information (NCBI) under accession numbers; SRR631214, SRR631215, SRR631216, SRR631217, SRR631828, and SRR631829.
Chemical characterisation of samples
Results for chemical analysis of samples are indicated in Table 1. Partially treated produced water samples from Meren offshore, water samples from produced water discharge area at Escravos and samples from Escravos skimmer pit had low sulphate concentrations which ranged between 0.02 and 0.04 mM while samples from treated produced water, produced oil and injection water from Meren offshore had relatively high sulphate concentration which ranged between 9 and 24.5 mM. Nitrate concentrations were low in all samples (0-0.05 mM). Ecsravos skimmer pit samples and water samples from produced water discharge area had relatively high acetate concentrations which ranged between 18.8 and 20.9 mM; the rest of the samples had relatively low acetate concentrations. Propionate was present at relatively low concentrations in samples 1N1, IN3, 1N5 and 1N6 (1.4-1.8 mM) while butyrate was not detected in any of the samples. Ammonium ion was present in all the samples (0.02-1.39 mM). Meren offshore injection water samples recorded the lowest concentration of ammonium ion (0.02 mM) while partially treated produced water from Meren offshore recorded the highest (1.56 mM).
Microbial activities and counts
Detailed microbial activities are indicated in Figures S1 to S6 (Supplementary material) while microbial counts are shown in Table 2. Partially treated produced water, treated produced water and oil/water mixture from Meren offshore showed no SRB counts (Table 2), consequently there were no activities of lactate utilising and VFA utilising SRB as well as VFA utilising hNRB and so-NRB (Figure S4 to S6). Injection water samples from Meren offshore had relatively low SRB counts (102 cell/ml) with moderate activities of lactate utilizing and VFA utilizing SRB (Figure S2); however, significant concentrations of sulphide were generated in lactate media. There were no activities of hNRB and so-NRB. The highest concentration of SRBs were detected in samples from Escravos skimmer pit (106 cell/ml) followed by samples from produced water discharge area at Escravos (105 cell/ml), consequently activities of lactate utilizing and VFA utilizing SRB were high (Figures S1 and S3) with considerable generation of sulphide in lactate media. There were no activities of hNRB and so-NRB in skimmer pit water samples but considerable activities of hNRB and so-NRB were recorded in samples from produced water discharge area at Escravos with significant reduction of sulphide by the so-NRB. It will be recalled from the chemical analysis data that samples from produced water discharge area at Escravos had some residual nitrate concentration and possibly presence of indigenous so-NRB.
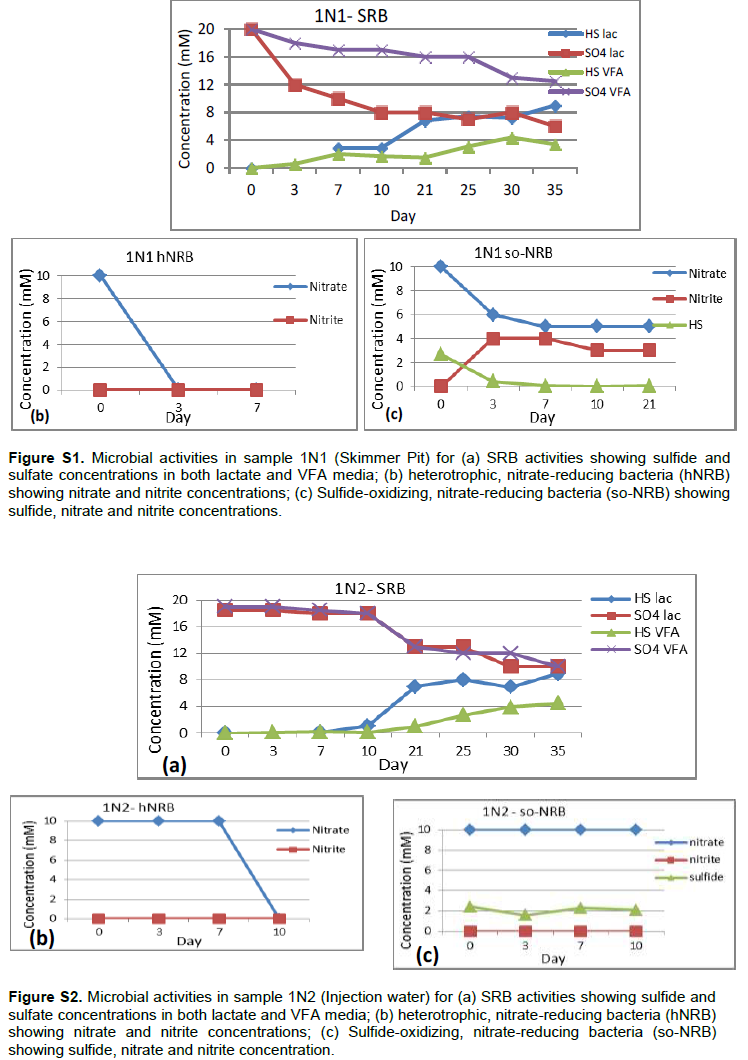
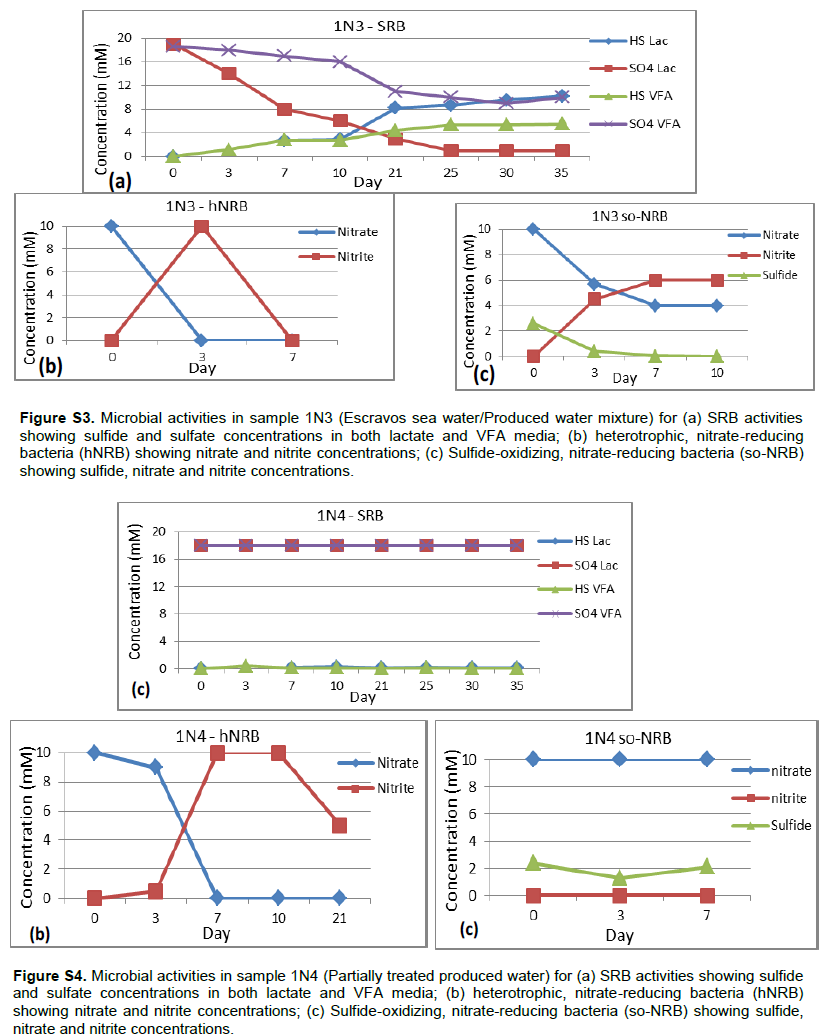
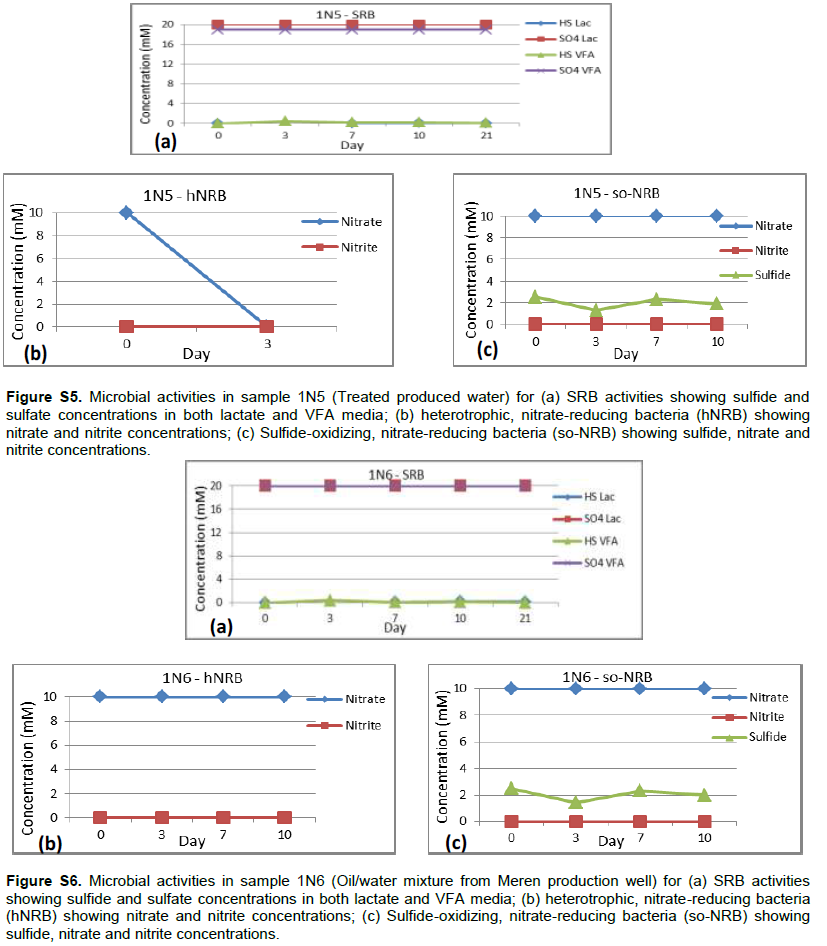
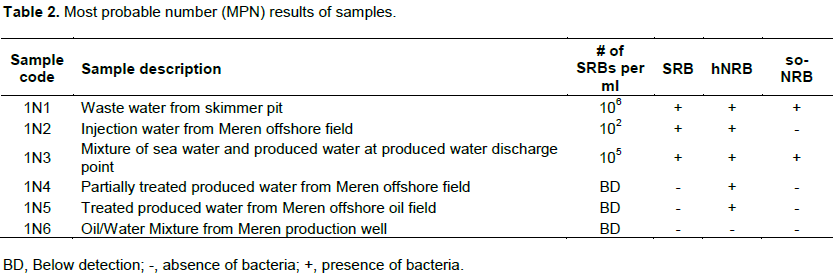
Microbial communities in Escravos and Meren offshore samples
Bacterial DNA was success fully extracted and purified from all samples including those with zero SRB counts and microbial activity. This was done to show further proof of the usefulness of culture independent methods in identifying more diverse group of organisms that could not be identified with culture dependent methods. Following PCR amplification and purification by agarose gel electrophoresis which showed bands that verified the presence of 16S rRNA gene in all the samples (Figure 1); the 16S rRNA amplicons of the samples were subjected to pyrosequencing. The sequences clustered into 191 to 218 OTUs (groups of sequence with 95% sequence identity) for Escravos samples and 152-359 OTUs for Meren samples. The relationship between the number of identified OTUs and the number of sequences is shown in refraction curves (Figure 2) and the relationship three is shown in Figure 3. These indicated that microbial diversity was on average higher at Meren offshore than at Escravos neareshore. The normalised shanon index on the average was also higher at Meren than Escravos (Table 3).
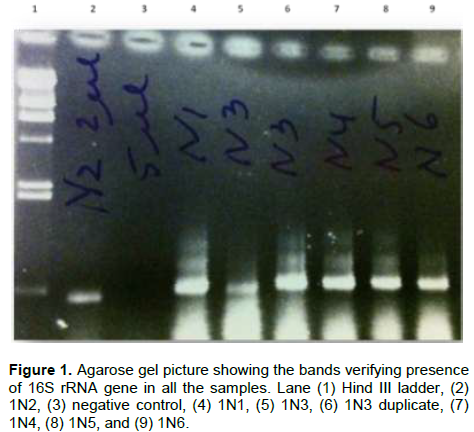
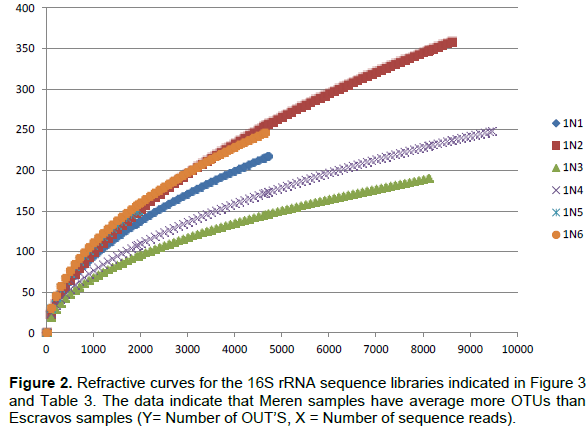
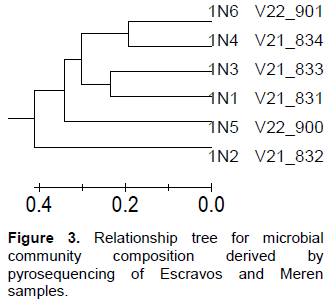
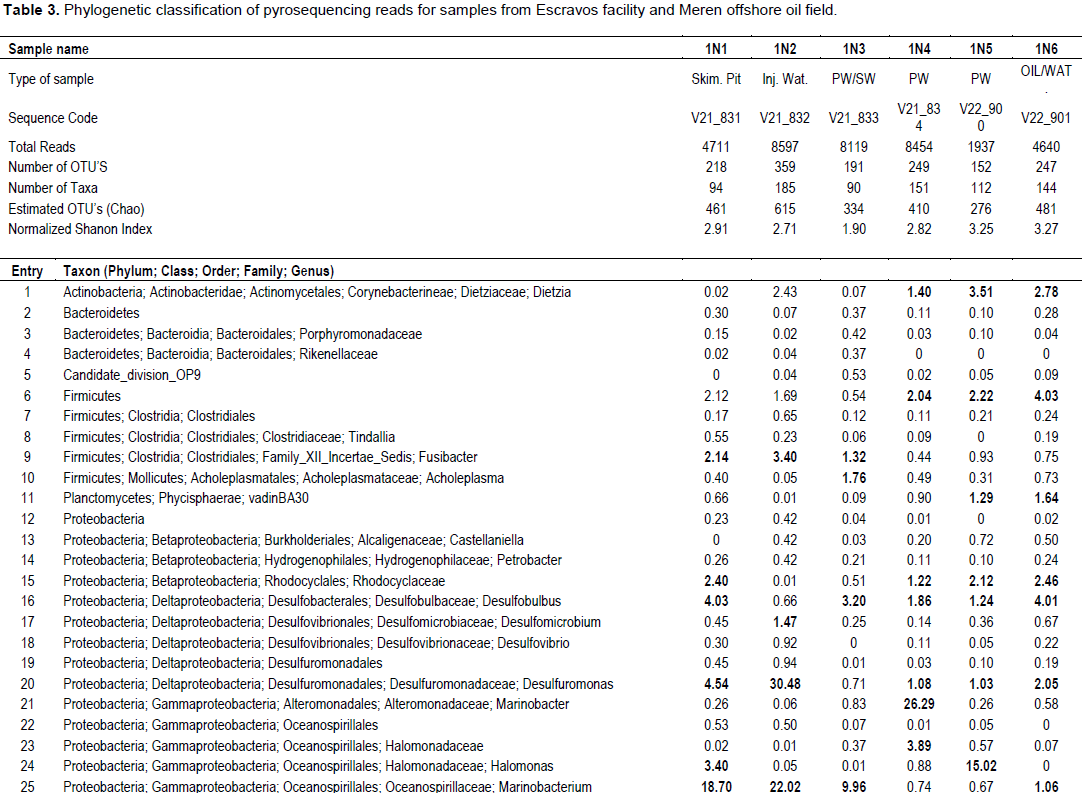
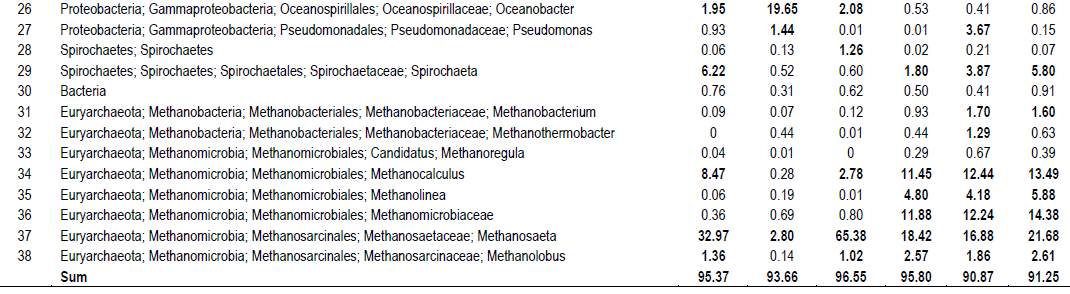
Sequence analysis indicates the presence of 90 to 185 taxa in microbial communities in these fields (Table 3). Microbial community diversity analysis showed that the Escravos skimmer pit samples (1N1) were dominated by methanogenic archeae with genus Methanosaeta, an acetotrophic methanogen and another predominant microbial flora is the proteobacteria with genus Marinobacterium. Samples from Escravos skimmer pit (1N1) showed some similarity in microbial composition and diversity with those from produced water discharge area (IN3) suggesting that there might be some form of relationship between them. For instance, the same methanogenic archeae that dominated skimmer pit water samples also dominated produced water discharge area samples). The presence of moderate acetate concentration from Escravos (18.8 to 20.9 mM) may be the reason for observable presence of acetotrophic methanogens in these samples. Comparatively, microbial composition and diversity from Meren samples are considerably different from those from Escravos. For instance, Meren injection water were dominated by Proteobacteria (Deltaproteobacteria) with genus Delsufuromonas detected followed closely by Gammaproteobacteria with genus Marinobacterium and Oceanobacter present). Generally, the partially treated produced water (1N4) and fully treated produced water (IN5) showed some similarity in microbial diversity as shown in Table 3; however, the composition of the microbial flora was differed. While the genus Marinobacter was present in sample IN4, Holomonas was present in 1N5.
Microbial diversity and composition of oil water mixture (produced oil from well head) from Meren were similar with those of Meren produced water (partially treated and treated). They were dominated by the presence of methanogenic archeae with genus Methanocalculus, Methanolinea, Methanomicrobiacea, Methanosaeta and Methanobolus. It should be noted that these samples (1N4-1N6) recorded no SRB counts and activities of SRB, hNRB and so-NRB. Methanogenic activities which were not part of the initial culture dependent analysis carried out and which is significant in corrosion and souring related issues was revealed by the culture independent analysis as indicated in Table 3.
Corrosion rates of coupons exposed to Escravos and Meren samples
The corrosivity of four samples (1N1, IN2, IN3 and IN4) which showed the considerable bacterial and archaeal activities was examined as part of a larger experiment in which incubations were labelled CR1 to CR8. Escravos samples are referred to as CR3/1N1 and CR8/1N3 whereas Meren samples are referred to as CR5/1N2 and CR7/IN4 as shown in Figure 4. General corrosion rates were determined by weight loss of coupons suspended in unammended samples under anaerobic conditions with head space of 90% (v/v), N2 and 10% (v/v) CO2. Following incubation for 4 weeks, observable precipitate indicating FeS formation was evident in samples CR3/1N1 and and CR8/1N3 as shown in Figure 4. Filtration of samples through a 0.2 µm filter decreases sulphide formation and associated corrosion in sample CR8/1N3 but not in sample CR3/1N1. Corrosion rates obtained from the measured weight loss of the carbon steel coupons were 0.065 ± 0.0078 (CR3/1N1), 0.037 ± 0.003 (CR5/1N2), 0.026 ± 0.0025 (CR7/1N4), and 0.060 ± 0.0018 (CR8/1N3).
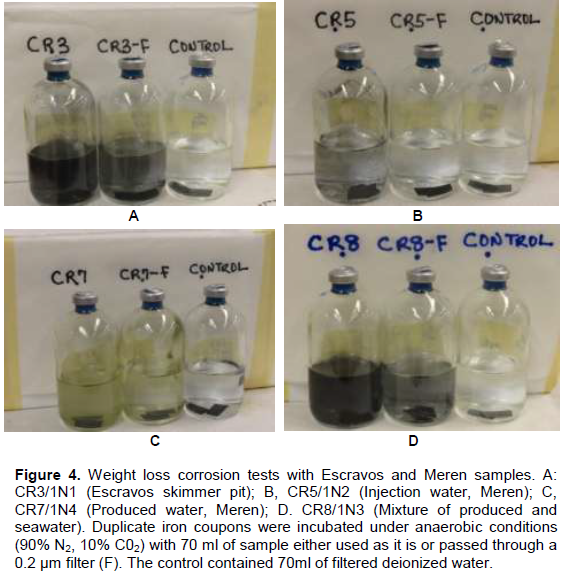
It is a well-established scientific fact that oil reservoirs harbour and sustain diverse bacterial and archaeal communities (Zhu et al., 2003; Grabowski et al., 2005; Youssef et al., 2009, Li et al., 2006 and 2007) and multiple groups of microorganisms with diverse physiological and metabolic activities and phylogenetic affiliations have routinely been recovered from oil reservoirs (Voordouw et al., 1996; Orphan et al., 2000; Green and Voordouw, 2003; Jan-Roblero et al., 2004; Magot, 2005; Li et al., 2006, 2007, Dahle et al., 2008; Pham et al., 2009; Okoro et al., 2014). A comprehensive assessment of the diversity, metabolic processes and habitat conditions within a petroleum reservoir is therefore an important component of reservoir management because the knowledge gained can be useful in mitigating ahead of time potential cases of souring and bio-corrosion and can also be used to improve oil production and recovery processes (Li et al., 2012; Youssef et al., 2009).
In the present study, we have used both cultivation based and molecular methods to characterise microbial communities with potential for souring and corrosion after routine biocide treatment in two Nigerian oil production facilities that has been producing oil for the past 20 years with a combined production capacity of about 155,000 barrels per day. Among the six samples that were analysed, we found the highest SRB counts (106 cell/ml) and activities in sample 1N1 which was collected from the waste water of skimmer pit where all the liquid wastes from Escravos facility drains into there/it after washing and high temperature flushing of the facility. The physicochemical analysis indicated very low sulphate concentration in the skimmer pit (0.04 mM) suggesting that high SRB population may have depleted the available sulphate with possible generation of hydrogen sulphide which can result to souring (Vance and Trasher, 2005). The pyrosequencing survey indicated that this sample contained about 94 anaerobic taxa including the dominant acetotrophic methanogen, Methanosaeta. Another prominent methanogen present is the methylotrophic methanogen, Methanolobus. Among the SRB are the predominant Marinobacterium, Oceanobacter, Holomonas, Desulfuromonas and Desulfobolus. Escravos facility processes crude from Abiteye (Swamp location), Okan (Offshore) and Olero creek (swamp) and the microbial community of the skimmer pit where oily waste drains into is expected to reflect those of Abiteye, Okan and Olero. Compared with samples collectedfromtreatedproducedwaterdischargeareaat Escravos which is represented in sample 1N3, we also observed high SRB counts (105 cell/ml) and activity and most microorganisms that were found to be predominant in skimmer pit samples were also found to be predominant in samples collected from produced water discharge area. Some examples include Acetotrophic methanogen, Methanosaeta and Methylotrophic methanogen, Methanolobus. The observed SRB from the samples were Oceanobacter, Marinobacterium and Desulfobulbus. The data suggest that the microbial communities of skimmer pit and produced water discharge area at Escravos are similar and possibly represent indigenous microbial communities of Abiteye, Okan and Olero creek. Youssef et al. (2009) advanced that the global presence of specific microbial lineages in geographically isolated oil reservoirs is a good indication of their indigenous nature, on that note we state that most of the indigenous microbial communities from the present study are truly indigenous because they have been isolated elsewhere in oil field environments from different geographical locations around the world for example; Methanobacteriales (Faisal et al., 2013), Methanosaeta (Dahle et al., 2008), Methanolobus (Pham et al., 2009), Desulfobulbus (Wilms et al., 2006), Spirochaeta, Desulfomicrobium (Dahle et al., 2008), Marinobacter, Halomonas (Van der Kraan et al., 2009), and Marinobacterium (Li et al., 2012). These microorganisms have always been associated with corrosion and souring and may have been responsible for the corrosion of metal coupons and subsequent production of hydrogen sulfide under laboratory conditions (Figure 2).
Treatment of Meren injection water with continuous chlorination and alternate application of an amine and gluterldehyde biocide at a concentration of 200 ppm (6 h weekly) significantly reduced SRB population (102cell/ml) and activity but pyrosequencing survey reveal that some SRB such as Desulfuromonas, Marinobacterium, and Oceanobacter with potential for souring and corrosion still persists confirming earlier assertion that SRB was difficult to eliminate at Meren field despite continuous biocide treatment (Adetoba, 1985; Lumley et al., 2000). The pyrosequence data also suggests that the major metabolic processes in Meren injection water is sulphate reduction as opposed to methanogenesis that seem to dominate microbial activities in Meren production well and produced water. Grabowski et al. (2005) observed that methanogenesis is a dominant terminal process in a low temperature biodegraded oil reservoir which Meren facility is close to. The diversity and composition of the microbial community in Meren production well and produced water samples (1N4, IN5 and IN6) are similar. The predominant microbial flora includes an actinobacteria of the genus Dietzia; this genus seems not to be popular in oil fields and as such its originality cannot be verified. Others include the family Rhodocyclaceae, genus Desulfobulbus, Desulfuromonas and Spirochaeta among the bacterial community and Methanocalculus, Methanolinea, Methanomicrobiaceae, Methanosaeta and Methanolobus among the archaeal community as indicated in Table 3. Most of these organisms are indigenous to oil fields and some have been associated with souring and corrosion in the past (Dahl et al., 2008; Pham et al., 2009; Wilms et al., 2006; Li and Hendry, 2008; Okoro et al., 2014).
In summary, the pyrosequencing survey of the two oil facilities investigated indicate that sample IN1 was dominated by sequences affiliated with Euryarchaeota (44%), and Proteobacteria (39%) indicating methanogenesis and sulphate reduction while 1N2 was dominated by sequences affiliated with Proteobacteria (82%) indicating only sulphate reduction. Other samples and their respective dominant phyla include; 1N3 (Euryarchaeota, 70.79%), IN4 (Euryarchaeota, 51.78% and Proteobacteria, 38%), IN5 (Euryarchaeota, 52% and Proteobacteria, 28%), and IN6 (Euryarchaeota, 62.6%). It has been advanced that both Proteobacteria and Euryarchaeota have capabilities for sulphate reduction (Youssef et al., 2009; Magot et al., 2000); therefore, it is expected that the major metabolic activities in these fields where Proteobacteria and Euryarchaeota are present will be sulphate reduction where sulphate is present but in the absence of sulphate or nitrate, water mediated fermentation of oil organics to methane and carbon dioxide becomes a dominant process (Grabowski et al., 2005; Gray et al., 2011; Pham et al., 2009; Youssef et al., 2009; Zengler et al., 1999). Laboratory investigation using weight loss method and microbial activity showed that the microbial communities from both Escravos and Meren samples were able to induce corrosion and souring.
In conclusion, we advance that this investigation on microbial diversity and metabolic processes of Escravos oil processing and loading facility and Meren offshore fields after routine biocide treatment have expanded substantially our knowledge on the extent of microbial diversity in these fields with potential to induce souring and corrosion despite regular biocide treatments and have also high lightened the abundance and complexity of microbial community that have potential to tolerate biocides and initiate souring and bio-corrosion in these fields. Future research efforts should therefore focus on the biocidal efficacy of the biocides currently used to mitigate corrosion and souring related problems in these fields.
The authors have not declared any conflict of interests.
REFERENCES
Adetoba AL (1985). Meren Field Offshore Treating Facilities for Sea Water Injection. SPE PAPER 14465 presented at the 60th annual technical conference and exhibition of the society for petroleum engineers held in Las Vegas, NV. September 22-25, 1985.
|
|
American Public Health Association (APHA) 1992. Standard Methods for the Examination of Water and Waste Water. 18th ed. Washington, D.C. American Public Health Association pp. 439-440.
|
|
|
Dahle H, Garshol F, Madsen M, Birkeland NK (2008). Microbial community structure analysis of produced water from a high temperature North Sea Oil Field. Antonie Van Leeuwenhoek 93:37-49.
Crossref
|
|
|
Gittel A, Sorenson KB, Skorhus TL, Ingvorsen K, Schramm A (2009). Prokaryotic community structure and sulphate reducer activity in water from high temperature oil reservoirs with and without nitrate treatment. Appl. Environ. Microbiol. 77(22):7086-7096.
Crossref
|
|
|
Grabowski A, Nercessian O, Fayoke F, Blanchet D, Jeanthon C (2005). Microbial diversity in production waters of a low temperature biodegraded oil reservoirs. FEMS Microbiol. Ecol. 54:427-443.
Crossref
|
|
|
Gray ND, Sherry A, Grant RJ, Rowen AK, Hubert CR, Callbeck CM, Aitken CM, Jones DM, Adams JJ, Larter SR, Head IM (2011). The qualitative significance of Syntrophaceae and Syntrophic partnership in Methanogenic degradation of crude oil alkanes. Environ. Microbiol. 13:2957-2975.
Crossref
|
|
|
Grigoryan AA, Cornish SL, Buziak B, Lin S, Cavallaro A, Arensdorf JJ, Voordouw G (2008). Competitive oxidation of volatile fatty acids by sulphate and nitrate reducing bacteria from oil field in Argentina. Appl. Environ. Microbiol. 74:4324-4335.
Crossref
|
|
|
Head IM, Jones DM, Larter SR (2003). Biological activity in the deep subsurface and the origin of heavy oil. Nature 426:344-352.
Crossref
|
|
|
Hubert C, Nemati M, Jenneman G, Voordouw G (2005). Corrosive risk associated with microbial souring control using nitrate or nitrite. Appl. Microbiol. Biotechnol. 68:272-282.
Crossref
|
|
|
Huson D, Richter D, Rausch C, Dezulian T, Franz M, Rupp R (2007). Dendroscope: an interactive viewer for large phylogenetic trees. BMC Bioinform. 8:460.
Crossref
|
|
|
Jan-Roblero J, Romero JM, Amaya M, Le Borgne S (2004). Phylogenetic characterisation of a corrosive consortium isolated from a sour gas pipeline. Appl. Microbiol. Biotechnol. 64:862-867.
Crossref
|
|
|
Lake LW (1989). Enhanced oil recovery. Prentice Hall, Englewood Cliffs (pub). 233 p.
|
|
|
Li D, Hendry P (2008). Microbial Diversity in Petroleum Reservoirs. Microbiol. Aust. 29:25-27.
|
|
|
Li D, Midgley DJ, Ross PS, Oytan Y, Abel GC, Vock H, Daud WA, Hendry P (2012). Microbial biodiversity of a Malaysian oil field and a systematic comparison with oil reservoir worldwide. Arch. Microbiol. 194:513-523.
Crossref
|
|
|
Li H, Yang SZ, Mu BZ, Rong ZF, Zhang J (2006). Molecular analysis of a bacterial community in a continental high temperature and water flooded petroleum reservoir. FEMS Microbiol. Lett. 257:92-98.
Crossref
|
|
|
Li H, Yang SZ, Mu BZ, Rong ZF, Zhang J (2007). Molecular phylogenetic diversity of a microbial community associated with high temperature petroleum reservoir at an offshore field. FEMS Microbiol. Ecol. 60:74-84.
Crossref
|
|
|
Lumley DE, Delorme G, Adeogba AA, Bee MF (2000). Meren Field Nigeria: A 4D Seismic Case Study. Paper 12098-MS presented at the Offshore Technology Conference. May 1-4, 2000. Houston Texas.
Crossref
|
|
|
Magot M (2005). Indigenous microbial communities in oil fields. In. Petroleum Microbiology. Ollivier B and Magot M (eds). ASM Press,Washington DC (pub). pp. 21-33.
Crossref
|
|
|
Magot M, Ollivier B, Patel B (2000). Microbiology of Petroleum Reservoirs. Antonie van Leeuwenhoek 77:103-116.
Crossref
|
|
|
Nazina TN, Griroryan AA, Feng QX, Shestakova NM, Babich TL, Pavlova NK (2007). Microbiological and Production Characteristics of the high temperature Kongdian petroleum reservoir revealed during field trial of biotechnology for the enhancement of oil recovery. Microbiology 76:297-309.
Crossref
|
|
|
Nemati M, Jenneman GE, Voordouw G (2001). Mechanistic study of microbial control of hydrogen sulphide production in oil reservoirs. Biotechnol. Bioeng. 74:424-434.
Crossref
|
|
|
Offshore Technology Conference (OTC) (2002). Focus on the Nigerian Oil and Gas industry. A publication of Offshore technology conference, Houston Texas, 2002.
|
|
|
Okoro C, Smith S, Chiejina L, Lumactud R, An D, Park HS, Voordouw J, Lomans BP, Voordouw G (2014). Comparison of microbial communities involved in souring and corrosion in offshore and onshore oil production facilities in Nigeria. J. Ind. Microbiol. Biotechnol. 41:665-678.
Crossref
|
|
|
Okoro CC (1999). Microbial degradation of hydrocarbons in produced water from crude oil production operations at Escravos Tank Farm. Ph.D. Thesis. University of Lagos. 226 p.
|
|
|
Okoro CC (2010). Microbiological Impacts of Produce Water discharges In Nearshore shallow Marine waters near Chevron's Escravos Tank Farm, Nigeria. Afr. J. Microbiol. Res. 4(13):1400-1407.
|
|
|
Orphan VJ, Taylor LT, Hafenbradl D, Delong EF (2000). Culture dependent and culture independent characterization of microbial assemblages associated with high temperature petroleum reservoirs. Appl. Environ. Microbiol. 66:700-711.
Crossref
|
|
|
Park HS, Chatterjee I, Dong X, Wang SH, Sensen CW, Caffrey SM, Jack TR, Boivin J, Voordouw G (2011). Effect of sodium bisulfite injection on the microbial community composition in a brackish-water-transporting pipeline. Appl. and Environ. Microbiol. 77: 6908-6917.
Crossref
|
|
|
Pham DV, Hnatow LL, Zhang S, Fallon DR, Jackson SC, Tomb JF, DeLong EF, Keeler SJ (2009). Characterizing microbial diversity in production water from an Alaskan Mesothermic Petroleum Reservoir with two independent molecular methods. Environ. Microbiol. 11(1):176-187.
Crossref
|
|
|
Pruesse EC, Quast K, Knittel B, Fuchs W, Ludwig J, Peplies J, Glöckner FO (2007). SILVA: a comprehensive online resource for quality checked and aligned ribosomal RNA sequence data compatible with ARB. Nucleic Acids Res. 35:7188-7196.
Crossref
|
|
|
Schloss PD, Westcott SL (2011). Assessing and improving methods used in OTU-based approaches for 16S rRNA gene sequence analysis. Appl. Environ. Microbiol. 77: 3219-3226.
Crossref
|
|
|
Schloss PD, Westcott SL, Thomas R, Hall JR, Hartmann M, Hollister EB, Lesniewski RA, Oakley BB, Parks DH, Robinson CJ, Sahl JW, Stres B, Thallinger GG, Van Horn DJ, Weber CF (2009). Introducing mothur: open-source, platform-independent, community-supported software for describing and comparing microbial communities. Appl. Environ. Microbiol. 75:7537-7541.
Crossref
|
|
|
Soh J, Dong X, Caffrey SM, Voordouw G, Sensen CW (2013). Phonenix 2. A locally installable large scale 16S rRNA gene sequence analysis pipeline with web interface. J. Biotechnol. 167:393-403.
Crossref
|
|
|
Trüper HG, Schlegel HG (1964). Sulfur metabolism in Thiorhodanceae. Quantitative measurements in growing cells of Chromatium okehii. Antonie van Leewenhoek 30:225-238.
Crossref
|
|
|
Van der Kraan GM, Bruining J, Lomans BP, van Loosdrecht MC, Muyzer M (2009). Microbial diversity of an oil water processing site and its associated oil field: The possible role of microorganisms as information carriers from oil associated environments. FEMS Microbiol. Ecol. 71:428-443.
Crossref
|
|
|
Vance I, Trasher DR (2005). Reservoir souring: Mechanism and Prevention. In. Petroleum Microbiology. B. Ollivier and M. Magot (eds). ASM Washington DC (pub). pp. 123-142.
Crossref
|
|
|
Voordouw G (2011). Production related Petroleum Microbiology: Progress and Prospects. Curr. Opin. Biotechnol. 22:401-405.
Crossref
|
|
|
Voordouw G, Armstrong SM, Reiner MF, Foults B, Telang AJ, Shen Y, Gevertz D (1996). Characterisation of 16S rRNA genes from oil field microbial communities indicates the presence of a variety of sulfate reducing, fermentative and sulfide ozidizing bacteria. Appl. Environ. Microbiol. 62:1623-1629.
|
|
|
Wilms R, Sass H, Kopke B, Koster J, Cypionka H, Englen B (2006). Specific Bacterial Archaeal and Eukaryotic communities in Tidal Flat Sediments along a vertical profile of several meters. Appl. Environ. Microbiol. 72(4):2756-2764.
Crossref
|
|
|
Youssef N, Elshahed MS, McLnerney MS (2009). Microbial process in oil fields; Culprits, Problems and Opportunities. In. Advances in applied microbiology. Laskin, AI, Sariaslani S, Gadd, GM (eds). ASM press (Pub.). 66:141-251.
|
|
|
Yue JC, Clayton MK (2005). A similarity measure based on species proportions. Commun. Stat. Theor. Methods 34:2123-2131.
Crossref
|
|
|
Zengler K, Richnow HH, Rossello-Mora R, Michaelis W, Widdel F (1999). Methane formation from long chain alkanes by anaerobic microorganisms. Nature 401:266-269.
Crossref
|
|
|
Zhu XY, Lubeck J, Kilbane JJ (2003). Characterisation of Microbial communities in Gas Industry Pipelines. Appl. Environ. Microbiol. 69(9):5354-5363.
Crossref
|
|