ABSTRACT
The study purified and investigated the physicochemical properties of rhodanese (a cyanide detoxifying enzyme) synthesized by Bacillus cereus. This was with a view to producing an industrially important enzyme. The bacterial strain was identified as B. cereus by sequencing of its 16SrRNA gene. B. cereus rhodanese was purified with a fold of 3.53, yield of 36.80% and specific activity of 25.30 µmol/min/mg protein. The molecular weight determined on SDS-PAGE was 33.800 kDa. The enzyme exhibited maximum activity at 9.0 pH and 50°C. The Kms of B. cereus rhodanese for sodium thiosulphate and potassium cyanide were 19.9 ± 1.05 and 31.4 ± 1.55 mM respectively, while Vmax were 6.19 ± 0.40 and 4.83 ± 0.93 RU/ml respectively. The substrate specificity study using different sulphur compounds showed that the enzyme prefers sodium thiosulphate. The enzyme showed stability at a temperature range of 40-50°C. At 10 mM concentration, metals such as (BaCl2, CaCl2, MnCl2, and SnCl2) had little influence on the enzyme activity while NaCl and HgCl2 inhibited enzyme activity. The presence and biochemical properties of B. cereus rhodanese suggest its possible application in cyanide detoxification.
Key words: Bacillus cereus, isolate, cyanide, purification, rhodanese, kinetics.
Cyanide strong affinity for metal cations makes it a favourable agent in the mining and electroplating industries (Dash et al., 2009). It is highly toxic to living organisms (Dursun et al., 1999). Cyanide, a potent inhibitor of cytochrome oxidase binds tightly to terminal oxidase (Porter et al., 1983). The release of cyanide to the environment can cause rapid breathing, nerve damage and other neurological effects (Department of Interior U.S Cyanide Fact Sheet, 2002). Industrial activities such as metal extraction, metal plating, and synthetic fibres production can increase the concentration of cyanide in the environment (Campos et al., 2006). Free and complex cyanide compounds are found in both environmental matrices and waste streams. The high metabolic inhibition potential of free cyanide classified it as the most toxic form (Knowles and Bunch, 1986). The oxidative product of cyanide such as cyanate (OCN-) can be produced during treatment of cyanide-containing effluents. It has been estimated that the release of cyanide from industrial processes is above 14 million kg/year and these effluents contain a high concentration of cyanide (Gurbuz et al., 2009).
Physical and chemical processes have been used in the treatment of effluents. However, the reagents and chemicals used in such treatment process are toxic and the end products of such processes required additional treatment prior to disposal (Behnamfard and Salarirad, 2009). Biodegradation method of cyanides removal is more efficient because it is more economical and has less operative cost (Akcil, 2003; Desai and Ramakrishna, 1998; Young and Jordan, 1995). Microorganisms can degrade cyanide into non-toxic products (thiocyanate). Organisms such as Bacillus sp. are resistant to cyanide even at high concentrations (Chen, 2003; Kao et al., 2003; Ebbs, 2004).
Rhodanese is an enzyme reported to be involved in cyanide detoxification in microorganisms (Raybuck, 1992; Colnaghi et al., 1996). Enzymatic bioremediation maybe prefers to the use of microorganism because proteins are not affected by inhibitors of microbial metabolism (Gianfreda and Rao, 2010; Rao et al., 2010). Rhodanese is one of the enzymes reported to be involved in cyanide detoxification (Westley, 1981; Agboola and Okonji, 2004). Proper treatment of cyanide-containing effluent is necessary to protect the environment and water bodies from cyanide toxicity. The presence and need for the cyanide detoxifying enzyme, such as rhodanese can therefore not be overemphasized. This study, therefore, aimed to purify and characterize rhodanese produced by a bacterium isolated from the effluents of Iron and Steel Smelting Company with the view to evaluating its potentials for applications in bioremediation of the cyanide-stressed environment.
Collection of samples
Effluents were gotten from Iron and Steel Smelting Company located at Fashina, Ile-Ife, Osun State, Nigeria (7°29’37”N 4°28’33’’E) using a 200 ml sterile bottle by submerging the bottle to a depth of about 20 cm, with the mouth facing slightly upwards below the surface of the water. The samples were labelled, put in ice packs, transported to the Laboratory and analysed within 1 h.
Isolation and screening for Rhodanese production
One millilitre of the water sample was serially diluted and a loopful of dilution 10-8 was plated on modified Bushnell Hass agar and incubated inverted at 37°C for 96 h to select for cyanide degrading bacteria. To screen for rhodanese production, the method of Zlosnik and Williams (2004) was used. Briefly, the isolates were grown in 100ml of a growth medium containing 0.3% KCN, 1% bacteriological peptone, 0.5 % NaCl and 0.5 % yeast extract, at pH of 9.5. The growth media prepared were inoculated with 1 ml of 0.5 McFarland standardized cells suspension. After incubation for 48 h at 30oC, the culture media were checked for rhodanese activity.
Isolates identification and characterization
The different isolates obtained were screened for their cyanide degrading abilities. The most productive strain was selected, identified using Bergey’s manual of determinative bacteriology and by sequencing its 16S rRNA gene. The molecular analyses were carried out using molecular techniques and equipment available at the Bioscience Centre of the International Institute of Tropical Agriculture (IITA), Ibadan, Oyo State, Nigeria. Extraction of bacteria DNA was carried out using modified method of Trindade et al. (2007).
Polymerase chain reaction (PCR) amplifications
Assay mixture for PCR amplification consists of 4 µl of the DNA solution, 0.4 µl of 10 mM dNTPs, 2 µl of 25 mM MgCl2, 1 µl of 10 pmol each of primer (Forward 5’- CCAGCAGCCGCGGTAATACG -3’ and Reverse 5’-ATCGGCTACCTTGTTACGACTTC -3’), 0.24 µl of Taq polymerase (1 U/µl) (Promega USA) and the 5 µl of 5× PCR buffer. Sterile DNase free water was added to make a volume of 25 µl. The PCR amplicons were visualized using 1.5% agarose gel electrophoresis. Sequence similarity search of the GenBank data was done using the National Centre for Biotechnology and Information (NCBI) Basic Alignment Search Tool (BLAST) program.
Enzyme assay
Rhodanese activity was measured using sodium thiosulphate and potassium cyanide as substrates. The assay mixture consists of borate buffer (pH 9.4, 50 mM), 0.25 M KCN, 0.25 M sodium thiosulphate and 0.1 ml of the enzyme in a final volume of 1 ml. After 1 min of incubation at 37°C, the reaction was terminated by adding 0.5 ml 15% formaldehyde. Concentration of thiocyanate produced was determined by the addition of 1.5 ml Sorbo reagent (which is made up of 10 g Fe (NO3)2. 9H2O, 20 ml HNO3 and 80 ml distilled water) (Sorbo, 1953b). The absorbance of the reaction medium was taken at 460 nm. The unit of rhodanese activity (RU) is defined as the micromoles of product (thiocyanate) formed in one minute. The protein concentration was determined by the method of Bradford (1976), standard used was bovine serum albumin (BSA).
Rhodanese purification on CM-Sephadex C-50 and Biogel P-100
To purify rhodanese produced, all the cultures were pooled together at 39th h of incubation. Bacterial cells were removed by centrifugation. The filtrate was subjected to 80% ammonium sulphate saturation and left in the refrigerator overnight. 5 ml of the concentrated enzyme was layered on CM-Sephadex c-50 column. Fractions were collected at a flow rate
of 36 ml/h. Protein and rhodanese activity in the fractions were determined. Pooled active fractions were dialyzed against 50% glycerol in 100 mM phosphate buffer pH 6.5. 5 ml of the active fraction was layered on Biogel P-100 column equilibrated with 100 mM phosphate buffer pH 6.5. Fractions of 2 ml were collected from the column at 20 ml/h and monitored for protein and rhodanese activity.
Molecular weight determination
The molecular weight of native rhodanese was determined on Sephadex G-100 (2.5 x 90 cm) using the marker proteins Lysozyme (14.5kDa), α Chymotrypsinogen (25 kDa), Ovalbumin (45 kDa) and BSA (66 kDa). The elution volume (Ve) of Blue Dextran (2 mg/ml) was used to estimate the void volume (Vo) of the column. The elution volume of rhodanese was estimated by layering 10 ml of the enzyme solution on the same column.
Determination of Subunit Molecular Weight using SDS-PAGE
The method of Weber and Osborn (1975) was used to determine the subunit molecular weight of the purified enzyme on a 10% slab gel apparatus with a notched glass plate. Gels of 1.5 mm thickness were prepared using perplex spacers of the same size.
Kinetic studies
The purified enzyme kinetics parameters (Km and Vmax) were determined using KCN and Na2S2O3 as substrates. Km and Vmax values for KCN were determined using Lineweaver and Burk plot (1934) obtained by varying the concentration of KCN in the reaction medium between 20 mM and 100 mM at a fixed concentration of Na2S2O3. Concentration of Na2S2O3 was varied between 20 mM and 100 mM at a fixed concentration of KCN.
Substrate specificity of the purified enzyme
Different sulphur containing compounds were used as substrates in a typical rhodanese assay mixture. Rhodanese activity was measured as earlier described. The relative activities were compared with sodium thiosulphate which was taken as control.
Effect of pH and temperature on the purified enzyme
The influence of pH on the enzyme activity was determined by assaying for the enzyme in different buffer solutions. Also, the effect of temperature was checked by varying the temperature of the assay mixture between 30 and 80°C at 10°C interval.
Heat stability of the purified enzyme
Thermal stability of the enzyme was tested by incubating aliquot of the enzyme at a particular temperature for 60 min, at 10 min interval, the enzyme solution was assayed for residual activity.
Effect of salts on the purified enzyme activity
The influence of various salts on B. cereus rhodanese was investigated. The tested salts were BaCl2, CaCl2, HgCl2, MnCl2, SnCl2, KCl and NaCl at 1 mM and 10 mM in assay mixture.
The isolated strain, B. cereus, share a maximum of 98% homology with B. cereus KX65992 and 97% homology with B. cereus KF973315. The agarose gel of 16S rRNA amplicon band of isolate is shown in Figure 1. The presence of rhodanese has been identified in different species of bacteria such as Escherichia coli (Ray et al., 2000), Azotobacter vinelandii (Kaewkannetra et al., 2009), Bacillus brivis (Oyedeji et al., 2013) and P. aeruginosa (Cipollone et al., 2008). B. cereus rhodanese was purified with a fold of 3.53, yield of 36.80% and a specific activity of 25.30 µmol/mg of protein. A summary of purification results is presented in Table 1. The elution profiles for CM Sephadex c-50 ion-exchange chromatography and Biogel P-100 are shown in Figures 2 and 3, respectively. The specific activity of Rhodanese as determined by different researchers varied between 5.21 to 131 RU/mg (Fagbounka et al., 2004; Ehigie et al., 2015).
Enzyme activity and protein concentration were determined after each step. Activity was determined using 0.25 mM KCN, 0. 25 mM Na2S2O3 and 50 mM Borate buffer pH 9.4. Protein was determined using Bradford’s method.
The molecular weight of native and denatured rhodanese were determined using the marker proteins Lysozyme (14.5kDa), α Chymotrypsinogen (25 kDa), Ovalbumin (45 kDa) and BSA (66 kDa) to be about 34,000 Da by Gel filtration and SDS PAGE, respectively indicating that the enzyme is of monomer in nature. The calibration curve for the determination of the native molecular weight of B. cereus rhodanese is shown in Figure 4 while the electrophoresis pictogram and calibration curve obtained for the sodium dodecyl sulphate polyacrylamide gel electrophoresis (SDS PAGE) are shown in Figures 5 and 6, respectively. Studies have shown that rhodanese molecular weight falls between 31 to 37 kDa (Akinsiku et al., 2010; Saidu, 2004; Lee et al., 1995; Okonji et al., 2015). A molecular weight of 34,800 and 35,700 Dalton were reported for rhodanese isolated from mouse and fruit bat (Eidolon helvum, Kerr) liver respectively (Lee et al., 1995). Akinsiku et al. (2010) reported a weight of 34.5 and 36.8 kDa for African catfish liver rhodanese.
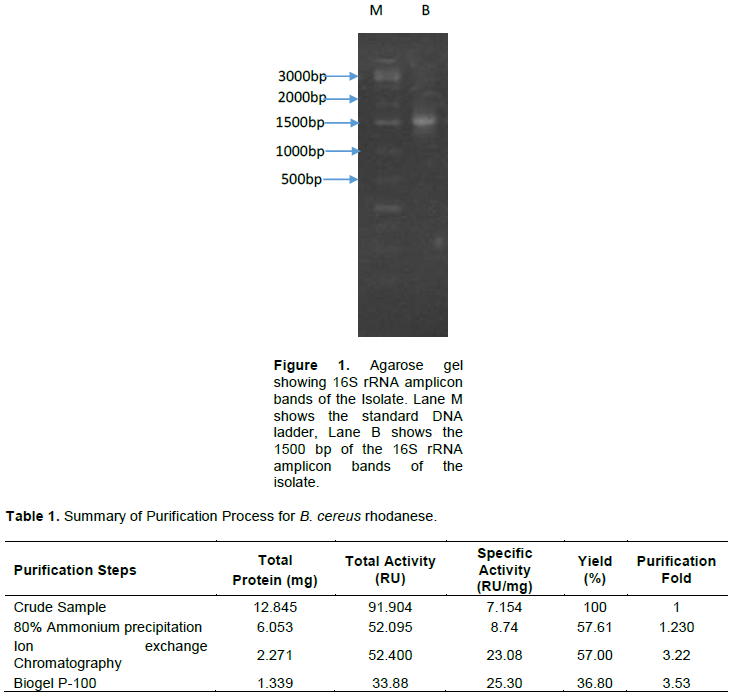
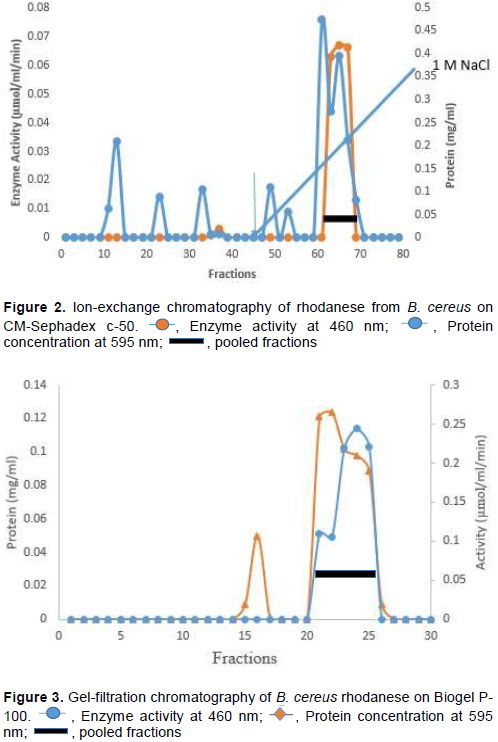
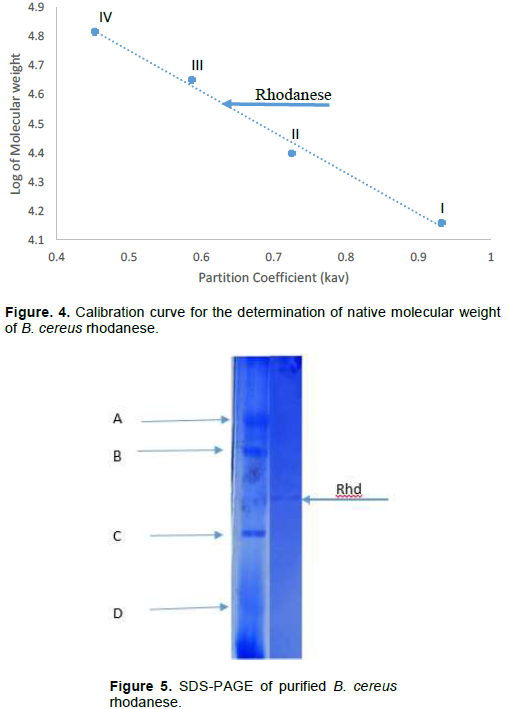
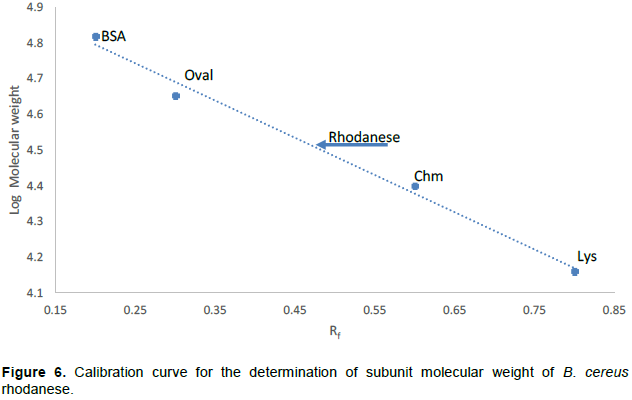
The molecular weight of rhodanese from B. cereus was determined on Sephadex G-100 Column (2.5 × 90 cm).
The standard proteins were: I = Lysozyme (14.5kDa), II = α Chymotrypsinogen (25 kDa), III = Ovalbumin (45 kDa) and IV = BSA (66 kDa)
The standard molecular weight markers used included: D = Lysozyme (14.5 kDa), C = α-Chymotrypsin (25.0 kDa), B = Ovalbumin (45.0 kDa), and A = BSA (66.0 kD). Rhd = Purified B. cereus rhodanese.
The standard molecular weight markers include: Lysozyme (14.3kDa), α-Chymotrypsin (20.0 kDa), Ovalbumin (45.0 kDa) and BSA (66.0 kDa).
The Km values of B. cereus rhodanese for both substrates were 31.4 ± 2.08 and 19.9 ± 1.05 mM for KCN and Na2S2O3 respectively. The kinetic data are summarised in Table 2. The Km values for B. cereus rhodanese falls within the range reported from other sources. Km values as determined by different researches were varied between 13.5 to 78 mM (Keith and Volini, 2000; Hossein and Reza, 2011; Agboola and Okonji, 2004). Apparent Km values of 36.81 and 19.84 mM were reported for rhodanese in Liver of Rainbow (Hossein and Reza, 2011). Escherichia coli rhodanese was reported to have apparent Km values of 78 mM and 17 mM for KCN and Na2S2O3 respectively (Keith and Volini, 2000). Km values obtained in this study indicate that the enzyme has an affinity for thiosulphate and this could clarify how the organism is able to thrive in the cyanogenic environment.
Table 3 summarizes the results of percentage residual activity of the different sulphur containing compounds as substrates. The substrate specificity study showed that rhodanese from B. cereus had a preference for thiosulphate for its catalytic activity. B. cereus rhodanese was inactivated by 2-mercaptoethanol and sodium metabisulfite. Rhodanese ability to use different sulphur-containing compounds was reported by Okonji et al. (2008). The use of sulphane sulphur in rhodanese cyanide detoxification mechanism was reported by Westley (1981). Chew and Boey (1972) reported the inactivation of rhodanese from tapioca leaf by 2-mercaptoethanol.
Optimum B. cereus rhodanese activity was obtained at pH 9.0 and 50°C (Figures 7 and 8). The enzyme retained activity up to 50°C for 20 min (Figure 9). The pH value obtained in this study is similar to rhodanese from other sources. pH range of 8.0-11.0 has been reported by different researchers (Lee et al., 1995; Hossein and Reza, 2011). Oyedeji et al. (2013) reported an optimum pH range of 5.5-9.5 for rhodanese from Pseudomonas aeruginosa and Bacillus brevis respectively. Optimum temperature of 50 and 40°C were reported for the P. aeruginosa and B. brevis rhodanese respectively. At 60 and 70°C, the enzyme lost about 50 and 80% of its activity respectively. This value is in agreement with heat stability study of rhodanese from other sources.
Metals salts such as BaCl2, CaCl2, MnCl2, KCl, and SnCl2 showed little effect at 1 mM and 10 mM concentration while NaCl and HgCl2 inhibited the enzyme at 10 mM concentration (Table 4). The observed activity suggests consistent exposure to these metals by the organism. The inhibition of B. cereus rhodanese by Hg2+ and Na+ was likely because of the reaction of these metal ions with the active site residues of the enzyme which may prompt the change in the functional structure of the enzyme. The influence of metal ions on enzyme structures has been reported (Tayefi-Nasrabadi et al., 2006). Agboola and Okonji (2004) reported the inhibition of rhodanese from fruit bat liver by Hg2+ ion. Several other researchers have reported the influence of metal ions on rhodanese (Fagbounka et al., 2004).
Industrial effluents contain a high concentration of cyanide which is toxic to the aquatic life if discharged to the environment without proper treatment. In this study, a cyanide degrading bacterium was isolated from an industrial effluents and subjected to rhodanese production. The biochemical and catalytic properties of rhodanese produced suggests the involvement of the enzyme in cyanide degradation mechanism of the organism, a function that can be employed in the treatment of cyanide-containing effluents.
The authors have not declared any conflict of interests.
REFERENCES
Agboola FK, Okonji, RE (2004). Presence of rhodanese in the cytosolic fraction of the fruit bat (Eidolon helvum) Liver. Journal of Biochemistry and Molecular Biology 37(3):275-281.
|
|
Akcil A (2003). Destruction of cyanide in gold mill effluents: biological versus chemical treatments. Biotechnology Advance 21:501-511.
Crossref
|
|
|
Akinsiku O, Agboola F, Kuku A, Afolayan A (2010). Physicochemical and kinetic characteristics of rhodanese from the liver of African catfish Clarias gariepinus Burchell in Asejire Lake. Fish Physiology and Biochemistry 36:573-86.
Crossref
|
|
|
Behnamfard A, Salarirad MM (2009). Equilibrium and kinetic studies on free cyanide adsorption from aqueous solution by activated carbon. Journal of Hazardous Materials 170(1):127-133.
Crossref
|
|
|
Bradford KM (1976). A rapid and sensitive method for the quantitation of micrograme quantities of protein utilizing the principle of protein-dye binding. Analytical Biochemistry. 72: 248-254.
Crossref
|
|
|
Campos MG, Pereira P, Roseiro JC (2006). Packed-bed reactor for the integrated biodegradation of cyanide and formamide by immobilised Fusarium oxysporum CCMI 876 and Methylobacterium sp. RXM CCMI 908, Enzyme Microbiology Technology 38:848-854
Crossref
|
|
|
Chen SC (2003). Biotransformation of cyanide to methane and ammonia by Klebsiella oxytoca. Chemosphere 50:1055-1061.
Crossref
|
|
|
Chew MY, Boey CG (1972). Rhodanese of tapioca leaf. Phytochemistry 11: 167-160
Crossref
|
|
|
Cipollone R, Ascenzi P, Tomao P, Imperi F, Visca P (2008). Enzymatic detoxification of cyanide: Clues from Pseudomonas aeruginosa rhodanese. Journal of Molecular Microbiology and Biotechnology 15(2-3):199-211.
Crossref
|
|
|
Colnaghi R, Pagani S, Kennedy C, Drummond M (1996). Cloning, sequence analysis and overexpression of the rhodanese gene of Azotobacter vinelandii. The FEBS Journal 236(1):240-248.
|
|
|
Dash RR, Balomajumder C, Kumar A (2009). Removal of cyanide from water and wastewater using granular activated carbon. Chemical Engineering Journal 146(3):408-413.
Crossref
|
|
|
Department of Interior US Cyanide Fact Sheet (2002). Bureau of Reclamation, Technical Service Center, Water Treatment Engineering and Research Group. pp. 1-4.
|
|
|
Desai JD, Ramakrishna C (1998). Microbial degradation of cyanides and its commercial application. Journal of Science India 57:441-453
|
|
|
Dursun AY, Alik AC, Aksu Z (1999). Degradation of ferrous (II) cyanide complex ion by Pseudomonas fluorescens. Process Biochemistry 34:901-908.
Crossref
|
|
|
Ebbs S (2004). Biological degradation of cyanide compounds. Current Opinions in Biotechnology 15(3):231-236.
Crossref
|
|
|
Ehigie lO, Okonji RE, Ehigie AF (2015). Purification and characterization of rhodanese from the leave of bitter melon (momordica charantia). 3(5):47-58
|
|
|
Fagbounka BS, Adenuga GA, Okonji RE, Agboola FK (2004). Properties of rhodanese from hepatopancreas of giant snail, Archachatina marginata. Science Focus 1:76-80.
|
|
|
Gianfreda L, Rao MA (2010). Potential of extra cellular enzymes in remediation of polluted soils: a review. Enzyme and Microbial Technology 35(4):339-354.
Crossref
|
|
|
Gurbuz F, Ciftci H, Akcil A (2009). Biodegradation of cyanide containing effluents by Scenedesmus obliquus. Journal of Hazardous Materials 162(1):74-79.
Crossref
|
|
|
Hossein T, Reza R (2011). Some biochemical properties of rhodanese from liver of rainbow trout. International Conference of Medical, Biological and Pharmaceutical Sciences 1:1-3
|
|
|
Kaewkannetra KT, Imai FJ, Garcia G, Chiu SC (2009). Cyanide removal from cassava mill wastewater using Azotobacter vinelandii TISTR 1094 with mixed microorganisms in activated sludge treatment system. Journal of Hazardous Materials 172:224-228.
Crossref
|
|
|
Kao CM, Liu JK, Lou HR, Lin CS, Chen SC (2003). Biotransformation of cyanide to methane and ammonia by Klebsiella oxytoca. Chemosphere 50:1055-1061
Crossref
|
|
|
Keith A, Volini M (2000). Properties of E. coli Rhodanese. Journal of Biology Chemistry 262(14):6595-6604
|
|
|
Knowles CJ, Bunch AW (1986). Microbial cyanide metabolism. Advanced Microbiology Physiology 27:73-111.
Crossref
|
|
|
Lee CH, Hwang JH, Lee YS, Cho KS (1995). Purification and Characterization of mouse liver rhodanese. Journal of Biochemistry and Molecular Biology 28:170-176.
|
|
|
Lineweaver H, Burk D (1934). The determination of enzyme dissociation constants. Journal of the American Chemical Society 56: 658-666.
Crossref
|
|
|
Okonji RE, James IE, Madu JO (2015). Fagbohunka, B. S. and Agboola, F. K. Purification and characterization of rhodanese from the hepatopancreas of garden snail, limicolaria flammea. Ife Journal of Science. 17 (2):289-303.
|
|
|
Okonji RE, Aladesanmi OT, Kuku A, Agboola FK (2008). Isolation and properties of partially purified rhodanese from hepatopancreas of fresh water prawn (Macrobrachium rosenbergii de Man). Ife Journal of Science. 10 (20): 255 -262.
|
|
|
Oyedeji O, Awojobi KO, Okonji RE, Olusola OO (2013). Characterization of rhodanese produced by Pseudomonas aeruginosa and Bacillus brevis isolated from soil of cassava processing site. African Journal of Biotechnology 12(10):1104-1114.
|
|
|
Porter N, Drozd JW, Linton JD (1983). The effects of cyanide on the growth and respiration of Enterobacter aerogenes in continuous culture. Microbiology 129:7-16.
Crossref
|
|
|
Rao MA, Scelza R, Scotti R, Gianfreda L (2010). Role of enzymes in the remediation of polluted environments. Journal of Soil Science and Plant Nutrition 10(3):333-353.
Crossref
|
|
|
Ray R, Padmaya G, Balogopalan C (2000). Extracellular Rhodanese Production by Rhizopus oryzae. Zentralblatt Mikrobiologie 145(4):259-268.
|
|
|
Raybuck SA (1992). Microbes and microbial enzymes for cyanide degradation. Biodegradation 3:3-18.
Crossref
|
|
|
Saidu Y (2004). Physicochemical features of rhodanese: A review. African Journal of Biotechnology 3(4):370-374.
|
|
|
Sorbo BH (1953b). Crystalline Rhodanese enzyme catalyzed reaction. Acta Chemica Scandinavica 7:1137-1145.
Crossref
|
|
|
Tayefi-Nasrabadi H, Keyhani E, Keyhani J (2006). Conformational changes and activity alterations induced by nickel ion in horseradish peroxidase Biochimie 88(9):1183-1197.
Crossref
|
|
|
Trindade LC, Marques E, Lopes DB, Ferreira MA (2007). Development of a molecular method for detection and identification of Xanthomonas campestris pv. viticola. Summa Phytopathologica 33(1):16-23.
Crossref
|
|
|
Westley J (1981). Methods in y. (S.P. Colowick and N.O. Kaplan, eds.) Academic Press, New York 77:285-291.
|
|
|
Young CA, Jordan TS (1995). Cyanide Remediation: Current and Past Technologies. In: Kabunde KJ, chair Chemical Destruction. Proceedings of the 10th annual conference on hazardous waste research pp. 104-129.
|
|