This study assessed the seasonal variation of microbiological, physicochemical and heavy metal analysis of water samples from Ayao Stream around a hospital waste dumpsite. Four samples were collected downstream and analyzed for microbial count, coliforms, pH, electrical conductivity, BOD5, alkalinity, total dissolved solids, water hardness, sulphate, nitrate, chloride and phosphate using standard method. Heavy metals (Fe, Mn, Cu, Zn, Cd and Pb) in the water were determined by Atomic Absorption Spectrophotometry. Results obtained showed that the stream was greatly polluted with microbial and coliform count of 2.1 × 104 to 3.6 × 105 CFU/ml, 1.8 × 102 to 8.0 × 102 CFU/ml, and 1.1 × 103 to > 1.1 × 103 CFU/100 ml for bacteria, fungi and coliform count, respectively during both wet and dry season. The bacteria recovered from the samples included the antibiotic resistant Pseudomonas and Klebsiella. The mean values of pH (8.0 to 9.1) and BOD5 (17.2 to 26.3) were obtained during dry and wet season, respectively and were found to be beyond the acceptable limit in drinking water. This result established a relationship between the BOD5 and microbial load which indicate organic pollution through runoff input from the dumpsite into the stream. The concentration of Mn, Fe, Cu, Pb and Cd especially during rainy season was found to exceed the stipulated limit in drinking water. These metals could be bio accumulated to toxic level in aquatic organisms and end up in human through the food chain. This study shows that Ayao Stream is greatly polluted and unfit for domestic uses.
Water is a universal solvent and one required by all living organisms for survival. In most communities in Nigeria, treated pipe-borne water is lacking, so groundwater and surface water from streams and rivers are the major sources of domestic water. Water is contaminated when its acceptable quality has been altered through anthropogenic input by humans. As a result, the aesthetic value decreases having undesirable colour, taste, odour turbidity, conductivity, organic matter content, harmful chemical contents (heavy metals, persistent organic pollutants) and infectious microorganisms (Abiona et al., 2012; Ogunfowokan et al, 2005). Major sources of surface water pollution are direct discharges from industrial effluents or sewage effluents or sewage treatment plant, run-off from hospital or municipal waste dumpsite, agricultural lands or other polluted land areas.
Hospital wastes are wastes generated within the hospital environment which include sharps, chemicals, pharmaceuticals, radioactive, laboratory, general and kitchen waste. This waste is majorly composed of liquid and solid materials which have effects on the element of the environment, altering its natural composition (Akter, 2000; WHO, 2005). The treatment and disposal method appropriate for hospital waste are incineration and sanitary landfills which is monitored against leachate seeping into the soil (Diaz et al., 2005). However, in Nigeria, more than 90% of the hospital waste generated is directly disposed on land because it is economical and there is lack of proper waste disposal management technique. There is also deficiency in the designation and identification of infectious waste, segregation, packaging, and final disposal of the waste (Mayet, 1993; Vivan et al., 2011).
Hospital waste contains a great variety of chemicals, heavy metals, disinfectants and specific detergents resulting from diagnosis and research activities. Disposal of these wastes on open land area alters the natural composition of soil through the release of heavy metals, pesticides, and salts. Also, run-off from the surrounding land area causes extensive damage to the water quality characteristics, especially when microbial degradation of the anthropogenic input is unable to remove the pollutants fast enough to prevent environmental degradation. Release of heavy metals to the environment is of particular concern due to their persistence and toxicity to humans (Odewande and Abimbola, 2008; Khairiah et al., 2004). Manganese, nickel, selenium, zinc, copper, chromium, iron, lead and cadmium are typical examples of heavy metals.
Among others, lead and cadmium are known to be toxic and carcinogenic at elevated levels. Uptake of heavy metals by plants or animals results in bioaccumulation which moves through the food chain and eventually ends up in man (Laurent et al., 2011). Hence, the adverse effect of surface water pollution is the creation of a harsh environment which adversely affects the activities of aquatic microflora and aquatic lives. Improper management of hospital waste may also contribute to oxygen demand and nutrient loading of the water and ultimately result in biological and environmental loss (Sharholy et al., 2008).
The inter-relationship between the real public health effects and the range of hospital waste management processes regulated is an important cause of concern to the public at large today. There have been an increasing number of epidemiological research reports linking a range of ill-health outcomes to hospital waste management installations. These studies include childhood cancers, birth abnormalities and liver cancers associated with incinerators and landfills facilities. In this study, information has been provided on the physicochemical properties, heavy metal concentration and microbial activities of Ayao Stream located within the vicinity of the hospital with a view to establishing the pollution status of the stream as a result of anthropogenic input.
Study area
The water samples were collected along the course of Ayao Stream situated at about 110 m from a hospital waste dumpsite (07° 36’ 801’’ N and 004° 43’ 984’’ E) with an elevation of 387 m in Ilesa East Local Government Area of Osun State (Figure 1).
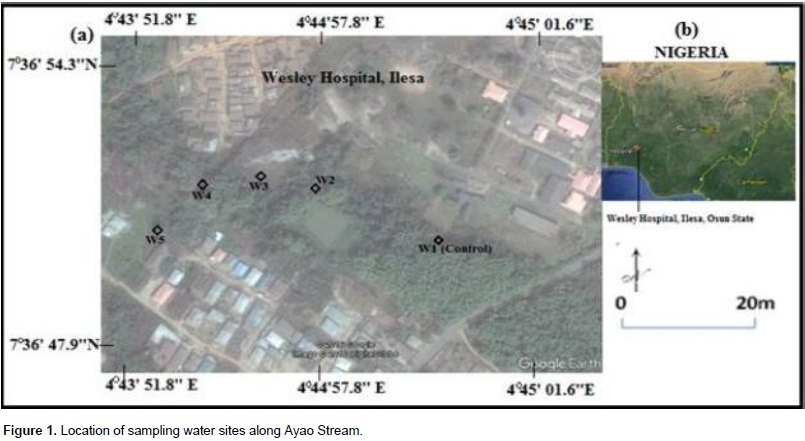
Collection of water samples
Samples for microbial and physicochemical analysis were collected from five different locations (W1, W2, W3, W4, and control sample) of the Ayao Stream during rainy season between May and October, 2013 and dry season between November, 2013 and March, 2014. Samples W1, W2, W3 and W4 were taken at about 60 m from other downstream (after the dumpsite) along the Ayao Stream. The control sample was taken at about 100 m upstream before sample location W1. Samples for physicochemical and heavy metal analysis were collected in 1-L pre-cleaned plastic bottles, rinsed with the sample and filled. The samples were taken to the laboratory and refrigerated at about 4°C prior to analysis.
The physicochemical parameters analyzed are as follows: pH, conductivity, alkalinity, chloride, hardness, nitrate, sulphate and phosphate. Standard methods were followed in determining the aforementioned variables as described by APHA (1998). The samples for microbial analysis were taken at about 10 cm below the water surface into 250-ml plastic bottles already disinfected with 75% ethanol and rinsed again with sterile distilled water. Each of the sampling containers was rinsed with water from sampling point twice before the collection of the water sample. The water samples were brought into the laboratory in plastic coolers with iced flakes and analysed within three hours of collection.
Acid digestion
Digestion of water was done according to standard method (APHA, 1998). 50 ml water sample was measured into an evaporating dish and digested by adding 5 ml concentrated HNO3 and 1 ml HClO4. The solution was heated on a steam bath until the volume was reduced to about 15 ml. The solution was allowed to cool, it was filtered and made up to 25-ml mark in a volumetric flask. Analysis for heavy metal concentration was carried out using the Perkin Elmer 400 atomic absorption spectrophotometer by flame atomization, using air-acetylene flame and single element hollow cathode lamp at the Central Science Laboratory of Obafemi Awolowo University, Ile-Ife, Nigeria.
Total microbial counts
Enumerations of total microbial (bacterial and fungal) counts were carried out using the serial dilution and pour plate method. Serial10-fold dilutions in sterile distilled water were carried out and 1 ml of each dilution was aseptically dispensed in sterile Petri-dishes in duplicates. A volume of 20 ml of molten nutrient agar (Lab M) cooled to 45°C for bacterial population or Malt Extract Agar (Lab M) for fungi was later added to each of the plates. The mixture was allowed to solidify and incubated inverted for 24 to 48 h at 35°C for bacteria.
For fungi, the cultured plates were incubated un-invertedly and aerobically at 30°C for 5 to 7 days (until the plates showed no further increase in the number of fungal colonies). The plates were observed for growth and selected for count after the expiration of the incubational periods. The cultured plates in which the number of colonies was between 30 and 300 were selected. The count obtained was multiplied by the dilution factor and expressed as colony forming unit (CFU) per ml of the original sample. This is the viable count.
Presumptive coliform count
The water samples were processed using the multiple fermentation tube method to determine the presumptive coliform count or most probable number (MPN) of coliforms based on standard methods (Senior, 2006). Suspensions from positive tubes were sub-cultured onto MacConkey agar and incubated at 37°C for 24 to 48 h, and the resulting colonies identified using standard procedures (Forbes et al., 2007).
Confirmatory test
The tubes showing gas and/or acid production (MPN tubes) were sub-cultured on Eosin Methylene blue (EMB) agar incubated at 35°C for 24 h and examined for typical colonies of E. coli (greenish metallic sheen). If typical colonies were seen, then the completed test was carried out.
Completed test (Eijkman test)
Eijkman’s test, which is for the detection and enumeration of faecal coliform, involves the use of elevated temperature for the differentiation of faecal and non-faecal coliforms. Measured volume of water samples were inoculated into lactose broth in similar combinations as in the presumptive test for total coliforms and incubated at 44°C for 48 h. The number of positive tubes was recorded at the end of the incubation period and the MPN of faecal coliform was read from the MPN index table.
Isolation of pure cultures of microorganism
The distinct colonies from the mixed culture on each of the nutrient agar and the malt extract agar plates were picked and streaked onto fresh plates. Pure colonies of each bacterium and fungus were then picked for identification using standard biochemical tests which included Gram’s stain, catalase, MRVP, citrate utilization, indole, oxidase, nitrate reduction, hydrogen sulphide production and sugar fermentation tests for bacteria.
Physicochemical parameters in steam water
The mean value ± standard deviation results of some physicochemical parameters analyzed in the stream water for the period of sampling are shown in Table 1. The level of pH varied between 7.9 to 8.1 and 8.8 to 9.7 during dry and wet seasons, respectively. The mean pH values during wet season exceeded the Standard Organisation of Nigeria (SON, 2008) limit between 6.5 and 8.5 for drinking water. The high pH values suggest the presence of dissolved gases such as NH3 and some alkali chemicals in the water resulting from runoff from the hospital dumpsite. Nevertheless, the high pH values in the study area suggest that the water may not influence the solubility of toxic metals in the water bodies.
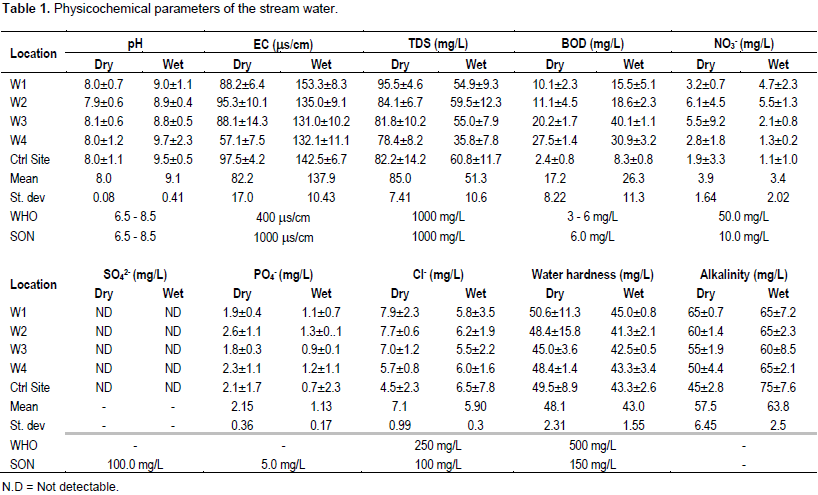
The electrical conductivity (EC) varied between 57.1 to 97.5 ms/cm during dry season while higher EC values were obtained in the wet season (131 to 153.3 ms/cm). Higher values during wet season may be due to increased concentration of ionized substances in the water resulting from run-off of nutrients or chemicals from surrounding land area of the hospital waste dumpsite into the stream water. The mean EC values in both seasons were within the SON (2008) limit of 1000 ms/cm in drinking water.
Total dissolved solids (TDS) varied between 78.4 to 95.5 mg/L and 35.8 to 60.8 mg/L during dry and wet seasons, respectively. Higher TDS value during dry season could be as a result of reduction in the volume of water, which results in high concentration of the mineral content. The results of TDS obtained in this water shows that the values are within the acceptable limit of 1000 mg/L (WHO, 2006) for domestic use regardless of other parameters. TDS level higher than 1000 mg/L in drinking water increases turbidity of water, affecting the aesthetic value of water and making it unpalatable to taste.
BOD5 is a measure of oxygen demand by microorganism while breaking down organic matter. The BOD5 values range from 10.1 to 27.5 mg/L during dry season and 15.5 to 40.1 mg/L during wet season compared to 3.0 mg/L and 3.0 to 6.0 mg/L set by WHO (2006) and SON (2008), respectively. Higher BOD5 values obtained during the wet season may result from leaching and runoff from land surrounding the dumpsite which increase the level of organic matter and activities of microorganism in the stream. As a result, the dissolved oxygen will decrease over time, promoting toxic algae blooms and leading to a destabilized aquatic ecosystem. Hence, BOD5 result shows that the water is polluted and unfit for use for domestic purposes.
Chloride is a major ion in surface water. The mean concentrations of Cl- in the stream water were minimal, well below the permissible limit of 100 mg/L (WHO, 2006) in water. Chloride occurred in the dry season in the range of 5.7 to 7.9 mg/L and 5.5 to 6.1 mg/L during wet season.The mean value of water hardness during dry season varied moderately between 45.0 to 50.6 mg/L and 41.3 to 45.0 mg/L during wet seasons. However, the values of water hardness fall into the category of soft water according to Radojevic and Bashkin (1999), which suggest low solubility of toxic multivalent metals in water.
The alkalinity values showed no observable variation in both seasons as it occurred in the range of 50 to 65 mg/L during dry season and 60 to 65 mg/L during wet season, although it is still within the limit of 100 mg/L allowed for
potable water. Although, alkalinity has little public health significance (Radojevic and Bashkin, 1999), highly alkaline water is unpalatable and not good for domestic water supply.
The concentration of nitrate and phosphate varied between 2.8 to 6.1 mg/L and 1.8 to 2.6 mg/L, respectively during dry season and 1.3 to 5.5 mg/L and 0.9 to 1.3 mg/L during wet season. The levels of nitrate and phosphate in the samples did not exceed the SON (2008) safe limit of 10 and 5 mg/L, respectively. Low phosphate values were evidenced by the low chlorophyll in the stream water. Sulphate was below the detection limit of the instrument in all the samples.
Heavy metal concentration in stream water
The result obtained from the metal analysis of stream water samples in the study area using Atomic Absorption Spectroscopy are presented in Figure 2. Statistical T-test analysis for metal concentration during wet and dry seasons indicates that significant difference (P > 0.05) exist in Mn, Zn and Cd concentration in the stream water. The general trend observed for the metals are Fe>Mn>Zn>Cu>Cd>Pb for dry season; and Fe>Mn>Cu>Zn>Pb>Cd for wet season. The highest concentration value was recorded for Fe among other metals in both seasons. Iron (Fe) in the water samples ranged from 1 to 98 mg/L and 104 to 135 mg/L for dry and wet seasons, respectively, with the highest value occurring at sampling point W1 in both seasons.
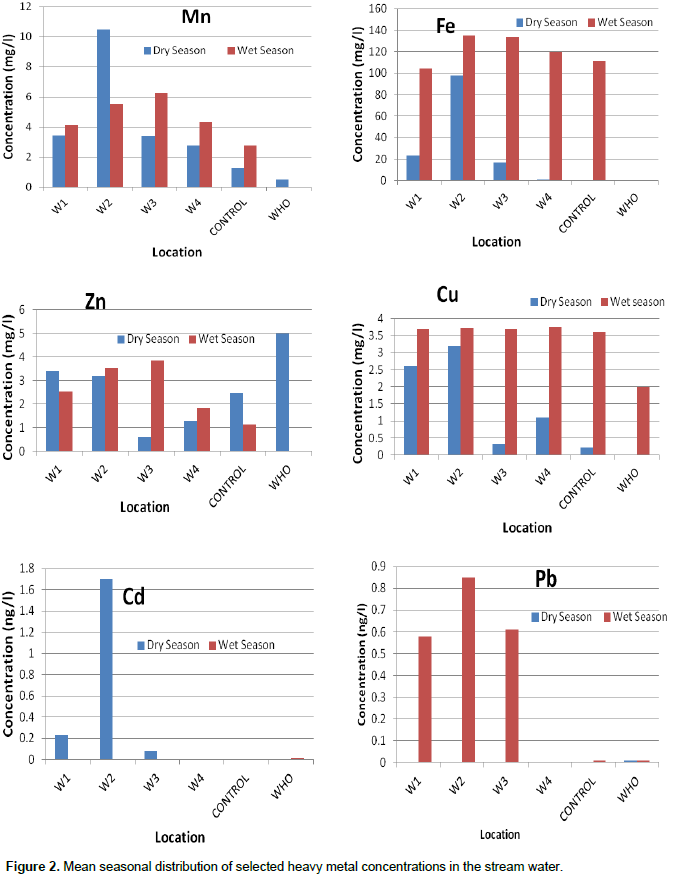
The mean concentration values for Fe in all samples for both seasons were higher than the WHO (2006) standard value of 0.3 mg/L for Fe in drinking water. High levels of iron found in the control sample during wet season suggest that there may be a direct discharge of pollutant substances into the water up stream. High Fe levels may result from runoff of land soil in complex with organic matter around the hospital waste dumpsite. The higher concentration of Fe obtained during wet season may be due to increased decomposition of organic matter in the hospital waste that is washed off into the stream water during rainfall. Also, iron could be leached from corroding metal which is abundant in the hospital dumpsite and eventually washed off into the stream.
According to Canfield et al. (1984), Fe at high levels contributes to water coloration and reduces light penetration which is evident in the study area and may affect the biogeochemical cycling of organic matter, phosphorus and nitrogen. Photo-oxidation of organic matter is enhanced by Fe (Minor et al., 2006). Also, Fe can have direct and indirect toxic effects on aquatic biota. Precipitation of Fe on fish gills can impair ion regulation and oxygen uptake (Peuranen et al., 1994). In humans, iron toxicity affects the red blood cells chemistry and delay normal and mental development in children (Ekiz, 2005).
Concentrations of Manganese (Mn) obtained in the water samples ranged between 2.79 to 10.48 mg/L and 4.14 to 6.28 mg/L for dry and wet seasons, respectively. These values exceed the WHO (2006) standard value of 0.5 mg/L for Mn in drinking water. Anthropogenic sources of manganese include mining and smelting, engineering, traffic and agriculture. It is also used in the manufacture of steel, glass, dry batteries and chemicals. Manganese can be released to the stream water by leaching and runoff of these materials from the dumpsite. Excessive amount of Mn causes seizure activity, poor bone formation, impair fertility, birth defect in humans and irreversible brain diseases with prominent psychological and neurological disturbances (Agency for Toxic Substances and Disease Registry (ATSDR), 2000; United States Environmental Protection Agency (USEPA), 1984).
Concentrations of Copper in the water range between 0.32 to 3.19 mg/L in the dry season while it was found in the range of 3.68 to 3.74 mg/L in the wet season. Higher concentration values of copper during the wet season exceeded the WHO (2006) stipulated safe limit of 2.0 mg/L. The levels of zinc in water were within the WHO limit of 5 mg/L in drinking water. Lead, a toxic heavy metal was below detection limit in the samples collected during the dry season. However, during wet season it appeared at high levels above the WHO (2006) stipulated limit of 0.05 mg/L in the water, as it ranged from 0.58 to 0.85 mg/L. High levels of exposure to Pb may result in toxic biochemical effects in humans which in turn cause problems in the synthesis of haemoglobin, affects the kidneys, gastrointestinal tract, joints and reproductive system, and could cause acute or chronic damage to the nervous system (Goyer, 1986).
Cadmium in water occurred at high levels ranging from 0.08 to 0.23 mg/L during dry season, but was below the detection limit in the samples during wet season. The values for dry season were above the safe limit of 0.003 mg/L Cd stipulated by WHO (2006). The absence of Cd during the wet season might be due to the effect of rainfall which increased the volume of water and water flow rate, eventually lowering the concentration of Cd. Excessive amount of Cd is known to cause damage to body cells by increasing their permeability and enhancing the accumulation of other heavy metals. It is also known to cause kidney damage and bone pains in man (Jarup, 2003). This result shows a higher concentration of the metals in the stream during wet season which indicate there was more discharge through surface runoff during rain which is enhanced by the sloppy topography of the land area.
With exception of zinc, higher levels of Fe, Mn, Cu, Cd and Pb in the stream water suggest anthropogenic input through run-off from the hospital waste dumpsite (Figures 3 and 4). Also, anoxic condition in the water with respect to the BOD5 level may enhance the solubility of some of these metals which increase the leaching of metallic objects in the hospital dumpsite. Other anthropogenic sources that may be responsible for the presence of high values of these metals in the study samples include atmospheric deposition and the use of agrochemicals in nearby farmlands as shown in Figure 2. This result indicates that the aquatic biota may bioaccumulate these metals to toxic levels and enters the food chain to human. Hence, the assessment of metal concentration in Ayao Stream shows that the water is polluted and therefore unfit for domestic and recreational purposes.
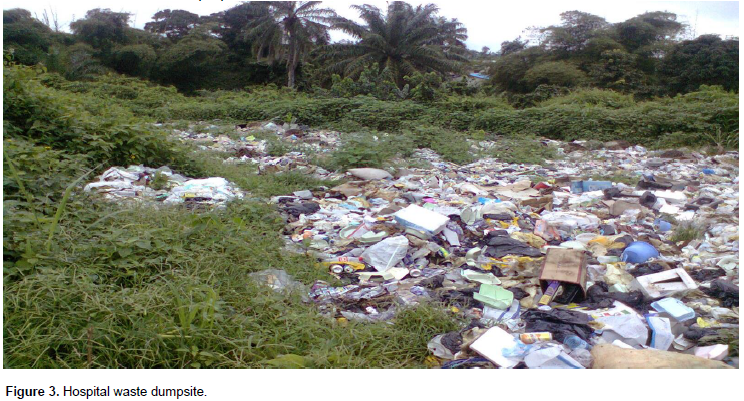
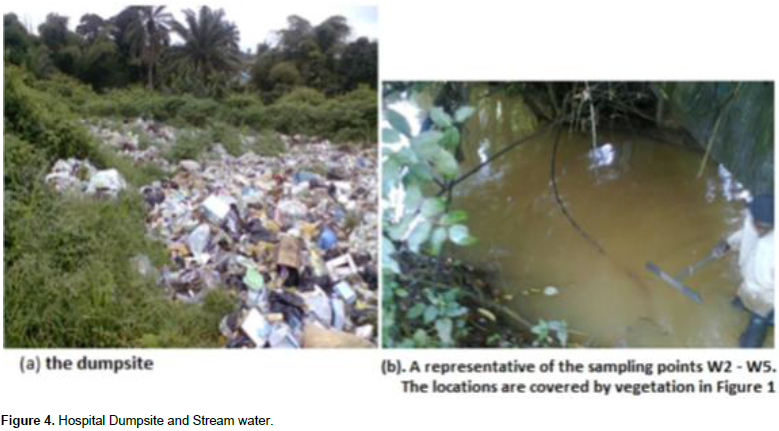
Dominant genera of microorganisms isolated from sampling points of Ayao Stream
A total of 23 strains of bacteria representing 9 genera and 7 species of fungi were isolated from the water samples of Ayao Stream as shown in Tables 2 and 3. The dominant bacteria genera in the upstream samples were Pseudomonas, Enterobacter, Aerococcus and Acinectobacter while Klebsiella, Escherichia, Shigella and Proteus were the major genera isolated in downstream water samples. Six fungal species which included Rhizopus japonicus, Pullularia pollulans, Trichoderma sp., Absidia sp., Aspergillus glaucus and Aspergillus fumigatus were isolated from both the upstream and downstream water samples while additional Mucor mucedo was isolated from the control site sample.
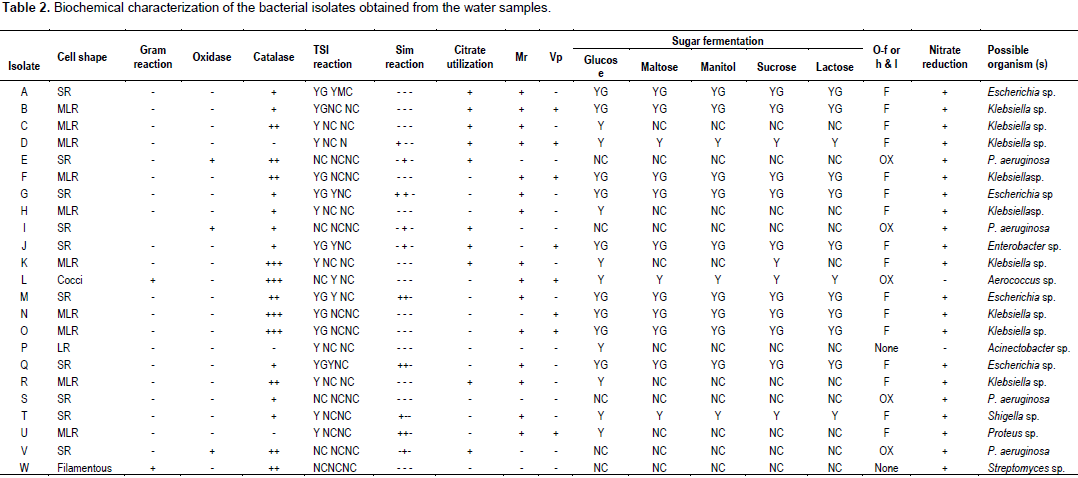
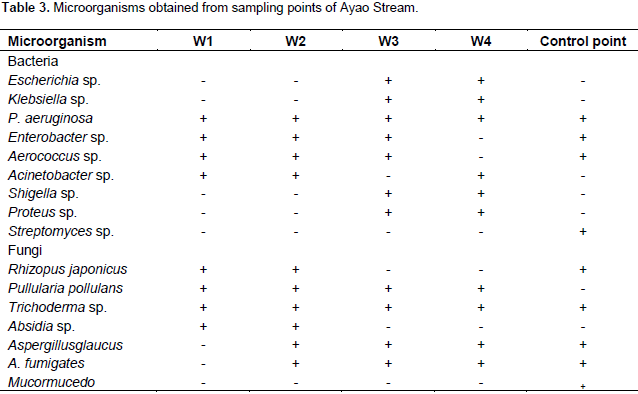
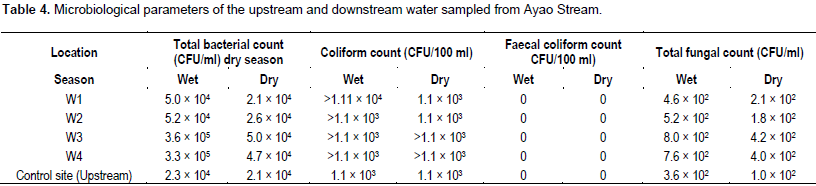
Microbiological parameters of stream water
The microbiological parameters of the Ayao Stream (upstream and downstream) sampled and analysed with bacterial, coliform bacterial, faecal coliform and fungal counts are presented in Table 4. The downstream samples (W3 and W4) contained higher bacterial (4.7 × 104 - 3.6 × 105 CFU/ml) and fungal (4.0 × 102 - 8.0 × 102 CFU/ml) counts in both dry and wet seasons. A higher bacterial and fungal load was observed during the wet season in all the samples, which is most likely due to the surface runoff of decaying organic matter which suitably introduced more organisms and increased microbial activity in the stream. Similar findings were reported by Adewoye (2010) and Fakorede et al. (2013). However, the control samples (upstream) contained lesser loads of bacteria count (2.3 × 104 and 2.1 × 104 CFU/ml) and fungal count (3.6 × 102 and 1.0 × 102 CFU/ml) when compared with the downstream samples for wet and dry seasons respectively.
The low microbial counts in the control samples suggest that the runoff from the dumpsite is a point source of pollution. Also, the microbial load was observed to increase with increasing distance from the dumpsite (downstream). This is in agreement with the BOD5 value which could result from lower water volume and flow rate downstream. Hence, the microbial load is more concentrated. The coliform bacterial counts ranged from 1.1 × 103 to >1.1 × 103 CFU/100 ml. The result of the coliform count in the water samples is suggestive of the fact that the dumpsite contributed to the presence of coliform bacteria in the water bodies. Therefore, none of the water bodies is safe for drinking since their bacterial and coliform bacterial densities greatly exceeded the World Health Organization (WHO, 2004) standard of 10 CFU/ml in drinking water.
Faecal coliforms were however, not isolated from all the samples collected indicating that faecal wastes were not being introduced into water body from the dumpsite. The bacterial strains isolated from the samples belong to the genera Escherichia, Klebsiella, Pseudomonas, Acinectobacter, Streptomyces, Proteus, Shigella, Enterobacter and Aerococcus. Some of these bacteria are implicated in infections of wounds, burns, ulcer and urinary tract. The presence of these microbes is of public health importance because of their potentials as pathogens and the fact that they can be multi resistant to antibiotics which may make the choice of antibiotics for treatment of infections arising from them a herculean task. The fungi isolated from these samples especially Trichoderma sp. are implicated in human skin diseases.
From the results obtained in this study, the values of physicochemical parameters in stream water were within the standard limit stipulated by WHO (2006) and SON (2008). However, significant pollution was indicated by the high values of pH and BOD especially during wet season above the standard limit in water. High BOD value in stream water indicates organic pollution due to run-off input from the hospital dumpsite and agricultural land which increase microbial decomposition of organic matter in water. On the other hand, the total metal concentration of Fe, Mn, Cu, Pb and Cd in stream water were higher than the standard set by WHO (2006). This suggests that there could be danger of bioaccumulation of toxic metals for aquatic life which eventually leads to toxicity in the biota over time.
The microbiological results revealed that the Ayao Stream was greatly polluted by the run-off from the dumpsite into the stream as the samples contained very high microbial loads throughout the sampling period. The coliform count indicate that the water bodies is unsafe for drinking since their bacterial and coliform bacterial densities greatly exceeded the acceptable limit by World Health Organization (WHO) in drinking water. Certain bacteria and fungi strain isolated from the stream water have the potential to become pathogens and can be multi resistant to antibiotics. This study is particularly of public health significance because this stream flowed outside the hospital premises and it is being used by a good number of people including farmers for irrigation, washing, bathing and other domestic purposes. This suggests that hospital waste in the environment is a major health and environmental threat, which call for a proper regulatory system of its disposal.