ABSTRACT
This study was designed to characterize morphological, physiological and molecular responses of sugarcane genotypes to a simulated water deficit stress. Two genotypes (TSP05-4: Drought-tolerant; TCP02-4589: Drought-sensitive) were subjected to a 20-day water deficit treatment and an 8-day recovery period. Leaf photosynthesis (Pn), transpiration rate (E), stomatal conductance (Gs), leaf greenness index (SPAD) and variable-to-maximum chlorophyll a fluorescence ratio (Fv/Fm), were evaluated before, during and after water deficit. Root-to-shoot ratio (R/S), stalk height (SH), diameter (SD) and stalk weight (SW) were evaluated at the end of the experiment. Real-time RT-PCR confirmed seven differentially-expressed transcript-derived fragments (TDFs) identified by cDNA-AFLP. Pn rates were similar between the genotypes under well-watered conditions. However, under water deficit, TSP05-4 had higher Pn rates. SPAD, Fv/Fm and R/S were also generally higher in TSP05-4, regardless of soil moisture status. Water deficit-induced reductions in SH and SW were greater in TCP02-4589 than in TSP05-4. Three TDFs showing sequence similarities to genes encoding a putative expressed pentatricopeptide, a protein kinase CK2 regulatory subunit CK2β3, and a glucose-6-phosphate/phosphate translocator 2 were identified in TCP02-4589. One TDF similar to a drought-inducible protein was identified in TSP05-4. Recovery of physiological processes and gene expression patterns to the water stress levels was fast.
Key words: Differential gene expression, water deficit stress, re-watering, Saccharum spp.
Sugarcane (Saccharum sp.) is an economically important crop that is cultivated in more than 90 countries for sugar, ethanol and biomass production. Since sugarcane production is concentrated in many regions where water supply is either inadequate or irrigation infrastructures are underdeveloped, water deficit stress is a major limitation to optimal productivity of this crop (Inman-Bamber and Smith, 2005). Developing varieties that use water more efficiently is, therefore, an important goal for sugarcane improvement programs.
The period between 60 and 150 days of crop age, known as the formative phase, has been shown to be very sensitive to water deficit stress in sugarcane (Naidu, 1976). Water deficit during this phase has been shown to adversely affect gene and protein expression, morphological, physiological and biochemical traits, and consequently, cane and sugar yields (Rocha et al., 2007; Silva et al., 2008; Cha-um and Kirdmanee, 2009; Rodrigues et al., 2009).
Plasticity in adjusting to and recovering from drought is often overlooked in drought response studies, even though these mechanisms can enhance crop survival during water shortages (Ashton, 1956; Inman-Bamber, 1995). A better understanding of these responses and mechanisms is necessary in developing guidelines and procedures to efficiently screen germplasm for stress tolerance.
Studies have focused on understanding the morphological, physiological and molecular processes responsible for high performance under drought conditions in sugarcane (Inman-Bamber and Smith, 2005; Rocha et al., 2007; Silva et al., 2008; Cha-Um and Kirdmanee, 2009; Rodrigues et al., 2009; Zingaretti et al., 2014). However, few studies have integrated these processes to achieve progress in genetic improvement of sugarcane drought tolerance. Such studies may contribute to the development of tolerant genotypes, either for transgenic plant development or for marker-assisted breeding.
The objective of this study was to characterize morphological, physiological and molecular responses to water deficit stress and to re-watering in two sugarcane genotypes.
Plant materials and growth conditions
This study was conducted from March to July, 2009, in a greenhouse at the Agrilife Research and Extension Center, Weslaco, Texas, USA. Two sugarcane genotypes classified as either drought tolerant (TSP05-4) or sensitive (TCP02-4589) were used. Two-week plantlets, transplanted into 15-L pots containing MM200 substrate, were watered daily, and fertilized two times per week with 10N-4.4P-8.3K (Peter’s Corp., St. Louis, Mo.) until 69 days after planting (DAP). The average daily photosynthetic photon flux at canopy level was 15±3.8 mol·m-2. Average day/night temperatures were 28.8±4.4 / 21.7±3.2°C and average day/night relative humidity values were 48±11/68±11%. At 70 DAP, irrigation treatments were initiated. Plants of each genotype were randomly divided into one group subjected to a water deficit stress regime by maintaining volumetric soil moisture (VSM) at ~15%, and another group being well-watered (VSM ~ 35%). Volumetric soil moisture contents were monitored continuously using soil moisture sensors (EC5, Decagon Devices, Inc) connected to data loggers (Em5b, Decagon Devices, Inc). Irrigation treatments were maintained until 90 DAP.
Physiological and morphological analyses
Leaf photosynthesis (Pn), stomatal conductance (Gs), transpiration rate (E), variable-to-maximum chlorophyll a fluorescence ratio (Fv?Fm), leaf greenness index (SPAD) and leaf relative water content (RWC) were evaluated at two days before water deficit stress initiation (T0), at two, twelve and twenty days after initiation of water deficit stress treatment (T1, T2 and T3, respectively), and at eight days after re-watering (T4).
The traits Pn, Gs and E were measured using a portable gas exchange system CIRAS-2 (PPSystems) under ambient temperature, light saturation (1,500 µmol·m-2·s-1) and CO2 partial pressure of 35 Pa. A pulse amplitude modulation fluorometer (Model OS5-FL, Opti-Sciences, Tyngsboro, MA, USA) was used to measure Fv?Fm of leaves dark-adapted for 30 min. Fv is the variable fluorescence (Fm-F0), Fm is the maximal fluorescence yield following a saturating pulse of light and F0 is the minimal fluorescence yield in the absence of actinic light.
Leaf greenness index (SPAD) measurements were made with a Minolta SPAD-502 chlorophyll meter (Minolta Corp., Ramsey, NJ, USA). The measurements of SPAD, Pn, Gs and E were completed between 10:00 and 12:00, using the 3rd leaf from the top-most visible dewlap of stalk. Following gas exchange and SPAD measurements, leaf disks (1 cm2) were sampled from each plant and used for RWC determination. Leaf tissue samples for RWC determination were collected around 15:00. RWC was calculated following the method of Matin et al. (1989).
Following physiological measurements at T4, plants were harvested and root-to-shoot ratio (R/S), stalk height (SH), stalk diameter (SD) and stalk weight (SW) were measured. Plants were harvested at soil level and divided into leaves, stems and roots. SH was measured from the base of the top-most visible dewlap to the soil level while SD was measured with a pair of calipers at 10 cm from the soil level. Individual biomass components were oven-dried (70°C, 72 h) and the dry weight data were used to calculate R/S.
cDNA-AFLP analysis
Leaf tissue samples were collected at the T1, T2 and T4 evaluation times from three plants of each treatment. Tissue samples were immediately frozen in liquid nitrogen and stored at -80ºC. Total RNA extraction and Poli (A)+ RNA isolation were achieved using the QIAGEN Rneasy Plant Mini Kit (Qiagen, Valencia, CA) and MicroPoly(A) PuristTM Kit (Ambion), respectively, following the manufacturer’s instructions. Single and double stranded-cDNAs were synthetised using the SuperScriptTM Double-Stranded cDNA Synthesis (Invitrogen).
cDNA-AFLP analysis was performed using the AFLP® Expression Analysis Kit of LI-COR (LI-COR, Lincoln, NE). Restriction enzymes TaqI and MseI were used to digest the cDNA and to generate pre-amplification PCR products. Selective PCRs were performed with 22 primer combinations obtained by the eight MseI+2 primers and the eight TaqI+2 primers, where +2 represents two selective nucleotides: +GA, +GT, +TC, +TG, +CT, +CA, +AG and +AC on both adaptor primers. The TaqI+2 selective primers were labeled with 700 and 800-nm infrared dye (LI-COR, IRDye 700 and IRDye 800). Selective PCR products were resolved on 6.5% denaturing polyacrylamide gels in a LI-COR DNA analyser (model 4300 LI-COR®). Eletrophoretic run parameters were: 1500 V, 40 W, 40 mA, 45°C, 25-min pre-run and 2-h main run. Data images were collected using LI-COR’s Saga AFLP Analysis Software.
Isolation of differentially-expressed TDFs was perfomed according to the AFLP® Expression Analysis Kit, mentioned previously. The TDFs excised from gels were purified using the ZymocleanTM Gel DNA recovery Kit (Zymo Research). The purified TDFs were subsequently cloned into the pGEM®-T Easy vector (Promega Corp., Madison, WI) and then used to transform Escherichia coli DH5α competent cells. Recombinant plasmids were isolated using ZyppyTM plasmid Miniprep kit (Zymo Research). Purified plasmids containing the insert were sequenced using an automated DNA sequencer (Applied Biosystem, Inc.) at Iowa State University’s, DNA Facility (Ames, Iowa, USA).
Nucleotide and translated sequences were analysed for homology with nucleotide and protein sequences available in the GenBank (http.//www.ncbi.nlm.nih.gov/BLAST) database using the BLASTx and BLASTn search tools, respectively.
Real-time RT-PCR analysis
Real-time reverse transcription PCR (RT-PCR) was used to confirm 7 TDFs isolated. The primers (Table 1) were designed using the Primer 3 program (http://frodo.wi.mit.edu/cgi-bin/primer3/primer3_www.cgi) and compared to the NCBI database using the Blast tool to verify the specificity of sequences. RT-PCR reactions were performed using iQTM SYBR® Green Supermix (Bio-Rad laboratories, Hercules, CA, USA). The glyceraldehide-3-phosphate de-hydrogenase (GAPDH) gene was used as an endogenous reference gene (Iskandar et al., 2004). The reactions were performed for each sample in triplicates. The following amplification program was followed using a BioRad iCycler iQ5 thermocycler (Bio-Rad laboratories, Hercules, CA, USA): 50ºC for 2 min, 95ºC for 10 min followed by 40 cycles of 95ºC for 15 s and 60ºC for 1 min. The level of relative gene expression of each fragment normalized to the endogenous reference gene were calculated using the 2-Δ(ΔCt) method (Livak and Schmittgen, 2001).
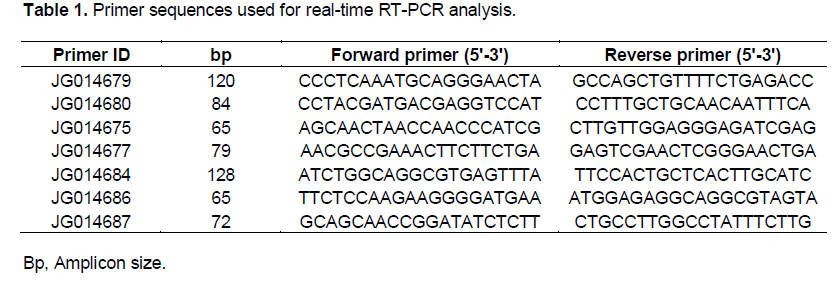
Experimental design and statistical analysis
The physiological experiment setup was a split-plot in time arrangement, with evaluation times as the main plot, and water supply regimes and genotypes as sub-plots replicated four times. The growth experiment setup was a split-plot, with water supply as the main plot, and genotypes as sub-plots replicated four times. Analysis of variance was performed at probability of 5%.
Physiological and morphological analyses
Gas exchange parameters (Pn, E and Gs) were significantly reduced by water deficit stress in both genotypes (Figure 1). Prior to initiation of water deficit stress (T0), there were no significant differences in these parameters between the genotypes. By the evaluation times T1, T2 and T3, however, stress-induced differences were observed.
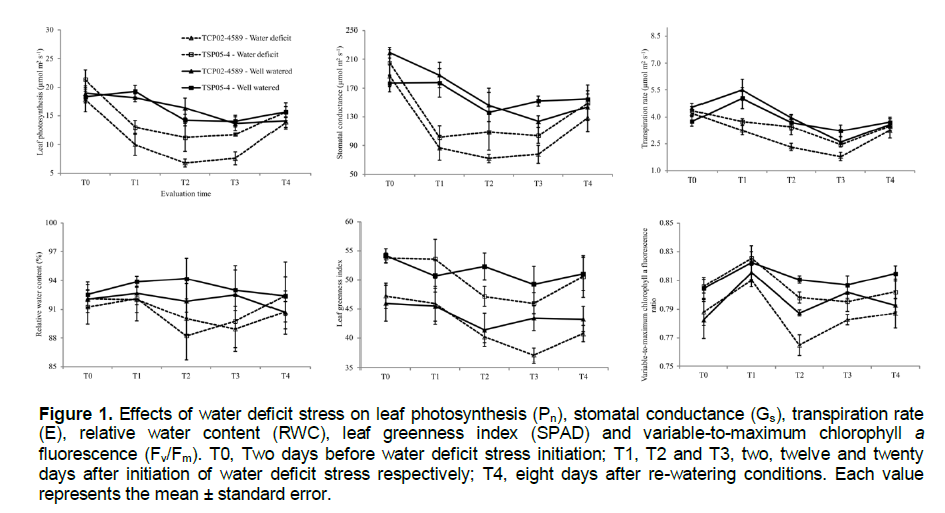
Under well-watered conditions, the average gas exchange parameters of both genotypes were similar (Figure 1). Under water deficit, however, the average Pn, E and Gs of TSP05-4 were, respectively, about 48, 31 and 33% higher than those of TCP02-4589. The lower reduction in Gs of TSP05-4 may explain why its Pn values were also higher than those of TCP02-4589 under water deficit stress. Regulation of water loss through transpiration by stomata is a well-known mechanism for maintaining higher and favorable plant water status, which in turn allows the plant to sustain physiological processes under mild water deficit conditions.
Despite the higher reductions in gas exchange parameters of TCP02-4589, RWC did not differ between the tolerant and sensitive genotypes (Figure 1). RWC values of TCP02-4589 were still high (above 80%) compared to results found in other sugarcane studies (Jangpromma et al., 2007; Cia et al., 2012; Boaretto et al., 2014), which RWC values of sensitive genotypes were lower than 70% under severe water stress. A possible explanation for the lack of differences among drought-tolerant and sensitive genotypes is that water stress was not severe enough to influence the RWC.
A complete recovery of Pn, Gs, E, and RWC was observed for both genotypes when plants were re-watered for 8 days (T4), following the water deficit stress treatments (Figure 1). These results suggest a high degree of physiological plasticity of the sugarcane genotypes in response to changing water conditions, as has been reported for other sugarcane genotypes (Ashton, 1956; Inman-Bamber, 1995).
SPAD values differed between genotypes with TSP05-4 having higher values (~51) than TCP02-4589 (~43; Figure 1). Also, decline in Fv/Fm was slightly more severe in genotype TCP02-4589. The higher values of SPAD and Fv/Fm found in genotype TSP05-4 suggest a greater capacity for radiation capture and radiation use efficiency in this genotype.
R/S ratio was higher in genotype TSP05-4 (0.62) compared to TCP02-4589 (0.36), regardless of water supply conditions (Figure 2). Under well-watered conditions, the average SH and SW of TCP02-4589 were, respectively, about 37 and 34% greater than that of TSP05-4. Stress-induced reductions in SH and SW of TCP02-4589, however, were much greater (about 22 and 31%, respectively) than those of TSP05-4 (about 4 and 13%, respectively). This maintenance of TSP05-4 probably resulted from higher values of Pn in this genotype. Despite these results, stalks of TCP02-4589 were heavier (about 13%, respectively) than those of TSP05-4, suggesting potential tradeoffs in productivity for survival. The average SD was not different between genotypes.
cDNA-AFLP analysis
About 1550 transcript-derived fragments (TDFs) were detected and an average of 70 TDFs per primer combination was produced. At least 30 TDFs were classified as differentially-expressed, with 24 TDFs being down-regulated and six up-regulated in response to water deficit. Twenty-three and six TDFs were detected exclusively in TCP02-4589 and TSP05-4, respectively, while one TDF was simultaneously present in both genotypes. Nineteen TDFs detected exclusively in TCP02-4589 were down-regulated, whereas in TSP05-4, 4 TDFs were down-regulated and 2 were up-regulated. These results show that at the molecular level, water stress responses were detected earlier in genotype TCP02-4589.
A total of 11 and eight differentially-expressed TDFs were exclusively detected at T1 and at T2, respectively, while 11 TDFs were detected at both evaluation times. One TDF was up-regulated at T1 and five at T2, while 10 TDFs were down-regulated at T1 and three at T2. All TDFs detected at both evaluation times were down-regulated.
In both genotypes, TDFs regulated by water deficit stress resumed their processes and normal expression patterns after the re-watering period. The ability to resume normal molecular and physiological functions indicated that the magnitude and duration of water deficit stress did not impair the ability for recovery.
Thirteen differentially-expressed TDFs were sequenced and its characteristics are described in Table 2. Five TDFs (JG014679, JG014675, JG014682, JG014686 and JG014687) showed significant similarity to genes with known or putative function, three (JG014680, JG014676, JG014684) were similar to hypothetical proteins and five (JG014681, JG014683, JG014677, JG014685 and JG014678) did not show significant similarity to any nucleotide sequence or protein in the non-redundant database.
Seven TDFs were selected for RT-PCR analysis and are indicated with an asterisk in Table 2. RT-PCR confirmed the regulation of five TDFs (JG014679, JG014680, JG014675, JG014684 and JG014686) while two (JG014677 and JG014687) did not show changes in the relative levels of gene expression between control and water deficit treatments (Figure 3).
Three TDFs showing significant sequence similarities to genes encoding a putative expressed pentatricopeptide (JG014679), a protein kinase CK2 regulatory subunit CK2β3 (JG014682) and a glucose-6-phosphate/phosphate translocator 2 (JG014686) were differentially-regulated in genotype TCP02-4589. JG014675 which is similar to a drought-inducible protein was differentially-regulated in TSP05-4 at T2. Also, one TDF (JG014687) similar to a tocopherol polyprenyltransferase gene was down-regulated in both genotypes at T2.
Pentatricopeptide repeat (PPR) is a protein family involved in plant development, organelle biogenesis, restoration of cytoplasmic male sterility, RNA processing and editing in mitochondria and chloroplasts, and responses to environmental stresses (Meierhoff et al., 2003; Lurin et al., 2004; Rodrigues et al., 2009). Under the water deficit conditions imposed in the present study, a PPR like-protein (JG014679) was suppressed at T1 and T2 in TCP02-4589. The suppression of JG014679 may have contributed to reduced Pn values observed in TCP02-4589 under water deficit. Two genes which show similarity to PPR proteins have also been previously observed in sugarcane genotypes exposed to waterdeficit stress (Rodrigues et al., 2009).
JG014686, which was also suppressed at T2 in TCP02-4589, is similar to a glucose-6-phosphate/phosphate translocator 2. Glucose-6-phosphate/phosphate translocator represents a distinct member of the phosphate translocator protein family and its proposed physiological functions include import of glucose-6-phosphate into amyloplasts of heterotrophic tissues for use as a precursor for starch and fatty acid biosynthesis, and as a substrate for the oxidative pentose phosphate pathway (Fischer and Weber, 2002).
JG014682 showed similarity to a regulatory subunit CK2β3 of kinase CK2 and was induced at T2 in TCP02-4589. Several kinases have been reported to be regulated by drought conditions in sugarcane (Rocha et al., 2007; Rodrigues et al., 2009). Plant kinase CK2 protein, also known as casein kinase II, is involved in many different processes such as, DNA transcription, RNA translation and cell-cycle regulation (Riera et al., 2001; Espunya et al., 2005).
JG014675 was slightly induced at T2 in TSP05-4 and showed similarity to a drought-inducible protein mRNA (SoDip22) in Saccharum officinarum (Sugiharto et al., 2002). Because of the hydrophilic nature of SoDip22, and since the signaling pathway for its induction is, at least in part, mediated by ABA, it is plausible that it belongs to the abscisic acid, stress and ripening-induced (Asr) protein family and functions in drought adaptation.
In conclusion, leaf photosynthesis, leaf greenness index, variable-to-maximum chlorophyll a fluorescence ratio, root-to-shoot ratio, stalk height, and stalk weight of two sugarcane genotypes responded to water deficit stress in a manner that is consistent with their classification as drought tolerant or sensitive. Water deficit effects were detected earlier in the sensitive genotype TCP02-4589, since a higher number of differentially-expressed, transcript-derived fragments were detected in this genotype. The fast and complete recovery of physiological processes and gene expression patterns after re-watering demonstrate a high degree of physiological and molecular plasticity in response to changing water conditions.
The authors have not declared any conflict of interest.
A fellowship from the Centro Nacional de Pesquisa e Desenvolvimento (CNPq), Brazil, to C.A. Pedrozo; USDA-NIFA Grants (2009-34461-15661; 2013-67009-21353) and a grant from the Texas AgriLife Research - Bioenergy Initiatives Program to J.L. Jifon are greatly appreciated.
REFERENCES
Ashton F (1956). Effects of a series of cycles of alternating low and high soil water contents on rate of apparent photosynthesis in sugarcane. Plant physiol. 31(4):266-274.
Crossref |
|
Boaretto LF, Carvalho G, Borgo L, Creste S, Landell MGA, Mazzafera P, Azevedo RA (2014). Water stress reveals differential antioxidante responses of tolerant and non-tolerant sugarcane genotypes. Plant Physiol. Biochem. 7:165-175. http://dx.doi.org/10.1016/j.plaphy.2013.11.016
Crossref |
|
Cha-Um S, Kirdmanee C (2009). Proline accumulation, photosynthetic abilities and growth characters of sugarcane (Saccharum officinarum L.) plantlets in response to iso-osmotic salt and water deficit stress. Agricultural Sciences in China. 8(1):51-58.
Crossref |
|
Cia MC, Guimarães ACR, Medici L, Chabregas SM, Azevedo, RA (2012). Antioxidant responses to water deficit by drought-tolerant and sensitive sugarcane varieties. Ann. Appl. Biol. 161(3):313.
Crossref |
|
Espunya MC, López-Giráldez T, Hernan I, Carballo M, Martínez MC (2005). Differential expression of genes encoding protein kinase CK2 subunits in the plant cell cycle. J. Exp. Bot. 56(422):3183-3192.
Crossref |
|
Fischer K, Weber A (2002). Transport of carbon in non-green plastids. Trends in Plant Science 7(8):345-351.
Crossref |
|
Inman-Bamber NG (1995). Automatic plant extension measurement in sugarcane in relation to temperature and soil moisture. Field Crop Res. 42(2-3):135-142.
Crossref |
|
Inman-Bamber NG, Smith DM (2005). Water relations in sugarcane and response to water deficits. Field Crops Res. 92(2-3):185-202.
Crossref |
|
Iskandar HM, Simpson RS, Casu RE, Bonnett GD, Maclean DJ, Manners JM (2004). Comparison of reference genes for quantitative real-time polymerase chain reaction analysis of gene expression in sugarcane. Plant Mol. Biol. Reporter 22:325-337.
Crossref |
|
Jangpromma N, Kitthaisong S, Daduang S, Jaisil P, Thammasirirak S (2007). 18 kDa protein accumulation in sugarcane leaves under drought stress conditions. KMITL Sci. Technol. J. 7(S1):44-54. |
|
Livak KJ, Schmittgen TD (2001). Analysis of relative gene expression data using real-time quantitative PCR and the 2-Δ(ΔCt) method. Methods 25(4):402-408.
Crossref |
|
Lurin C, Andrés C, Aubourg S, Bellaoui M, Bitton F, Bruyère C, Caboche C, Debast C, Gualberto J, Hoffmann B, Lecharny A, Le Ret M, Martin-Magniette M, Mireau H, Peeters N, Renou J, Szurek B, Taconnat L, Small Ian (2004). Genome-wide analysis of Arabidopsis pentatricopeptide repeat proteins reveals their essential role in organelle biogenesis. Plant Cell. 16(8):2089-2103.
Crossref |
|
Matin MB, Fegurson H (1989). Leaf water potential, relative water content, and diffusive resistance as screening techniques for drought resistance in barley. Agron. J. 81(1):100-105.
Crossref |
|
Meierhoff KF, Nakamura T, Bechtold N, Schuster G (2003). HCF152, an Arabidopsis RNA binding pentatricopeptide repeat protein involved in the processing of chloroplast psbB-psbT-psbH-petB-petD RNAs. Plant Cell 15:1480-1495.
Crossref |
|
Naidu KM (1976). Annual Report, Sugarcane Breeding Institute: India. |
|
Riera M, Peracchia G, Pagès M (2001). Distinctive features of plant protein kinase CK2. Mol. Cell. Biochem. 227(1-2):119-127.
Crossref |
|
Rocha FR, Papini-Terzi FS, Nishiyama Jr MY, Vêncio RZN, Vicentini R, Duarte RDC, Rosa Jr VE, Vinagre F, Barsalobres C, Medeiros AH, Rodrigues FA, Ulian EC, Zingaretti SM, Galbiatti JA, Almeida RS, Antonio VOF, Hemerly AS, Silva-Filho MC, Menossi M, Souza GM (2007). Signal transduction-related responses to phytohormones and environmental challenges in sugarcane. BMC Genomics 8(71):1-22.
Crossref |
|
Rodrigues FA, Laia ML, Zingaretti SM (2009). Analysis of gene expression profiles under water stress in tolerant and sensitive sugarcane plants. Plant Sci.176:286-302.
Crossref |
|
Silva MA, Soares RAB, Landell MGA, Campana MP (2008). Agronomic performance of sugarcane families in response to water stress. Bragantia 67(3):655-661.
Crossref |
|
Sugiharto B, Ermawati N, Mori H, Aoki K, Yonekura-Sakakibara K, Yamaya T, Sugiyama T, Sakakibara H (2002). Identification and characterization of a gene encoding drought-inducible protein localizing in the bundle sheat cell of sugarcane. Plant Cell Physiol. 43(3):350-354.
Crossref |
|
Zingaretti S, Demore P, Morceli T, Mantovanini L (2014). Glycine betaine biosynthesis genes differentially expressed in sugarcane under water stress. BMC Proceedings 8(S4):123.
Crossref |