ABSTRACT
Phyllosphere bacteria plays important role in mitigating biotic and abiotic stress. This study aimed to use phyllosphere bacterium of rice to enhance drought tolerance. Bacterial isolates obtained from rice phyllosphere were investigated for their plant growth promoting activities and role in alleviation of drought stress in rice. The isolates were identified as Bacillus altitudinis FD48, Bacillus pumilus FS20 and Bacillus aquimaris MD02 based on 16S rRNA gene sequence. B. altitudinis FD48 was found to produce indole acetic acid (IAA) (2.82 µg/ml) compared to other two isolates even under PEG induced drought conditions. However, under normal conditions, B. altitudinis FD48 produced 8.0 µg/ml. Quantitative estimation of 1-aminocyclopropane-1-carboxylate (ACC) deaminase activity was found to be 192 n moles a-ketobutyrate mg-1 h-1 and positive for accD gene. B. altitudinis FD48 increased the root and shoot length of rice under in vitro conditions and also improved the germination percentage of rice seeds at different concentration of PEG 6000. Inoculation of rice with plant growth promoting, drought tolerant B. altitudinis FD48 increased relative water content, chlorophyll stability index and membrane stability index compared to control (uninoculated plants) when the plants were subjected to drought by discontinuing water for 8 days after 30 days of germination. Similarly, rice treated with B. altitudinis increased proline content, phenolics content, catalase activity and reduced malondialdehyde (MDA) content in plants. Ethylene emission was significantly reduced by B. altitudinis FD48 inoculation under drought condition when compared with control. This study suggests that the isolate B. altitudinis FD48 may be used at field level to mitigate drought stress in rice.
Key words: Proline content, drought, 1-aminocyclopropane-1-carboxylate (ACC) deaminase, plant growth promoting bacteria, phenolics content, indole acetic acid (IAA).
Rice is a cereal crop which shapes the culture and economy of India and is cultivated in about 43.5 M ha area but with relatively low average productivity. Abiotic factors like drought, flood and salinity have adverse effect on rice production (Singh et al., 2016). Drought is one of the major abiotic stresses affecting the yield of the crop. Being a drought sensitive crop, rice exhibits deleterious effects when exposed to drought at critical growth stages such as panicle initiation, anthesis and grain filling (Weisburg et al., 1991). The responses of plants to drought are complex and affect several components. It not only affects cell water potential but also induces closure of stomata, decrease in photosynthesis, nitrate assimilation and various anabolic enzyme reactions (Zhang et al., 2010). It also induces the generation of active oxygen species which in turn cause lipid peroxidation and consequently membrane injury, protein degradation, enzyme inactivation, pigment bleaching and disruption of DNA strands (Pompelli et al., 2010).
Various tolerance mechanisms have been suggested on the basis of biochemical and physiological changes related to drought (Shukla et al., 2012). Recent studies have indicated that plant associated bacteria can help to withstand abiotic stresses more efficiently (Chauhan et al., 2015). If such microorganisms have the ability to promote growth, they become extra beneficial. Plant growth promoting bacteria (PGPB) can mitigate the impact of abiotic stresses on plants through a process called induced systemic tolerance (IST), which includes bacterial production of cytokinins, production of antioxidants and degradation of the ethylene precursor 1-aminocyclopropane-1-carboxylate (ACC) by bacterial ACC deaminase. Rhizosphere colonizing bacteria were well studied for their role in stress tolerance (Sandhya et al., 2011), but few studies were focused on phyllosphere bacterial amelioration of abiotic and biotic stress in plants.
Most of the research on phyllosphere bacteria has been paying attention on individual bacterial isolates using culture dependent methods with respect to their role in plant protection by pathogen suppression (Delmotte et al., 2009) and in plant growth promotion (PGP) (Papen et al., 2002) or through the production of beneficial substances such as 1-aminocyclopropane-1-carboxylate deaminase (ACCD). Phyllosphere colonizing Methylobacterium were reported to produce phytohormones like cytokinin and auxins (Madhaiyan et al., 2005) and stress response enzyme ACC deaminase (Chinnadurai et al., 2009). ACCD is responsible for the cleavage of the plant ethylene precursor, ACC, into ammonia and α-ketobutyrate (Honma and Shimomura, 1978).
By decreasing ACC levels in plants, ACC deaminase producing organisms reduce plant ethylene level. During stress condition, ethylene is present in high concentration and may cause damages to plant (Glick et al., 2007). Bacillus species is a major inhabitant of rhizosphere region which also exist as epiphytic microorganisms with plant growth promoting activities (Idris et al., 2004) and stress tolerance ability (Chandramohan and Mahadevan, 1968). Rice inoculated with Bacillus spp. was found to alleviate oxidative damage by improving plant growth and activating antioxidant defense systems, thereby improving the stability of membranes in plant cells under drought condition (Gusain et al., 2015). The objective of this study was to identify the role of Bacillus spp. in alleviating drought stress by altering the growth and biochemical parameters of rice.
Organism source and molecular characterization
The isolates FD48, FS20 and MD02 used in the study were obtained from Department of Agricultural Microbiology, Directorate of Natural Resources Management, Tamil Nadu Agricultural University, Coimbatore. The aforementioned isolates were previously isolated from the phyllosphere of rice. The genomic DNA from the three isolates was extracted using the standard protocol of hexadecyl-trimethyl ammonium bromide (CTAB) method as given by Melody (1997) with minor modifications. Nearly-full length of 16S rRNA gene was amplified from the genomic DNA from the three isolates using FD1 (5’AGA GTT TGA TCC TGG CTC AG 3’) and RP2 (5’ACG GCT ACC TTG TTA CCA CTT 3’) primers (Weisburg et al., 1991). A total of 20 µL of reaction volume contains 50 ng of genomic DNA, 0.2 mM of each dNTP, 1 μM of each primer, 2.5 mM of MgCl2 and 1 U of Taq DNA polymerase (all from Bangalore Genei, India) and the buffer supplied with the enzyme.
PCR amplification was performed in a thermocycler (Eppendorf Master Cycler, Germany) using conditions as follows: initial denaturation at 95°C for 10 min; 35 cycles consisting of 94°C for 1 min (denaturation); 55°C for 1 min (annealing); 72°C for 1 min (primer extension) and final extension at 72°C for 10 min. The cloned product was sent for sequencing to Bioserve technologies and sequenced through using ABI prism terminator cycle sequencing ready reaction kit and electrophoresis of the products were carried out on an Applied Biosystems (Model 3100) automated sequencer. The identity of 16S rDNA sequence was established by performing a similarity search against the GenBank database (website: http://www.ncbi.nih.gov/BLAST). The phylogenetic tree was constructed by neighbour-joining method (Saitou and Nei, 1987) using MEGA6 software.
Plant growth promoting activities of the isolates
Indole acetic acid (IAA) production
One millilitre of the culture at exponential stage was inoculated in 100 ml LB medium containing filter sterilized L-tryptophan (0.01% w/v) (Chandramohan and Mahadevan, 1968). The flasks were incubated at room temperature for 7 days. The cells were harvested by centrifugation at 10,000 rpm for 5 min and the supernatant was collected and concentrated to 25 ml. The culture filtrate was adjusted to pH 2.8 with 1N HCl. Equal volume of ice cold (4°C) diethyl ether and culture filtrate was added in a separating funnel, shaken well and allowed to stand in dark for 4 h with intermittent shaking. The aqueous phase was separated from organic phase and the extraction was repeated 3 times. Discarding the aqueous phase, the organic phases were pooled and evaporated to dryness in the dark. The residue was dissolved in 2 ml of absolute methanol and analyzed in HPLC (Thermo, Spectrasystem UV 2000). The quantity of IAA produced was estimated using Salper’s reagent (1 ml of 0.5N FeCl3 mixed in 50 ml of 35% perchloric acid) (Gorden and Paleg, 1957)..
ACC deaminase activity
1-Aminocyclopropane 1-carboxylate deaminase (ACCD) activity was assayed according to a modified method (Honma and Shimomura, 1978) which measures the amount of a-ketobutyrate produced when the enzyme ACCD cleaves ACC to a-ketobutyrate. The bacterial culture grown in LB medium for 24 h were transferred to minimal medium (DF salt medium) (Dworkin and Foster, 1958) with ACC as the sole source of nitrogen. Cultures were incubated overnight in a shaking water bath at 200 rpm at room temperature. The biomass accumulated was harvested by centrifugation at 8000 rpm for 10 min at 4°C. The supernatant was removed and the cells were washed with 5 ml DF salts minimal medium. Following an additional centrifugation for 10 min at 8000 rpm at 4°C, the cells were suspended in 7.5 ml DF salts minimal medium in a fresh culture tube.
Just prior to incubation, 45 ml of 0.5 M ACC (filter sterilized) was added to the cell suspension to obtain a final concentration of 3.0 mM. The bacterial cells were returned to the shaking water bath at room temperature for overnight incubation. The bacterial cells were harvested by centrifugation at 8000 rpm for 10 min at 4°C. The supernatant was removed and cells were washed by suspending the cell pellet in 5 ml of 0.1M Tris-HCl, pH 7 and transferred to a 1.5 ml microcentrifuge tube. The contents were centrifuged at 16000 rpm for 5 min and the cell pellet was suspended in 600 ml of 0.1M Tris-HCl, pH 8.5. Thirty microlitres of toluene was added to the cell suspension and vortexed at the highest setting for 30 s and 100 ml aliquot of the toluenized cells was set aside and stored at 4°C for protein assay at a later time.
The protein concentration of toluenized cells was determined (Chakraborty et al., 2013). The remaining toluenized cell suspension was used for ACCD activity assay. Two hundred microlitres of the toluenized cells were placed in a fresh 1.5 ml microcentrifuge tube and 20 ml of 0.5M ACC was added to the suspension, briefly vortexed and then incubated at 30°C for 15 min. Following the addition of 1 ml of 0.56 M HCl, the mixture was vortexed and centrifuged for 5 min at 16000 rpm at room temperature. One millilitre of the supernatant was vortexed with 800 ml of 0.56 M HCl and 300 ml of the 2, 4-dinitrophenyl hydrazine reagent was added to the glass tube, the contents were vortexed and then incubated for 30 min at 30°C. Following the addition and mixing of 2 ml of 2N NaOH, the absorbance was measured at 540 nm.
ACC deaminase gene amplification
The screening of ACC deaminase containing isolates from rice phyllosphere was done based on the amplification of ACC deaminase gene. Presence of accD gene (700 bp) in the phyllosphere isolates was detected (Saravanakumar and Samiyappan, 2007) using the primers ACCDF 5’ ATG AAC CTG CAA CGA TTC 3’and ACCDR 5’ TCA GCC GTC TCG GAA GAT 3’.
Drought tolerance level of the isolates
The effects of drought on the growth of isolates were studied using polyethylene glycol MW 6000 (PEG) (M/S Hi-Media, Mumbai, India) at different concentration ranging from 0 to 25%. The isolates were inoculated in LB broth containing different concentration of PEG (10, 15, 20, and 25%) and incubated at 28°C for two days. The bacterial growth was measured spectrophotometrically at OD 660 nm (Abdel-Salem et al., 2010).
IAA production under stress condition
LB broth amended with L-tryptophan was prepared at different level of PEG concentrations (10, 15 and 20%) and inoculated with the isolates. The production of IAA by the isolates was measured under increasing PEG concentration (Uma Maheshwari et al., 2013). The supernatant was collected by centrifuging at 10,000 rpm for 20 min. Two millilitres of supernatant was mixed with two drops of Orthophosphoric acid and 4 ml of Salkowski reagent. Tubes were incubated at room temperature for 25 min and OD value was measured at 535 nm (Gorden and Paleg, 1957).
PEG induced drought stress on seed germination of rice
The rice seeds were surface sterilized in 0.1% HgCl2 for 2 min rinsed five times with sterile water. The seeds were then treated with overnight grown cultures of FD48, FS20 and MD02. Methylobacterium species (Pink Pigmented Facultative Methylotrophs, PPFM) was maintained as standard and seeds treated with sterile water as control. The seeds were then placed on a filter paper in Petri dishes, moistened with 10, 15 and 25% of PEG (MW 6000) concentration corresponding to water potential of -1.4, -2.9 and -6.7 bars. The Petri plates were sealed with Parafilm to prevent evaporation and kept at room temperature. Seeds were considered germinated when the radical had extended for at least 2 mm. Germination percentage and root length were measured after 24, 48 and 72 h (Muscolo et al., 2014).
Paper towel culturing for rice
Overnight water soaked paddy seeds (cultivar ADT 36) were surface sterilized with sodium hypochlorite with 3% for 3 min followed by several washes with sterile distilled water. The seeds were placed on 2% sucrose agar plates spread with bacterial culture (T1 - control, T2 - MD02, T3 - PPFM, T4 - FD48, T5 - FS20) and incubated at room temperature for germination. The sprouted seeds (10 numbers) were placed in germination paper towels and kept in sterile polythene pouches, in a stand. The whole experimental set up was provided with light and dark at 12 h intervals. Watering was done using sterile distilled water at 4 days interval. Along with ACC deaminase producing bacterial inoculants as cell suspensions in distilled water (109 cfu per ml) was sprayed at 7, 14, and 21 days. After 25 days of inoculation, the root length, shoot length, root dry weight and shoot dry weight of the seedlings were recorded.
Pot-culture experiment
To assess the impact of bacterial inoculant on growth and drought tolerance of rice, an experiment was carried out in the green house of Department of Agricultural Microbiology, Tamil Nadu Agricultural University, Coimbatore. Wetland soil used for pot culture studies was sterilized and filled in pots at 5 kg pot-1. The bacterial culture was grown in LB broth and Methylobacterium spp. (PPFM) in AMS medium till the population reached to 1010 cells ml-1. Overnight water soaked paddy seeds (cultivar ADT 36) were surface sterilized with sodium hypochlorite for 3 min followed by several washes with sterile distilled water. Surface sterilized seeds were imbibed with above said bacterial inoculants for 1 h and shade dried before sowing. Intermittent foliar spraying of bacterial culture was also carried out.
Paddy seeds were sown directly in the pot, maintaining three plants per pot and the treatments were replicated seven times in a completely randomized block design. Soil water content (SWC) was estimated (Cha-um et al., 2012) using the formula: (FW-DW/AvFW-DW) × 100, where FW is the fresh weight of soil, DW is the dry weight of soil (at 110°C), AvFW is the average fresh weight of the soils on day 0 of the experiment (Ogbaga et al., 2014). After 30 days of germination, drought stress was induced by discontinuing watering for eight days (at 45% of water holding capacity). Observations were taken from non-stressed and drought stressed plants after 8 days of drought stress. Treatment details are T1, Control; T2, Bacillus altitudinis (FD48) and T3, Methylobacterium spp. (PPFM).
Relative water content (RWC)
Relative water content was determined (Schonfeld et al., 1988) where fresh weights for twenty discs from the youngest fully expanded leaf were determined within 2 h after excision. Turgid weight was obtained after soaking the discs for 16 to 18 h in distilled water. After soaking, discs were quickly and carefully blotted dry with tissue paper prior to determination of turgid weight. Dry weight was obtained after drying the discs sample for 72 h at 70°C. Relative water content was calculated from the following equation:
RWC = [(fresh weight - dry weight) / (turgid weight - dry weight)] × 100
Chlorophyll stability index
Two clean glass tubes were taken and 5 g of representative leaf sample was placed in them with 20 ml distilled water. One tube was then subjected to heat in water bath at 56°C for exactly 30 min. Other tube was kept as control. The leaves were then ground in a mortar for 5 min with 10 ml of 80% acetone. The contents were centrifuged at 3000 rpm for 10 min and the supernatant was made up to 25 ml using 80% acetone. The total chlorophyll content was measured at 652 nm in a spectrophotometer (Murthy and Majumdar, 1962):
and expressed as mg total chlorophyll per gram of fresh weight, V=Final volume of acetone extract, and W=Fresh weight in gram. The chlorophyll stability index is the ratio of total chlorophyll content of the treated sample to the untreated samples and expressing in percentage.

Membrane stability index
Membrane stability index (MSI) of fresh leaves was determined (Bailly et al., 1996; Kaya et al., 2003). The conductivity of solution was measured using a conductivity bridge meter using the formula:
MSI =1- C1/C2
Where, C1- conductivity at 40°C and C2- conductivity at 100°C.
Measurement of biochemical parameters
Assessment of biochemical (proline, MDA, total phenolics, and Catalase activity) responses of rice were carried out on fresh plant material that was immediately extracted and assayed according to the appropriate methods listed subsequently. Proline content was determined by the method of Bates et al. (1973). Total phenolics were estimated (Malik and Singh, 1980) using Folin reagent and the absorbance was measured at 660 nm against each blank. Catalase activity (CAT; EC 1.11.1.6) was determined (Gopalachari, 1963). The activity of enzyme was expressed as µg of H2O2 g-1 min-1. For estimating MDA content, a quantity of 250 mg plant sample was homogenized in 5 ml 0.1% Trichloro acetic acid (TCA). The homogenate was centrifuged at 10000 g for 5 min. To 1 ml aliquot of the supernatant 4 ml of 20% TCA containing 0.5% thio barbituric acid (TBA) were added. The mixture was heated at 95°C for 30 min, quickly cooled in an ice bath and centrifuged at 10000 rpm for 10 min.
The absorbance of the supernatant was read at 532 and 600 nm (Heath and Packer, 1968). For the estimation of ethylene, the plants were uprooted after 40 days, washed with sterile distilled water until free from soil and ethylene production was measured by gas-chromatography (GC) (Chinnadurai et al., 2009). The whole plant was packed in 120 ml vials and the vials were capped with a rubber septum and following 4-h incubation, 1 ml of headspace was sampled for each vial and the ethylene content was measured in a GC packed with poropak-N column at 70°C, equipped with a flame ionization detector. The carrier gas was N2 at the flow rate of 60 ml min-1 and the combustion gas was H2 at the flow rate of 50 ml min-1 with the combustion-supporting gas air at the flow rate of 500 ml min-1. The amount of ethylene detected through GC were manipulated and expressed as µmol of ethylene evolved per g of fresh plant in 1 h.
Statistical analysis:
Data were subjected to analysis of variance (ANOVA) using a statistical computer package AGRES to determine whether the treatments effects were significant. The treatment and variety means were separated using the least significant differences (LSD) test.
Molecular characterization of rice phyllosphere isolates
Phylogenetic tree was generated by using the phylogeny program and neighbor-joining method. The 16S rRNA sequences from Bacillus genus were used for diversity studies (the bacterial species and accession number are given in Figure 1).
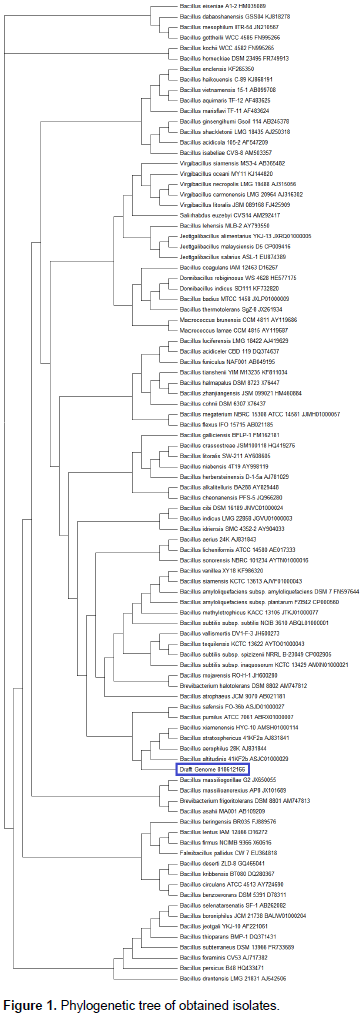
Plant growth promoting activities of the isolates
Among the isolates, the highest amount of IAA was produced by B. altitudinis (FD48) (8.14 µg/ml) followed by Bacillus pumilus (FS20) (5.64 µg/ml) and Bacillus aquimaris (MD02) (4.43 µg/ml). PEG treatment at 15, 20 and 25% reduced the level of IAA production in all isolates compared to control (without PEG). Among the three isolates tested, FD48 produced IAA (2.82 µg ml-1) at higher concentration of PEG (25%) whereas isolates FS20 and MD02 did not produce detectable amount of IAA at same level (Figure 2). Among the three phyllosphere isolates, B. altitudinis FD48 was able to grow on DF minimal broth supple-mented with 3 mM ACC as sole nitrogen source, whereas B. pumilus FS20 and B. aquimaris MD02 showed no growth. All the three isolates showed growth on DF minimal broth supplemented with 0.2% (NH4)2SO4. ACC deaminase activity of B. altitudinis FD48 was quantified and found to produce 192.5 n moles a-ketobutyrate mg-1 h-1. An amplification of 700 bp of accD gene was observed. This PCR based screening confirmed the presence of accD gene in B. altitudinis FD48.
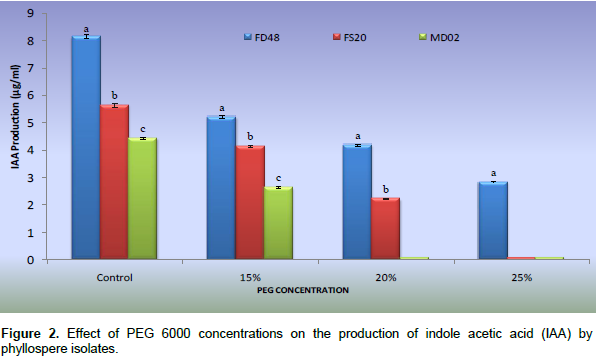
Drought tolerance level of isolates
The effect of drought towards the growth of all the three isolates were studied using LB broth supplemented with PEG. All the isolates grew well in LB broth without PEG. As the concentration of PEG increased, the growth decreased. The isolate B. altitudinis FD48 was able to grow at 25% PEG 6000 (0.55 OD) whereas the isolates B. pumilus FS20 (0.44 OD) and B. aquimaris MD02 (0.36 OD) were able to grow only upto 15% PEG 6000 concentration (Table 1).

Germination percentage and root length of rice seeds treated with phyllosphere bacterial cultures
Rice germination was decreased as the concentration of PEG increased, that is, 0 to 25%. However, the effect of PEG was greatly reduced by treating rice seeds with bacterial cultures viz., B. altitudinis FD48, B. pumilus FS20, B. aquimaris MD02 and Methylobacterium spp. (PPFM) (Table 2). Among the isolates tested, B. altitudinis FD48 greatly enhanced the germination percentage at 25% levels of PEG compared to uninoculated control. Root length was decreased as the concentration of PEG increased, that is, 0 to 25%. However, culture treated seeds recorded better root growth than the uninoculated control at all concentration of PEG. At higher concentration of PEG (25%), Ba. altitudinis FD48 treated seeds showed 0.56 cm root length followed by Methylobacterium spp. (PPFM) treated seeds (0.42 cm) whereas uninoculated control showed only 0.22 cm root length (Table 2).
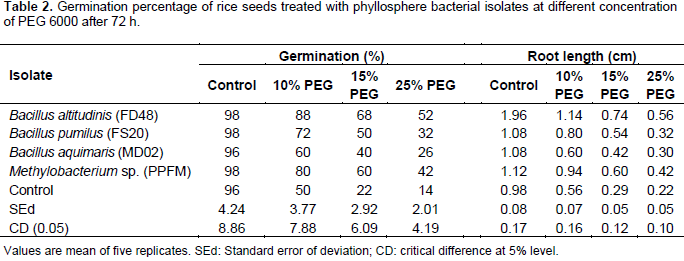
Effect of phyllosphere isolates on biometric characteristics of rice seedlings (cultivar- ADT 36) after 25 days of inoculation
Phyllosphere bacterial isolates with plant growth promoting activities were selected for gnotobiotic studies. Among the three isolates the highest shoot length was observed in seedlings treated with B. altitudinis FD48 (9.5 cm) which was significantly superior to Methylobacterium spp. (PPFM) (8.51 cm). The uninoculated control recorded the lowest shoot length (5.23 cm) while higher root length was recorded in B. altitudinis FD48 treated seedlings (15.23 cm) followed by Methylobacterium spp. (PPFM) treated seedlings (14.01 cm). The least root length was observed in control (6.76 cm). The root dry weight was the highest in B. altitudinis FD48 treatment (3.77 mg) followed by Methylobacterium spp. (PPFM) treatment (3.37 mg). The least RDW was observed in control (1.75 mg). B. altitudinis FD48 (5.11 mg) showed the highest shoot dry weight followed by Methylobacterium spp. (PPFM) (4.43 mg). The control recorded least shoot dry weight (2.89 mg) (Table 3).
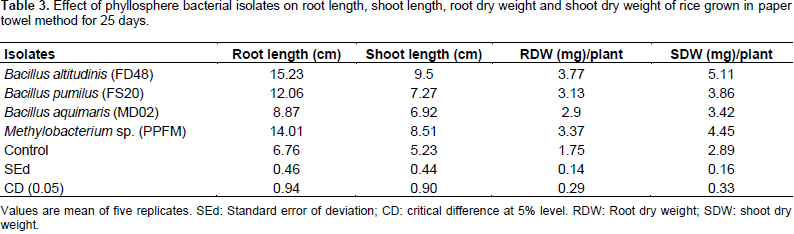
Crop response study to evaluate the efficiency of phyllosphere bacteria in alleviation of drought stress under in vitro conditions
The pot culture experiment was carried out at Department of Agricultural Microbiology, Tamil Nadu Agricultural University, Coimbatore to evaluate the efficiency of phyllosphere bacteria on the growth of rice (cultivar- ADT 46) under drought condition. Relative water content (RWC) of plants decreased in response to drought condition. However, culture treated plants were observed to have more RWC compared to control under induced drought condition. B.altitudinis FD48 treated plants showed 69.38% RWC followed by Methylobacterium spp. (PPFM) treated plants (68.61%) whereas the control recorded the lowest RWC (60.53%) (Table 4). Chlorophyll stability index (CSI) of plants decreased in response to drought condition. Drought stressed plants inoculated with B. altitudinis FD48 showed 69.23% CSI followed by Methylobacterium spp. (PPFM) inoculation (68.32%). The chlorophyll stability index of control plants were the lowest (55.4%) under drought condition (Table 4).
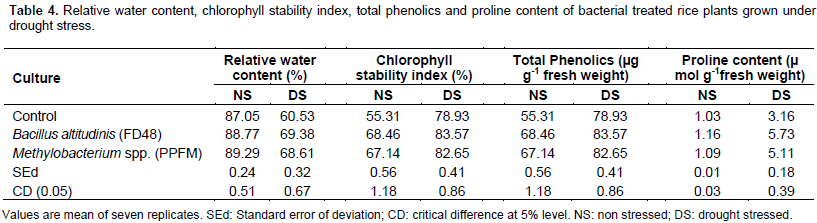
Proline content was significantly influenced by both drought stress and culture treatments. A substantial increase in the amount of free proline was observed in all treatments due to drought stress (Table 4). However, it was interesting to note that B. altitudinis FD48 treated rice plants produced the highest concentration of proline (5.73 µ mol g-1fresh weight) relative to Methylobacterium spp. (PPFM) treated plants (5.11 µ mol g-1fresh weight) and control (3.16 µ mol g-1 fresh weight). The phenolics content of plants in all treatments increased from well watered condition to drought condition. Highest phenol content under drought stress was observed in treatment Bacillus altitudinis FD48 (83.57 µg g-1 fresh weight) followed by Methylobacterium spp. (PPFM) (82.65 µg g-1 fresh weight).
The control plants had the lowest phenol content (78.93 µg g-1 fresh weight) (Table 4). Catalase activity did not differ significantly among the treatments under non stressed condition which indicated the reduced activity of catalase enzyme. The catalase activity increased under drought condition with B. altitudinis FD48 treated plants with significantly higher activity (3.51 µg of H2O2 g-1 min-1) followed by Methylobacterium spp. (PPFM) treated plants (4.01 µg of H2O2 g-1 min-1). The least catalase activity was observed in control (4.88 µg of H2O2 g-1 min-1) (Table 5). MDA content was significantly influenced by drought and culture treatment. The MDA content was higher in untreated (control) plants under drought condition (6.91 n mol/g fresh weight). Methylobacterium spp. (PPFM) treatment recorded 5.81 n mol/g fresh weight of MDA under stressed condition.
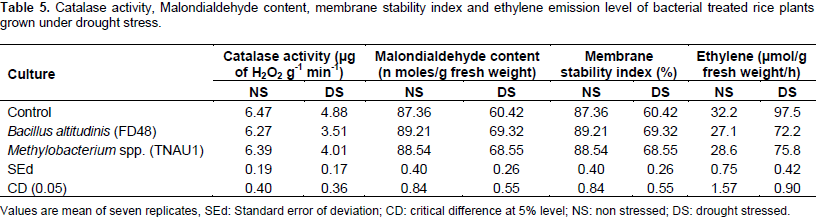
The accumulation of MDA content was lower in treatment B. altitudinis FD48 (4.66 n mol/g fresh weight) revealing reduced accumulation of lipid peroxides under drought stress (Table 5). Limiting watering caused a loss in membrane stability in untreated rice plants and treated plants (Table 5). However, B. altitudinis FD48 treated plants significantly improved membrane stability (69.32%) compared to Methylobacterium spp. (PPFM) treated plants (68.55%) and control (60.42%) (Table 5). The whole plant of rice was used to quantify ethylene emission by gas chromatography. The quantity of ethylene estimated from the plant was expressed by µmol per gram fresh weight of the plant per hour and presented in Table 5. Ethylene emission level was low under non stressed condition and under drought condition the level of ethylene emission increased. However, B. altitudinis FD48 treated plants resulted in low ethylene emission (72.2 µmol/g fresh weight) compared to control (97.5 µmol/g fresh weight) under stressed condition.
Plants are constantly exposed to abiotic stress, such as drought, which is one of the most serious problems associated with plant growth and development. Hence, inoculation with PGPB is most effective under drought-stress environment to increase productivity (Chanway and Holl, 1994). Growth promotion by the PGPB may be attributed to mechanisms such as production of Plant Growth Promoting hormones and other PGP activities (Glick, 1995). The phyllosphere, the interface between the aerial parts of terrestrial plants and the air, represent the largest biological habitat on earth (Delmotte et al., 2009). These bacterial populations are thus sufficiently large to have an important impact on the global biogeochemical processes of nutrient elements (e.g., carbon and nitrogen cycles) as well as the health and physiological characteristics of the plants.
The isolated phyllosphere bacteria were identified based on 16S rRNA gene sequencing. Further, the multiple plant growth promoting traits and tolerance level to drought stress conditions were examined. Influences of phyllosphere bacterial inoculation on growth of rice under drought condition were also studied in vitro conditions. Occurrence of Bacillus spp. in the phyllosphere of vegetables was reported earlier (Zhang et al., 2010). Presence of leaf associated culturable Bacillus spp. on tobacco leaves and their quorum quenching ability has been reported (Ma et al., 2013). B. altitudinis isolated from rice phyllosphere, showed antagonistic activity against Rhizoctonia solani (De Costa et al., 2008). It was reported that IAA production can increase the epiphytic fitness of bacteria (Manulis et al., 1998). At low concentrations, IAA plays an important role in loosening cell wall (Vanderhoff and Dute, 1981) and stimulating the release of saccharides, which serves as nutrient source for epiphytic bacteria and supports their growth (Fry, 1989; Goldberg, 1980).
The results revealed that the accD gene positive isolates could produce a partial amplification (700 bp) of accD. This PCR based detection is relatively easy to screen the isolates than the enzyme assay. Among 3 isolates, only B. altitudinis FD48 showed positive to accD. In earlier studies, the rhizobacteria were reported to be the predominant group of ACC deaminase producers in plants including Enterobacter cloacae (Penrose and Glick, 2001), Rhizobium (Ma et al., 2003), Pseudomonas, Alcaligenes, Bacillus, etc. The ACC deaminase producing Methylobacterium was first isolated from stem tissues of rice and designated as Methylobacterium oryzae (Madhaiyan et al., 2007). The presence of ACC deaminase producing Methylobacterium spp. in rice phyllosphere was reported (Chinnadurai et al., 2009). In this study, ACC deaminase activity of B. altitudinis FD48 was found to produce 192.5 nmoles a-ketobutyrate mg-1 h-1. This result was similar to the findings of Chinnadurai et al. (2009) who reported ACC deaminase activity of Methylobacterium spp. from rice phyllosphere in the range of 190 to 400 nmoles a-ketobutyrate mg-1 h-1.
The inoculated rice seeds showed higher root and shoot growth than the control. FD48 treated seeds performed better when compared with other isolates. This finding is in agreement with earlier studies conducted by Ghosh et al. (2003), Jiang and Lafitte (2007), and Madhaiyan et al. (2006) in different plant species. The increase in root and shoot length and their dry weight may be due to the plant growth promoting activities of the isolates. The isolate B. altitudinis FD48 also supported the germination of rice seeds under different PEG concentration. It has been suggested that production of betaine, an osmolyte by certain bacteria provides a barrier against dehydration (Sleator and Hill, 2002). It is not known, however, how the interaction of plants with such microorganisms improves plant response to drought. B. altitudinis FD48 also showed growth on increasing concentration of PEG 6000 (which induces stress) and proved their ability to withstand drought stress. The results are in agreement with Upadhyay et al. (2012) who reported the growth of Bradyrhizhobial isolates under increasing PEG concentration.
B. altitudinis FD48 reported IAA production at 25% PEG concentration, whereas other isolates did not produce any detectable quantities of IAA with increasing PEG concentration. These results are in confirmation with Uma Maheshwari et al. (2013) and Marulanda et al. (2009). Moreover, the ability of these strains to increase the production of IAA as much as the increased osmotic stress (PEG) in the growing medium would account for their osmotic tolerance. In addition to general plant growth, IAA stimulates stress tolerance because of physical and chemical changes in plant caused by these Plant Growth Promoting Bacteria (Mayak et al., 2004). IAA can improve the root proliferation and help plants to accumulate water from the surrounding environment, thereby improving the response to drought stress. The phyllosphere isolates showed increased content of proline, total sugars and total amino acid under PEG induced drought stress condition when compared with non-stressed condition. But the protein content was less in all isolates under drought stressed condition.
B. altitudinis FD48 performed better than other two isolates under drought condition. These results are in accordance with Sandhya et al. (2010) and Singh et al. (2016). Under stress conditions energy flow of the cells is directed towards protection mechanisms to synthesize osmolytes (sugars, proline, etc) to protect them against fluctuations in osmotic conditions (Timmusk, 2003) and these osmolytes accumulate to higher levels to alleviate stress effects (Rasanen et al., 2004). The accumulated osmolytes enhance the stability of proteins and membrane under water-limiting environments (Kogut and Russell, 1987). However, the concentration of protein was reduced significantly under stress indicating the degeneracy under stress conditions. Trehalose accumulation was also found in B. altitudinis FD48 which is a compatible osmolyte and may result in providing stress tolerance to the isolate. Trehalose accumulation in Azospirillum brasilense improved drought tolerance and biomass in maize treated with the culture (Rodriguez-Salazar et al., 2009).
The inoculation of phyllosphere isolate B. altitudinis FD48 improved the plant growth under drought condition by maintaining relative water content, increased accumulation of proline and phenolics and by enhancing the activity of antioxidant enzymes when compared to control. Under drought stress Relative water content (RWC) declined in inoculated and uninoculated seedlings. However, bacterial inoculation did help seedlings to maintain their Relative water content (RWC) during drought periods. Similar report was made on the use Pseudomonas spp. inoculation to help the maize plants to maintain their relative water content under drought condition (Sandhya et al., 2010). The mechanism behind the increased Relative water content (RWC) when treated with PGPB is yet to be elucidated. Some studies predict that this may be a result of bacterial absicisic acid which results in closure of stomata (Casanovas et al., 2002). Drought stress caused a disturbance in membrane permeability and expressed by an increase in solute leakage (Premchandra et al., 1990; Deshmukh et al., 1991).
The results on MSI showed a decreasing trend as the time without water prolonged. The leakage was higher in untreated plants than B. altitudinis FD48 treated plants indicating severe membrane damage in the former under drought stress. Bacillus spp. treated maize plants reduced the MDA content under stress condition compared to control (Sandhya et al., 2010). The higher leakage of solutes was probably due to enhanced H2O2 accumulation and lipid peroxidation under oxidative stress (Dionisio-Sese and Tobita, 1998). The plasma membrane is generally protected from desiccation-induced damage by the presence of membrane-compatible solutes, such as sugars and amino acids. Therefore, a link may exist between the capacity for osmotic adjustment and the degree of membrane protection from the effect of dehydration. Accumulation of antioxidant enzymes may also result in protecting membrane stability.
The inoculation also increased proline content under drought stress compared to control which may be due to up regulation of proline biosynthesis pathway to keep proline in high levels, which helps in maintaining cell water status, protects membranes, and proteins from stress (Yoshiba et al., 1997). Proline accumulation by repressed catabolic pathway under oxidative stress helps the plants to decrease oxidative damage (Nayer and Reza, 2008). The results obtained were in confirmation with several authors (Ruiz-Sanchez et al., 2011; Sandhya et al., 2010; Sandhya et al., 2011). Azospirillum and arbuscular mycorrhizal inoculation increased the shoot proline content in rice under drought condition when compared to control (Ruiz-Sanchez et al., 2011). In the present study, total phenolics were positively influenced by FD48 treatment and were significantly higher than control plants under stress condition. This result was in agreement with the report that inoculation of rice with Trichoderma spp. increased phenolics content under drought condition (Shukla et al., 2012). The phenolic compounds, besides having antifungal, anti-bacterial and antiviral activities also possess antioxidant properties and thus act as scavengers of activated free radicals (Malik and Singh, 1980).
High temperature causes membrane collapse, which leads to chlorophyll degradation in the plant. This results in the loss of chlorophyll pigment by stress, which ultimately results in the decline in the rate of photosynthesis and finally crop yield. Since Chlorophyll stability index is a function of temperature, the property of chlorophyll pigments can be correlated with drought tolerance/susceptibility of the crop plants. Prolonged drought stress reduced the chlorophyll stability index in all treatments. But FD48 treated plants showed more Chlorophyll Stability Index (CSI) when compared to Methylobacterium spp. (PPFM) and control. B. altitudinis FD48 and Methylobacterium spp. (PPFM) treated rice plants showed more catalase activity than control under drought condition. This result was in agreement with Shukla et al. (2012), Sandhya et al. (2011) and Gusain et al. (2015) who reported that under conditions of environmental stress, when ROS such as H2O2 are produced, catalase enzyme triggered by the bacteria act as scavenging enzymes and play a central role in protecting the cell from oxidative damage. Catalase and exopolysaccharides producing ability of rhizobacteria has been reported (Hussain et al., 2014).
The MDA, which is indicative of oxidative stress, increased as drought increased in plants. Our findings showed an increase in MDA in both treated and untreated plants though increase was less evident in B. altitudinis FD48 treated rice plants. Degree of accumulation of MDA content has been reported to be indicative of the rate of lipid peroxidation due to drought stress (Bailly et al., 1996). Our results are in agreement with Gusain et al. (2015) and Shukla et al. (2012) where bacterial treatment reduced the MDA content of rice under drought condition. Ethylene emission increased when rice plants were subjected to drought stress, but B. altitudinis FD48 and Methylobacterium spp. (PPFM) treated plants reported less ethylene emission when compared to control plants. This might be due to suppression of the stress-induced accelerated synthesis of ethylene by the ACC deaminase activity of the bacteria in the inoculated plants. Sharp increases in ACC levels and, consequently, ethylene synthesis in plants under drought stress conditions has been frequently reported (Asghar et al., 2002; Mayak et al., 2004). Therefore, the inhibitory effects of ethylene induced by drought stress might have been eliminated through the ACC deaminase activity of the isolates. Our results were supported by Zahir et al. (2008) who reported the reduced emission of stress ethylene by rhizobacteria treated peas under drought condition and the alleviation of water stress in chick pea by ACC deaminase producing rhizobacteria under axenic conditions (Sharma et al., 2013).
The present study shows that ACC deaminase producing B. altitudinis FD48 was capable of withstanding PEG induced drought by synthesizing osmolyte like proline. B. altitudinis FD48 treated plants withstand drought by accumulating proline, enhancing the activity of antioxidant enzymes and by reducing MDA content. Furthermore, reduction in ethylene was also reported in B. altitudinis FD48 treated plants under drought condition. It seems that B. altitudinis FD48 influenced the biochemical parameters of rice and helped them improving tolerance to water stress. B. altitudinis FD48 proved to have a promising role in improving plant performance under drought condition.
The authors have not declared any conflict of interests.
The authors gratefully acknowledge the financial support by the Ministry of Human Resource Development (MHRD-FAST CoE) (F.No.5-6/2013-TSVII), Government of India, New Delhi for carrying out this research.
REFERENCES
Abdel-Salam MS, Ibrahim SA, Abd-El- Halim SA, Badanwy SA, Abu-Aba SA (2010). Phenotypic characterization of indigenous Egyptian Rhizobial strains for abiotic stresses performance. J. Am. Sci. 619: 498-503.
|
|
Asghar HN, Zahir ZA, Arshad M, Khaliq A (2002). Relationship between in vitro production of auxins by rhizobacteria and their growth-promoting activities in Brassica juncea L. Biol. Fertil. Soils 35:231-237.
Crossref
|
|
|
Bailly C, Benamar F, Corbineau F, Cone D (1996). Changes in Malondialdehyde content and in superoxide dismutase, catalase and glutathione reductase activities in sunflower seeds as related to deterioration during accelerated aging. Physiol. Plant. 97:104-110.
Crossref
|
|
|
Bates LS, Waldren RD, Teare ID (1973). Rapid determination of free proline for water stress studies. Plant Soil 39:205-207
Crossref
|
|
|
Casanovas EM, Barassi CA, Sueldo RJ (2002). Azospirillum inoculation mitigates water stress effects in maize seedlings. Cer. Res. Commun. 30:343-350
|
|
|
Chakraborty U, Chakraborty BN, Chakraborty AP, Dey PL (2013). Water stress amelioration and plant growth promotion in wheat plants by osmotic stress tolerant bacteria. World J. Microbiol. Biotechnol. 29:789-803.
Crossref
|
|
|
Chandramohan D, Mahadevan A (1968). Indole acetic acid metabolism in soils. Curr. Sci. 37:112-113
|
|
|
Chanway CP, Holl FB (1994). Growth of outplanted lodepole pine seedlings one year after inoculation with plant growth promoting rhizobacteria. Forest Sci. 40:238-246
|
|
|
Chauhan H, Bagyaraj DJ, Selvakumar G, Sundaram SP (2015). Novel plant growth promoting rhizobacteria-Prospects and potential. Appl. Soil Ecol. 95:38-53.
Crossref
|
|
|
Chinnadurai C, Balachandar D, Sundaram SP (2009). Characterization of 1-aminocyclopropane-1-carboxylate deaminase producing methylobacteria from phyllosphere of rice and their role in ethylene regulation. World J. Microbiol. Biotechnol. 25:1403-1411.
Crossref
|
|
|
De Costa DM, Samarasinghe SST, Dias HRD, Dissanayake DMN (2008). Control of rice sheath blight by phyllosphere epiphytic microbial antagonists. Phytoparasitica 36:52-65.
Crossref
|
|
|
Delmotte N, Knief C, Chaffron S, Innerebner G, Roschitzki B, Schlapbach R, von Mering C, Vorholt JA (2009). Community proteogenomics reveals insights into the physiology of phyllosphere bacteria. Proc. Natl. Acad. Sci. USA. 106:16428-16433.
Crossref
|
|
|
Deshmukh PS, Sairam RK, Shukla DS (1991). Measurement of ion leakage as a screening technique for drought resistance in wheat genotypes. Ind. J. Plant Physiol. 35:89-91.
|
|
|
Dionisio-Sese ML, Tobita S (1998). Antioxidant responses of rice seedlings to salinity stress. Plant Sci. 135:1-9.
Crossref
|
|
|
Dworkin M, Foster J (1958). Experiments with some microorganisms which utilize ethane and hydrogen. J. Bacteriol. 75:592-601
|
|
|
Fry SC (1989). Cellulases, hemicelluloses and auxin-stimulated growth: a possible relationship. Physiol. Plant. 75:532–536.
Crossref
|
|
|
Ghosh S, Penterman JN, Little RD, Chavez R, Glick BR (2003). Three newly isolated plant growth-promoting bacilli facilitate the growth of canola seedlings. Plant Physiol. Biochem. 41:277-281.
Crossref
|
|
|
Glick B (1995). The enhancement of plant growth by free-living bacteria. Can. J. Microbiol. 41:109-117.
Crossref
|
|
|
Glick BR, Cheng Z, Czarny J, Duan J (2007). Promotion of plant growth by ACC deaminase-producing soil bacteria. Eur. J. Plant Pathol. 119:329-339.
Crossref
|
|
|
Goldberg R (1980). Cell wall polysaccharidase activities and growth processes: a possible relationship. Physiol. Plant. 50:261-264.
Crossref
|
|
|
Gopalachari NC (1963). Changes in the activities of certain oxidizing enzymes during germination and seedling development of Phaseolus mungo and Sorghum vulgare. Indian J. Exp. Biol. 1:98-100.
|
|
|
Gorden SA, Paleg LG (1957). Quantitative measurements of indole acetic acid. Physiol. Plant. 4:24-27.
|
|
|
Gusain YS, Singh US, Sharma AK (2015). Bacterial mediated amelioration of drought stress in drought tolerant and susceptible cultivars of rice (Oryza sativa L.). Afr. J. Biotechnol. 14:764-773.
Crossref
|
|
|
Honma M, Shimomura T (1978). Metabolism of 1-aminocyclopropane- 1-carboxylic acid. Agric. Biol. Chem. 42:1825-1831.
|
|
|
Hussain, MB, Zahir ZA, Asghar HN, Asghar M (2014). Can catalase and exopolysaccharides producing rhizobia ameliorate drought stress in wheat? Int. J. Agric. Biol. 16:3-13.
|
|
|
Idris EES, Bochow H, Ross H, Boriss F (2004). Use of Bacillus subtilis as biocontrol agent VI. Phytohormone action of culture filtrate prepared from plant growth promoting Bacillus amyloliquefaciens FZB24, FZB42, FZB45 and Bacillus subtilis FZB37. J. Plant Dis. Prot. 111:583-597.
|
|
|
Jiang W, Lafitte R (2007). Ascertain the effect of PEG and exogenous ABA on rice growth at germination stage and their contribution to selecting drought tolerant genotypes. Asian J. Plant Sci. 6:684-687.
Crossref
|
|
|
Kaya C, Higgs D, Ince F, Amador BM, Cakir A, Sakar E (2003). Ameliorative effects of potassium phosphate on salt stressed pepper and cucumber. J. Plant Nutr. 26:807-820.
Crossref
|
|
|
Kogut M, Russell NJ (1987). Life at the limits: considerations on how bacteria can grow at extremes of temperature and pressure, or with high concentrations of ions and solutes. Sci. Prog. 71:381-399.
|
|
|
Ma A, Zhuang X, Zhuang G (2013). Quorum quenching in culturable phyllosphere bacteria from tobacco. Int. J. Mol. Sci. 14:14607-14619.
Crossref
|
|
|
Ma W, Sebestianova S, Sebestian J, Burd GI, Guinel F, Glick B (2003). Prevalence of 1-aminocyclopropaqne-1-carboxylate in deaminase in Rhizobia spp. Antonie Van Leeuwenhoek 83:285-291.
Crossref
|
|
|
Madhaiyan M, Kim BY, Poonguzhali S, Kwon SW, Song MH, Ryu JH, Go SJ, Koo BS, Sa TM (2007). Methylobacterium oryzae sp. nov., an aerobic, pink-pigmented, facultatively methylotrophic, 1- aminocyclopropane-1-carboxylate deaminase-producing bacterium isolated from rice. Int. J. Syst. Evol. Microbiol. 57:326-331.
Crossref
|
|
|
Madhaiyan M, Poonguzhali S, Lee HS, Hari K, Sundaram SP, Sa TM (2005). Pink-pigmented facultative methylotrophic bacteria accelerate germination, growth and yield of sugarcane. Biol. Fertil. Soils 41: 350-358.
Crossref
|
|
|
Madhaiyan M, Poonguzhali S, Ryu J, Sa TM (2006). Regulation of ethylene levels in canola (Brassica campestris) by 1-aminocyclopropane-1-carboxylate deaminase containing Methylobacterium fujisawaense. Planta 224:268-278.
Crossref
|
|
|
Malik CP, Singh MB (1980). Phenolics. Plant enzymology and histoenzymology, Kalyani Publishers, New Delhi, India. 286 p.
|
|
|
Manulis S, Haviv-Chesner A, Brandl MT, Lindow SE, Barash I (1998). Differential involvement of indole-3-acetic acid biosynthetic pathways in pathogenicity and epiphytic fitness of Erwinia herbicola pv. Gypsophilae. Mol. Plant Microbe Interact.11:634-642.
Crossref
|
|
|
Marulanda A, Barea JM, Azcon R (2009). Stimulation of plant growth and drought tolerance by native microorganisms (AM fungi and bacteria) from dry environments mechanisms related to bacterial effectiveness. J. Plant Growth Regul. 28:115-124.
Crossref
|
|
|
Mayak S, Tirosh T, Glick BR (2004). Plant growth-promoting bacteria that confer resistance to water stress in tomato and pepper. Plant Sci. 166:525-530.
Crossref
|
|
|
Melody SC (1997). Plant Molecular Biology - A laboratory manual. Springer- Verlag, New York.
|
|
|
Murthy KS, Majumdar SK (1962). Modifications of technique for determination of chlorophyll stability index in relation to studies of drought resistance in rice. Curr. Sci. 31:470-471.
|
|
|
Muscolo A, Sidari M, Anastasi U, Santonoceto C, Maggio A (2014). Effect of PEG-induced drought stress on seed germination of four lentil genotypes. J. Plant Interact. 9:354-363.
Crossref
|
|
|
Nayer M, Reza H (2008). Drought-induced accumulation of soluble sugars and proline in two maize varieties. World App. Sci J. 3:448-453.
|
|
|
Ogbaga CC, Stepien P, Johnson GN (2014). Sorghum (Sorghum bicolor) varieties adopt strongly contrasting strategies in response to drought. Physiol. Plant. 152(2):389-401.
Crossref
|
|
|
Papen H, Gebler A, Zumbusch E, Rennenberg H (2002). Chemolithoautotrophic nitrifiers in the phyllosphere of a spruce ecosystem receiving high atmospheric nitrogen input. Curr. Microbiol. 44:56-60.
Crossref
|
|
|
Penrose DM, Glick BR (2001). Levels of 1-aminocyclopropane- 1-carboxylic acid (ACC) in exudates and extracts of canola seeds treated with plant growth-promoting bacteria. Can. J. Microbiol. 47:368-372.
Crossref
|
|
|
Pompelli MF, Baratta Luis R, Vitorino H, Gonclaves E,Rolim E, Santos M, Almeida-Cortez J, Endrez L (2010). Photosynthesis, photoprotection and antioxidant activity of purging nut under drought deficit and recovery. Biomass Bioenergy 34:1207-1215.
Crossref
|
|
|
Premchandra GS, Saneoka H, Ogata S (1990). Cell membrane stability an indicator of drought tolerance as affected by applied nitrogen in soybean. J. Agric. Sci. 115:1332-1343.
|
|
|
Rasanen LA, Saijets S, Jokinen K, Lindstrom K (2004). Evaluation of the roles of two compatible solutes, glycine betaine and trehalose, for the Acacia senegal Sinorhizobium symbiosis exposed to drought stress. Plant Soil 260:237-251.
Crossref
|
|
|
Rodriguez-Salazar J, Suarez R, Mellado JC, Iturriaga G (2009). Trehalose accumulation in Azospirillum brasilense improves drought tolerance and biomass in maize plants. FEMS Microbiol. Lett. 296:52-59.
Crossref
|
|
|
Ruiz-Sanchez M, Armada E, Munoz Y, De Salamone IEG, Aroca R, RuIz-Lozano JM, Azcon R (2011). Azospirillum and arbuscular mycorrhizal colonization enhance rice growth and physiological traits under well-watered and drought conditions. J. Plant Physiol. 168:1031-1037.
Crossref
|
|
|
Saitou N, Nei M (1987). The neighbor-joining method: a new method for reconstructing phylogenetic trees. Mol. Biol. Evol. 4(4):406-425.
|
|
|
Sandhya V, Ali Z, Grover M, Reddy G, Bandi V (2011). Drought tolerant plant growth promoting Bacillus spp.: effect on growth, osmolytes, and antioxidant status of maize under drought stress. J. Plant Interact. 6:1-14.
Crossref
|
|
|
Sandhya V, Ali SZ, Grover M, Reddy G, Venkateswaralu B (2010). Effect of plant growth promoting Pseudomonas sp. on compatible solutes antioxidant status and plant growth of maize under drought stress. Plant Growth Regul. 62:21-30.
Crossref
|
|
|
Saravanakumar D, Samiyappan R (2007). ACC deaminase from Pseudomonas fluorescens mediated saline resistance in groundnut (Arachis hypogea) plants. J. Appl. Microbiol. 102:1283-1292.
Crossref
|
|
|
Schonfeld M, Johnson R, Carver B, Mornhinweg D (1988). Water relations in winter wheat as drought resistant indicators. Crop Sci. 28:526-531.
Crossref
|
|
|
Sharma P, Khanna V, Kumar P (2013). Efficacy of aminocyclopropane-1-carboxylic acid (ACC)-deaminase-producing rhizobacteria in ameliorating water stress in chickpea under axenic conditions. Afr. J. Microbiol. Res. 7:5749-5757.
Crossref
|
|
|
Shukla N, Awasthi RP, Rawat L, Kumar J (2012). Biochemical and physiological responses of rice (Oryza sativa L.) as influenced by Trichoderma harzianum under drought stress. Plant Physiol. Biochem. 54:78-88.
Crossref
|
|
|
Singh R, Singh Y, Xalaxo S, Verulkar S, Yadav N, Singh S, Singh NK (2016). Plant science from QTL to variety-harnessing the benefits of QTLs for drought, flood and salt tolerance in mega rice varieties of India through a multi- institutional network. Plant Sci. 242:278-287.
Crossref
|
|
|
Sleator RD, Hill C (2002). Bacterial osmoadaptation: the role of osmolytes in bacterial stress and virulence. FEMS Microbiol. Rev. 26(1):49-71.
Crossref
|
|
|
Timmusk S (2003). Mechanism of action of the plant growth promoting bacterium Paenibacillus polymyxa. Doctoral dissertation, Acta Universitatis Upsaliensis.
|
|
|
Uma Maheshwari TC, Sivagurunathan P, Sangeetha D (2013). Performance of Bradyrhizobial isolates under drought conditions. Int. J. Curr. Microbiol. Appl. Sci. 2:228-232.
|
|
|
Upadhyay SK, Maurya SK, Singh DP (2012). Salinity tolerance in free living growth promoting rhizobacteria. Indian J. Sci. Res. 3:73-78.
|
|
|
Vanderhoff LN, Dute RR (1981). Auxin-regulated wall loosening and sustained growth in elongation. Plant Physiol. 67:146-149.
Crossref
|
|
|
Weisburg WG, Barns SM, Pelletier DA, Lane DJ (1991). 16S Ribosomal amplification for phylogenetic study. J. Bacteriol. 173:697-703.
Crossref
|
|
|
Yoshiba Y, Kiyosue T, Nakashima K, Yamaguchi-Shinozaki K, Shinozaki K (1997). Regulation of levels of proline as an osmolyte in plants under water stress. Plant Cell Physiol. 38:1095-1102.
Crossref
|
|
|
Zahir ZA, Munir A, Asghar HN, Shaharoona B, Arshad M (2008). Effectiveness of rhizobacteria containing ACC deaminase for growth promotion of peas (Pisum sativum) under drought conditions. J. Microbiol. Biotechnol.18:958-963.
|
|
|
Zhang B, Bai Z, Hoefel D, Wang X, Zhang L, Li Z (2010). Microbial diversity within the phyllosphere of different vegetable species. Current Research, Technology and Education Topics in Applied Microbiology and Microbial Biotechnology. 2:1067-1077.
|
|