ABSTRACT
This study assessed the health risks of organochlorine pesticides in fish products from Lake Victoria. Nine organochlorine pesticides (α- Hexachlorocyclohexane (HCH), β- HCH, γ- HCH (Lindane), Hexachlorobenze (HCB), Dieldrin, p, pꞌ- DDE, α- endosulfan, Oxychlordane and α- chlordane) were identified at variable levels in fish products. Of the nine organochlorine pesticides which were identified, p, pꞌ- DDE (3.73µg/kg) was the highest in deep fried Nile tilapia while the lowest amount was α- HCH (0.14 µg/kg) in smoked Nile tilapia. In the smoked products, several of the organochlorine pesticides were detected at high concentrations. This is attributed to the fact that organochlorine pesticides are fat soluble and therefore found in the lipid phase of the fish during smoking. There were high levels and prevalence of p, pꞌ- DDE, α- endosulfan, HCB, Dieldrin and Lindane in deep fried Nile tilapia compared to other fish products probably due to environmental contamination. However, the levels of organochlorine pesticides in this study are below the maximum residual limits (MRLs) set by FAO/WHO for fish products indicating that the fish products were safe for human consumption and do not present health risks in terms of organochlorine pesticides residues.
Key words: Cancer risk, hazard index, Kayabo, organochlorine pesticides, trims.
Organochlorine pesticides (OCPs) such as Dichlorodiphenyltrichloroethane (DDTs), Hexachlorocylohexanes (HCHs), Hexachlorobenzene (HCB), Aldrin, Dieldrin and Endosulfans are products
used for plant protection, pest control and preservation of foodstuffs during storage, and the residues derived from their use may be found in food products (Biego et al., 2010). They have also been used extensively in mosquito, termite and tsetse fly control programs (Farshid et al., 2012). In Tanzania, endosulfans are still used for agricultural purposes such as in cotton fields and horticultural production while DDTs are used to control mosquito populations to fight malaria (Henry and Kishimba, 2006; Polder et al., 2014; Mahugija et al., 2018). Organochlorine compounds are highly lipophilic (Farshid et al., 2012; Polder et al., 2014), persist in the environment (El-Mekkawi et al., 2009; Ssebugere et al., 2014a), readily transported over longer distances and tend to bioaccumulate through the food chain (Guzzella et al., 2005; Henry and Kishimba, 2006; Biego et al., 2010). The compounds accumulate in living organisms and are known for their carcinogenic, mutagenic and teratogenic effects. They also affect the nervous, immune, reproductive, renal and hepatic systems (Biego et al., 2010; Polder et al., 2014).
Lake Victoria is one of the most important water resources in Tanzania and neighbouring countries. The local communities dwelling around the lake use it as a major source of household and industrial water supply, irrigation water for agricultural production, facilitates boat transport and provides for fishing as a main source of animal derived protein as well as income. Several agricultural related activities involving the use of pesticides are practiced in large areas around the Lake Victoria basin (Polder et al., 2014). There are also numerous rivers and streams crossing through the fields discharging into the Lake Victoria basin suggesting that they may carry pesticides into the lake. Thus, Lake Victoria is receiving a considerable amount of pollution because of anthropogenic activities that are undertaken along the lake such as agricultural and industrial activities (Ssebugere et al., 2014a). In addition to that, due to the solubility of the pesticides in fat and lipids, fish and other aquatic organisms concentrate and accumulate pesticide residues in their fatty tissues (Mahugija et al., 2018).
Studies to assess the chemical and microbiological quality of fish and fishery products in Lake Victoria have focused mainly on fish products that are intended for export to Europe and other developed countries (Manirakiza et al., 2002; Henry and Kishimba, 2006; Kasozi et al., 2006; Darko et al., 2008; Kirema–Mukasa, 2013; Polder et al., 2014; Ssebugere et al., 2014b; Oluochi-Otiego et al., 2016; Baniga et al., 2017). Some of the studies have as well been limited in scope as they have been focusing on only few persistent organochlorine compounds (Henry and Kishimba, 2006; Polder et al., 2014). Few studies have documented the quality of fishery products such as salted and sundried Nile perch (Kayabo) in terms of microbiological quality and physico-chemical properties (Baniga et al., 2019). These products are highly consumed by low and middle-income communities in domestic and regional markets in countries like the Democratic Republic of Congo, Rwanda, Burundi, Malawi, Kenya and Uganda (LVFO, 2013).
This study aimed to determine the prevalence and levels of organochlorine pesticides in processed fish products from Lake Victoria as well as the human health risks associated with consumption of such products.
Description of the study area
The study was conducted at the Kirumba fish market in Mwanza between May and August 2017. The market is the largest fish market in the lake zone receiving fish products from all regions on the Tanzanian side of Lake Victoria. Fish folks and processors bring their fish products to the market where they are sold to middle men that then deliver the products to different markets in Tanzania and outside the country, for example in Uganda, DRC, Burundi, Rwanda, Zambia, Kenya and Malawi where they are an important source of protein and income (LVFO, 2013).
Fish samples collection and extraction
Six products of fish samples that is, salted and sundried Nile perch commonly known as Kayabo, trims of Nile perch, smoked Nile perch and Nile tilapia and deep fried products of both Nile perch and Nile tilapia were collected from randomly selected fish processors and sellers at the market. A total of 360 samples of fish products (that is 60 samples of each product) were collected for analysis of OCPs. Extraction and cleanup for determination of OCPs in fish samples were done using a modified quick, easy, cheap, effective, rugged and safe (QuEChERS) procedure at the National Fish Quality Control Laboratory in Mwanza, Tanzania. Thirty grams of each sample were measured in triplicates and homogenized using a blender. Fifteen grams of the homogenized samples were transferred into 100 mL centrifuge tubes as described by Wenaty et al. (2019). Thereafter, 2.5 g of sodium bicarbonate (NaHCO3), 60 mL of ethyl acetate and 15 g of anhydrous Na2SO4 were added and placed in a shaking machine to homogenize for 20 min. The supernatants were transferred into 15 mL centrifuge tubes containing 0.125 g of primary secondary amine (PSA) and 0.75 g of anhydrous MgSO4 (Anastassiades et al., 2003; Wenaty et al., 2019). The mixture was centrifuged at 2500 rpm for 10 min and left to separate for further 5 min. The supernatants were transferred into vials and then transported to Denmark (Technical University of Denmark (DTU)), for analysis. Prior to gas chromatographic (GC) analysis, the supernatants were evaporated with a gently stream of nitrogen to almost dryness and the solvent was changed to isooctane ready for GC analysis.
Chemical analysis
Chemical analysis was performed at the laboratory of Food Analytical Chemistry at the DTU. The collected fish samples were analysed for 19 OCPs namely; p, pꞌ- DDT, o, p- DDT and metabolites p, pꞌ- DDE and p, pꞌ- DDD, α – HCH, β – HCH, γ – HCH (Lindane), HCB, Heptachlor, Heptachlor epoxide, Aldrin, Dieldrin, Endrin, Isodrin, α– Endosulfan, Oxychlordane, γ– Chlordane, α– Chlordane and Transnonachlor. Most of the studied OCPs are listed in the Stockholm Convention on persistent organic pollutants (POPs) for initial elimination and reduction in use because of their adverse effects on the environment as well as living organisms (Polder et al., 2014).
Identification and quantification of organochlorine pesticides
Separation and detection of OCPs were performed on a Hewlett Packard Gas Chromatography (Agilent 6890 Series gas chromatography system; from Agilent Technologies) equipped with an auto sampler (Agilent 7683 Series; from Agilent Technologies). For optimum separation, a dual capillary column system with two separate columns of different polarity and selectivity were used (Chrompac CP – sil 5CB and J and W DB- 17), Nominal length 50 and 60 m respectively, 0.25 mm ID, 0.25 µm film thickness) and coupled to two 63Ni electron capture detectors (Agilent 6890 ECD) as described by Wenaty et al. (2019). The following GC conditions were used; injector temperature: 280°C; injection volume: 2 µL; injector mode: splitless; purge flow: 42 mL/min; purge time: 0.60 min; carrier gas: Helium; constant flow: 2.0 and 1.3 mL/min respectively and make up gas: Nitrogen. The temperature programme was 90°C held for 2.0 min; 30°C/min increased to 170°C held for 7.5 min; 2.0°C/min increased to 185°C; 3.0°C/min increased to 220°C held for 15 min; 3.0°C/min increased to 255°C held for 2.0 min and 5.0°C/min increased to 280°C held for 10 min. The detector temperature was 300°C (Wenaty et al., 2019).
Analytical quality control
To maintain the quality of analytical results, blanks and standards were analysed after every analysis of five samples. The limits of detection (LODs) of the analytes were calculated as concentrations whose peaks were three times the peaks of signal to noise (S/N) ratios while their corresponding limits of quantification (LOQs) were determined as concentrations whose peaks were ten times the peaks of signal to noise (S/N) ratios.
Data analysis
Statistical analysis used for the data analyses include subjecting the measured OCPs data to descriptive statistics in order to deduce the minimum, maximum, mean concentrations and standard deviations of the detected OCPs (SPSS Version 16). Data on OCP concentration were presented as mean ± SD. ANOVA was used to compare concentrations between fish products and the means were separated using Duncan's Multiple Range Test. In data processing, the concentrations of OCPs in samples below the limit of detection (<LOD) were treated as zero. Significant difference was declared at p<0.05 for all analyses.
Risk assessment model
The estimated dose (CDI) received through consumption of fish products was calculated using Equation 1 and the cancer risk (CR) using Equation 2, adopted from the Environmental Protection Agency (USEPA, 1997, 2009) of the United States.
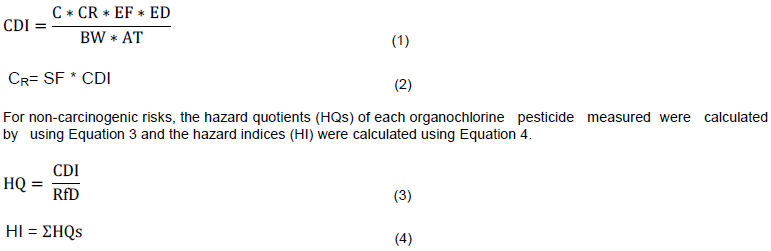
Where: CDI (mg/kg-day) is the estimated chronic daily intake; CR is the cancer risk via consumption of contaminated fish products; C (mg/kg) is the measured concentration of OCPs in fish products; IR (kg/day) is the consumption rate; HI (mg/kg-day) is the hazard index (overall non-cancer risk via consumption of contaminated fish products; HQ (mg/kg-day) is the hazard quotient (individual compound non- cancer risk via consumption of contaminated fish products); EF is the exposure frequency, 365 days/year (USEPA, 2009); ED is the exposure duration, 60 years for adults and 12 years for children (USEPA, 2009); SF is the cancer slope factor in this study, 2 (mg/kg-day)-1 (Ge et al., 2013) for all OCPs detected; RfD is the reference dose (mg/kg-day), varied from one organochlorine pesticide to another; BW is the hypothetical average body weight in this study, 70 kg for adults and 29 kg for children (USEPA, 2001); AT is the averaging time, 60 years × 365 days/year = 21900 days for adults and 12 years × 365 days/year = 4380 days for children (US EPA, 2001; Ge et al., 2013).
Qualitative descriptions of lifetime cancer risks were based on Agency of Toxic Substances and Disease Registry (ATSDR) standards as follows; very low when the estimated value is ≤10E-06, low: 10E-06<value≤10E-04, moderate: 10E-04<value≤10E-03, high: 10E-03<value≤10E-01 and very high when the estimated value is ≥10E-01 (ATSDR, 1995, Ge et al., 2013). For non- carcinogenic risks, HI, calculated as sum of HQs greater than one was considered risky while HI less than one was considered no risk associated with consumption of fish products.
Concentrations of organochlorine pesticides
The concentrations of the detected organochlorine pesticides in the analysed processed fish products are shown in Table 1. Of the 19 OCPs measured, only nine (α- HCH, β- HCH, γ- HCH( Lindane), HCB, Dieldrin, p, pꞌ- DDE, α- endosulfan, Oxychlordane and α- chlordane) were detected. The LODs of the detected components ranged between 0.01 and 0.40 µg/kg whereas their corresponding LOQs ranged between 0.04 and 1.30 µg/kg. Alfa (α- HCH) was detected in salted and sundried Nile perch and smoked products of Nile perch and Nile tilapia. The highest mean concentration was 0.54 µg/kg being detected in salted and sundried products, while the lowest mean concentration was 0.14 µg/kg in smoked Nile tilapia. The trims, deep fried Nile perch and Nile tilapia products had no detectable (<LOD) levels of α- HCH.
Beta (β- HCH) was detected in only one fish product (smoked Nile perch) at 1.43 µg/kg. The other five fish products (smoked Nile tilapia, salted and sundried Nile perch, trims, deep fried products of Nile perch and Nile tilapia) had no detectable (<LOD) concentrations of β- HCH.
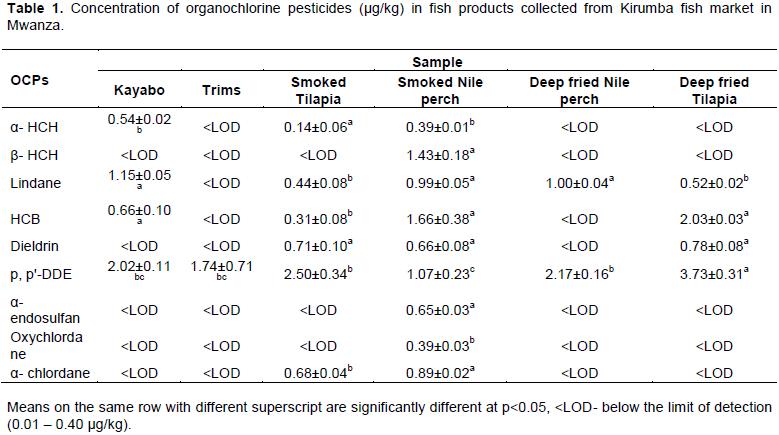
Lindane (γ- HCH) was found at detectable levels in 5/6 fish products including salted and sundried products of Nile perch, smoked products of both Nile perch and Nile tilapia and deep fried products of both fish species. The highest concentration was detected in salted and sundried products at 1.15 µg/kg while the lowest was 0.44 µg/kg, being detected in smoked products of Nile tilapia. Fish trims were free from γ- HCH. The concentrations of Lindane in all fish products were below the maximum residual limit (MRL) of 100 µg/kg set for fishery products (Biogo et al., 2010). The levels of HCHs determined in this study were far higher than those that were determined by Wenaty et al. (2019) in fresh species of L. niloticus and O. niloticus from the same study area. In a study by Wenaty et al. (2019), the levels of α- HCH and γ- HCH were below their lowest limits of detection (LODs) in both fish species while the concentration of β- HCH was up to 1.19 µg/kg in L. niloticus and 0.60 µg/kg in O. niloticus. Detection of high levels of HCHs in processed fish products than fresh fish muscles suggests that contamination occurs during fish processing in the value chain. However, the levels of HCHs detected in this study are far lower than the levels of the same that were detected in the Ugandan side of Lake Victoria where the concentrations ranged between 5.7 and 26 µg/kg, between 7 and 34 µg/kg and between 5 and 13 µg/kg for α- HCH, β- HCH and γ- HCH respectively (Ssebugere et al., 2014 a,b; Wenaty et al., 2019).
The ratio of α – HCH to γ – HCH in the fish products where these components were detected simultaneously ranged from 0.32 to 0.47. The ratios are normally used to establish whether degradation of technical HCH in the environment or food items is efficient and whether there is recent or past application of technical HCH in the study area. High ratios of α – HCH/ γ – HCH ranging from 4 to 7 are used to indicate fresh inputs of technical HCH in the environment or food items, while lower (<3) ratios suggest a historical use of Lindane in the area from which the samples originated (Yi et al. (2013) and Ssebugere et al. (2014a, b). The low ratios found in this study (0.32 – 0.47) suggest past exposure of the fish products to Lindane. Furthermore, the sum of HCHs concentrations found in the fish products were below the extraneous maximum residue limit (EMRL) of 5000 µg/kg set by FAO/WHO Codex Alimentarius Commission for fish and other fisheries products (FAO/WHO, 1997). The fish products that were considered in this study are therefore safe for human consumption with reference to HCHs residues.
Hexachlorobenzene (HCB) was observed in four out of six fish products as presented in Figure 1. There were high concentrations of HCB in deep fried Nile tilapia (2.03 µg/kg) compared to other fish products, while the lowest detectable levels of HCB were detected in smoked Nile tilapia (0.31 µg/kg). The fish trims and deep fried Nile perch had no detectable (<LOD) levels of HCB. The highest concentration of HCB in this study was about four times higher than the highest concentration of HCB in fresh fish muscles in another study where 0.59 µg/kg was found (Wenaty et al., 2019). The levels of HCB in this study were also in the same range as those detected by Polder et al. (2014) from four different lakes in Tanzania. The study by Polder et al. (2014) reported HCB levels in O. niloticus ranging from 1.4 to 4.0 µg/kg (L. Victoria), 1.1 to 1.3 µg/kg (L. Tanganyika), 1.2 to 2.9 µg/kg (L. Nyasa) and 0.6 to 2.8 µg/kg (L. Babati). It was further observed that the levels of HCB in Tanzanian fish is due to long-range atmospheric transport rather than local sources as there have been no historical use of HCB in Tanzania. The levels of HCB were far lower than the tolerable limits of 200 µg/kg recommended by FAO/WHO suggesting that the fish products are safe for human consumption with respect to HCB levels. HCB is generally used as a pesticide and generated as a byproduct in industrial productions (Wang et al., 2013). Since there is no historical use of HCB in Tanzania (Polder et al., 2014), it may be that its presence in food items is due to its volatile nature and long-range atmospheric transport (Wenaty et al., 2019).
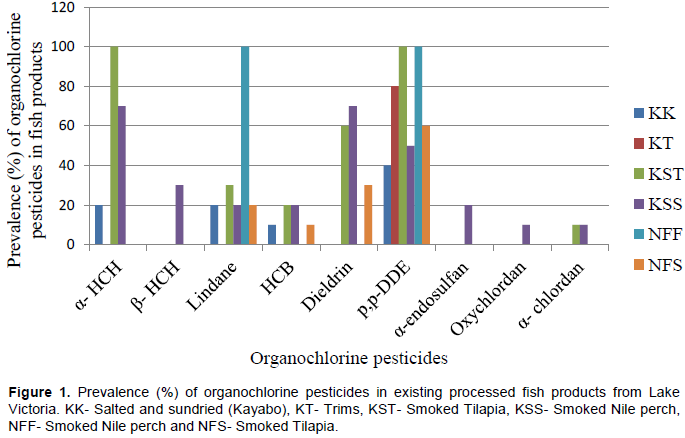
Of the four cyclodienes analysed, only Dieldrin was detected in smoked products of Nile perch and Nile tilapia and deep fried products of Nile tilapia as shown in Table 1. The deep fried products contained higher concentrations (0.78 µg/kg) compared to other products, whereas smoked Nile perch had the lowest mean concentration (0.66 µg/kg) though the difference was not significant. Salted and sundried fish, trims and deep fried Nile perch products had no traces of Dieldrin. Aldrin and other drin residues were not detected in any fish products. This is because Aldrin is converted to Dieldrin once it enters the environment or the body of an organism by the action of sunlight and bacteria. Dieldrin is resistant to bacterial and chemical breakdown (Orris et al., 2000; Afful et al., 2013). Other drins such as Isodrin and Endrin are also unstable in the environment. The ratios of the detected Dieldrin to Aldrin residues (Dieldrin/Aldrin) are usually used to ascertain whether the detected residues are due to current applications of Aldrin or not (Madadi et al., 2006; Wenaty et al., 2019). Ratios <1 indicate current applications of Aldrin while ratios >1 indicate past applications of Aldrin. As Aldrin was not detected in any of the fish products, the ratios were not established. The concentrations of Dieldrin detected in the current study are in the same range as the concentrations that were detected in fresh fish species of L. niloticus and O. niloticus; whereby Dieldrin was up to 1.06 µg/kg, whereas the concentration of Aldrin was 0.34 µg/kg (Wenaty et al., 2019). However, the concentrations of Dieldrin in the fish products were lower than the maximum residue limit (MRL) of 200 µg/kg recommended by the competent authorities for fish and other fishery products (Biogo et al., 2010).
Of the four isomeric DDTs analysed, only p, pꞌ- DDE was found in all six fish products at variable concentrations as presented in Table 1. Deep fried products of Nile tilapia had the highest concentrations (3.73 µg/kg), while smoked Nile perch products had the lowest concentrations. The two DDT isomers; p, pꞌ- DDT and o, p- DDT were not detected at all. This is an indication of past use of DDT in the study area. Similarly, the other decomposition product p, pꞌ- DDD was not detected. This observation implies that degradation of DDT in fish is an aerobic process that produces p, pꞌ- DDE and not p, pꞌ- DDD due to availability of oxygen (Kasozi et al., 2006). The concentrations of DDTs measured in the current study are far lower than the concentrations of these chemicals in fresh fish muscles which were detected by Henry and Kishimba (2006) in the same study area. Henry and Kishimba reported the levels of DDTs in fish species from Southern Lake Victoria up to 300 µg/kg, and the main source was the continued use of DDTs in agriculture and vector control programmes. The ratio of DDT to DDE+DDE is commonly used to establish whether there is current or past application of DDT (Liu et al., 2010; Kuranchie-Mensah et al., 2011; Bergkvist et al., 2012; Afful et al., 2013). In our study, the parent compound DDT was not detected in any fish products, which indicates historical use of DDT, and therefore, the ratios were not established. The concentrations of DDT metabolites were far below the MRL of 200 µg/kg recommended for fish and other fishery products (Biogo et al., 2010).
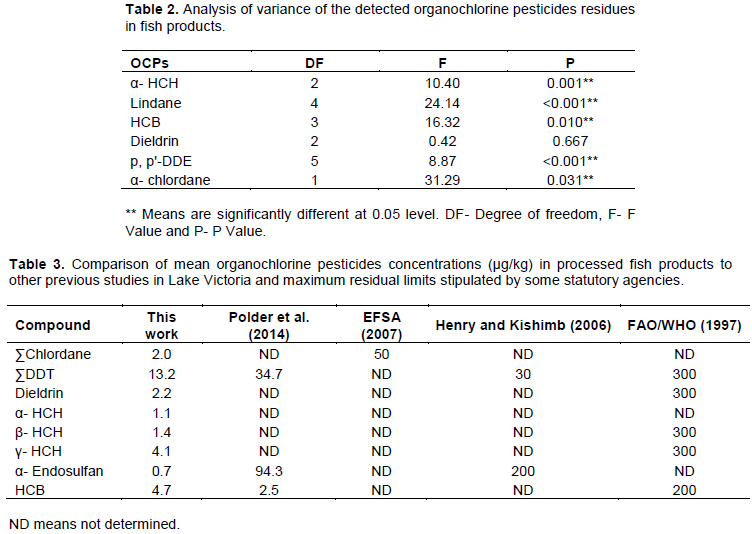
We analysed for three isomers of endosulfan; α- endosulfan, β- endosulfan and the degradation product; endosulfan sulfate, but only α- endosulfan was detected in one out of six fish products as described in Table 1. High α- endosulfan levels were detected in smoked Nile perch products (0.65 µg/kg) while other products had no detectable (<LOD) quantities of α- endosulfan. The presence of α- endosulfan in fish products indicates recent exposure of the fish products to the chemical through the food chain. However, β- endosulfan was not detected; this observation is probably due to variation of the two components in the technical endosulfan mixture that consists of 70% α- endosulfan and 30% β- endosulfan, with endosulfan sulfate being the decomposition product (Afful et al., 2013). Thus, in processed fish products, high concentrations of α- endosulfan than β- endosulfan could be anticipated.
Oxychlordane were detected at a mean concentration of 0.39 µg/kg in only one fish product; smoked Nile perch product as depicted in Table 1. All other fish products which were considered for this study had levels of Oxychlordane below their limits of detection (<LOD). Presence of Oxychlordane residues in fish products is due to environmental persistence.
Two fish products that were considered in this study; smoked products of Nile perch (0.89 µg/kg) and Nile tilapia (0.68 µg/kg) had trace amounts of α- chlordane and Oxychlordane as described in Table 1. However, the detected Oxychlordane and α- chlordane were below the Codex Alimentarius Commission extraneous residual limits (EMRL) of 0.05 mg/kg set for products of animal origin as sum of cis- and trans- isomers and Oxychlordane and expressed as chlordane (EFSA, 2007).
Heat treatments such as deep frying seems to significantly reduce the levels of persistent organochlorine pesticides in fish, as this method of frying accelerates drying of the fillets and evaporation of water and persistent organochlorine compounds due to the high temperature of the cooking oil, and by transfer of organochlorine compounds to the cooking oil which itself could be acting as an extraction solvent (Witczak, 2009). The deep fried fish products analysed contained high levels of organochlorine pesticides such as HCB, Dieldrin, p, pꞌ-DDE and Lindane as compared to other products like trims, salted-sundried and smoked products probably due to environmental contamination that occurs along the food chain as seen in Table 1. Smoking of fish tends to increase the levels of persistent organochlorine compounds due to a decrease in co-distillation with steam and the penetration of the compounds with smoke to the fish meat (Witczak and Tomza- Marciniak, 2010; Witczak, 2012). Our findings support this as most of the smoked samples had significant quantities of different persistent organochlorine pesticides.
Analysis of variance of the detected organochlorine pesticides in fish products collected from the market are given in Table 2. Mean separations using Duncan's Multiple Range Test indicate significant differences (p<0.05) of some OCP levels between fish products. For Oxychlordane, β- HCH and α- endosulfan, the means were not separated as they were detected in measurable levels in only one fish product each.
Evaluation of organochlorine residue levels
Table 3 compares mean organochlorine concentrations of the measured persistent organochlorine pesticides to MRLs recommended by some international statutory bodies for aquatic biota (Afful et al., 2013) and the OCP maximum residue concentrations found in fish meat in previous studies in Lake Victoria. Mean residue OCP levels were far lower (Henry and Kishimba, 2006) or in the same range (Polder et al., 2014) as compared with previous studies. The levels were also far below the MRL recommended by the European Food Safety Authority and Food and Agricultural Organization (FAO) of the United Nations as described in Table 3 for fish and other fishery products. Thus, persistent organochlorine pesticides do not seem to pose food safety health hazards to humans.
This study reports the human health risks associated with organochlorine pesticides in fish products from Lake Victoria that are sold in the domestic and regional markets. The results show that some organochlorine pesticides are present in fish products because of contamination in the value chain. The levels of the identified organochlorine pesticides were far lower than the previously reported concentrations in the study area and the MRLs recommended for fish and other fishery products. The cancer and non-cancer risks were ranked low and insignificant respectively. The fish products are therefore safe for human consumption and the organochlorine pesticides residue levels in the studied fish products do not represent a health risk to human beings. However, continuous monitoring is required in order to minimize the building up of the studied organochlorine compounds in the aquatic biota since they have the ability of increasing in concentration when they enter organisms at higher trophic niche such as human beings.
The authors have not declared any conflict of interests.
The authors acknowledge the Danish International Development Agency (DANIDA) through the Innovations and Markets for Lake Victoria Fisheries (IMLAF) project (DFC File No. 14 – P01 – TAN) for financial support to undertake this study. We are also grateful to the National Fish Quality Control Laboratory (NFQCL) staff in Mwanza for technical guidance during samples collection and extraction.
REFERENCES
Afful S, Awudza JAM, Osae S, Twumasi SK (2013). Persistent Organochlorine Compounds in the Water and Sediment Samples from Lake Bosomtwe in Ghana. American Chemical Science Journal 3(4):434-448.
Crossref
|
|
Anastassiades M, Lehotay SJ, Stajnbaher D, Schenck FJ (2003). Fast and Easy Multiresidue Method Employing Acetonitrile Extraction/Partitioning and "Dispersive Solid-Phase Extraction" for the Determination of Pesticide Residues in Produce. Journal of Association of Official Analytical Chemistry International 86:412-431.
|
|
|
ATSDR (1995). Public Health Assessment. Health and Human Services, Atlanta, New York 357p.
|
|
|
Baniga Z, Dalsgaard A, Mhongole OJ, Madsen H, Mdegela RH (2017). Microbial quality and safety of Rastrineobola argentea from Lake Victoria, Tanzania. Food Control 81:16-22.
Crossref
|
|
|
Baniga Z, Dalsgaard A, Kusiluka LJM, Mdegela R (2019). Microbiological quality of Nile perch (Lates niloticus) and physico- chemical properties of salted sun-dried products sold at regional markets, Tanzania. African Journal of Microbiology Research 13(7):128-133.
Crossref
|
|
|
Bergkvist C, Aune M, Nilsson I, Sandanger TM, Hamadani JD, Tofail F, Oyvind- Odland J, Kabir I, Vahter M (2012). Occurrence and levels of organochlorine compounds in human breast milk in Bangladesh. Chemosphere 88:784-790.
Crossref
|
|
|
Biego GHM, Yao KD, Ezoua P, Kouadio LP (2010). Assessment of organochlorine pesticides residues in fish sold in Abidjan markets and fishing sites. African Journal of Food, Agriculture, Nutrition and Development 10(3):2305-2323.
Crossref
|
|
|
Darko G, Akoto A, Lowor S, Yeboah P (2008). Persistent organochlorine pesticide in Fish, Sediments and Water from Lake Bosomtwi, Ghana. Chemosphere 72(1):21-24.
Crossref
|
|
|
EFSA (2007). European Food Safety Authority, Chlordane as undesirable substance in animal feed, Scientific Panel on Contaminants in the Food Chain. The European Food Safety Authority Journal 582:1-53.
Crossref
|
|
|
El-Mekkawi H, Diab M, Zaki M, Hassan M (2009). Determination of chlorinated organic pesticide residues in water, sediments and fish from private fish farms at Abbassa and Sahl- Al-Husainia, Sharkia Governorate. Australian Journal of Basic and Applied Sciences 3(4):4376-4383.
|
|
|
Farshid K, Amir HS, Rakhsareh M, Hamid NA (2012). Determination of organochlorine Pesticide Residues in Water, sediments and Fish from Lake Parishan, Iran. Journal of Fish and Marine Sciences 4(2):150:154.
|
|
|
FAO/WHO (1997). Codex maximum residual limits for pesticides, FAO/WHO, Rome Italy.
|
|
|
Ge J, Woodward LA, Li QX, Wang J (2013). Composition, distribution and risk assessment of organochlorine pesticides in soils from the Midway Atoll, North Pacific Ocean. Science of the Total Environment 452:421-426.
Crossref
|
|
|
Guzzella L, Roscioli C, Vigano L, Saha M, Sarkar SK, Bhattacharya A (2005). Evaluation of the concentration of HCH, DDT, HCB, PCB and PAH in the sediments along the lower stretch of Hugli Estuary West Bengal, Northeast India. Environmental International 31(4):523-534.
Crossref
|
|
|
Henry L, Kishimba MA (2006). Pesticide residues in Nile tilapia (Oreochromis niloticus) and Nile perch (Lates niloticus) from Southern Lake Victoria, Tanzania. Environmental Pollution 140(2):348-354.
Crossref
|
|
|
Kasozi GN, Kiremire BT, Bugenyi FWB, Kirsch NH, Nkedi - Kizza P (2006). Organochlorine Residues in Fish and Water Samples from Lake Victoria, Uganda. Journal of Environmental Quality 35:584-589.
Crossref
|
|
|
Kirema-Mukasa CT (2013). Regional Fish Trade in Eastern and Southern Africa- Products and Markets. A Fish Trader's Guide Prepared No. 013. Regional Fish Trade in Eastern and Southern Africa, Ebene, Mauritius 54p.
|
|
|
Kuranchie-Mensah H, Naa-Dedei PLM, Atiemo-Manukure N, Afful S, Adjei-Martey G (2011). Assessment of organochlorine pesticides and polychlorinated biphenyls levels in fishes from the Volta Lake, Ghana and their suitability for human consumption. Elixir Food Science 41:5982-5990.
|
|
|
Lake Victoria Fisheries Organization(LVFO) (2013). Processing and Marketing of Small- Sized Pelagics in Eastern and Southern Africa. Working Paper No 29. Published by the European Union. Jinja, Uganda 42p.
|
|
|
Liu Z, Zhang H, Tao M, Yang S, Wang L, Liu Y, Ma D, He Z (2010). OCPs in consumer fish and mollusks of Liaoning province, China: distribution and human exposure implications. Archives of Environmental Contamination and Toxicology 59:444-453.
Crossref
|
|
|
Madadi OV, Wandiga SO, Jumba IO (2006). The status of persistent organic pollutants in Lake Victoria catchment. In: Proceedings of the 11th World Lakes Conference. (Edited by Odada, E. O. and Daniel, O.), Kampala, Uganda, pp. 107-112.
|
|
|
Mahugija JAM, Nambela L, Mmochi AJ (2018). Determination of Dichlorodiphenyltrichloroethane (DDT) and Metabolites Residues in Fish Species from Eastern Lake Tanganyika. South African Journal Chemistry 71:86-93.
Crossref
|
|
|
Manirakiza P, Covaci A, Nizigiymana L, Ntakimazi G, Schepens P (2002). Persistent chlorinated pesticides and polychlorinated biphenyls in selected fish species from Lake Tanganyika, Burundi, Africa. Environmental Pollution 117(3):447-455.
Crossref
|
|
|
Oluoch-Otiego J, Oyoo-Okoti E, Kiptoo KKG, Chemoiwa EJ, Ngugi CC, Simiyu G, Omutange ES, Ngure V, Opiyo MA (2016). PCBs in fish and their cestode parasites in Lake Victoria. Environmental Monitoring and Assessment 188(483):1-14.
Crossref
|
|
|
Orris PL, Kaatz CK, Asbury J (2000). Persistent Organic Pollutants and Human Health. World Federation of Public Health Associations, Washington DC. 45 p.
|
|
|
Polder A, Muller MB, Lyche JL, Mdegela RH, Nonga HE, Mabiki FP, Mbise TJ, Skaare JU, Sandvik M, Skjerve E, Lie E (2014). Levels and patterns of persistent organic pollutants (POPs) in tilapia (Oreochromis sp) from four different lakes in Tanzania: Geographical differences and implications for human health. Science of the Total Environment 488(489):252-260.
Crossref
|
|
|
Ssebugere P, Sillanpa M, Wang P, Li Y, Kiremire BT, Kasozi GN, Zhu C, Ren D, Zhu N, Zhang H, Shang H, Zhang Q, Jiang G (2014a). Polychlorinated biphenyls in sediments and fish species from the Murchison Bay of Lake Victoria, Uganda. Science of the Total Environment 482(483):349-357.
Crossref
|
|
|
Ssebugere P, Sillanpa M, Kiremire BT, Kasozi GN, Wang P, Sojinu SO, Otieno PO, Zhu N, Zhu C, Zhang H, Shang H, Ren D, Li Y, Zhang Q, Jiang G (2014b). Polychlorinated biphenyls and hexachlorocyclohexanes in sediments and fish species from the Napoleon Gulf of Lake Victoria, Uganda. Science of the Total Environment 481:55-60.
Crossref
|
|
|
U.S. Environmental Protection Agency (USEPA) (1997). Exposure Factors Handbook General Factors. Environmental Protection Agency, Washington DC. 854 p.
|
|
|
U.S. Environmental Protection Agency (USEPA) (2001). Supplemental Guidance for Developing Soil Screening Levels for Superfund Sites. Environmental Protection Agency, Washington DC. 187pp.
|
|
|
U.S. Environmental Protection Agency (USEPA) (2009). Risk Assessment Guidance for Superfund. Human Health Evaluation Manual. Environmental Protection Agency, Washington DC. 291 p.
|
|
|
Wang L, Jia HL, Liu XJ, Sun YQ, Yang M, Hong WJ (2013). Historical contamination and ecological risk of organochlorine pesticides in sediment core in North Eastern Chinese River. Ecotoxicology and Environmental Safety 93:112-120.
Crossref
|
|
|
Wenaty A, Fromberg A, Mabiki F, Chove B, Dalsgaard A, Mdegela R (2019). Persistent Organochlorine Compounds Levels in Selected Fish Species from Lake Victoria and Associated Risks to Human Health. Agriculture, Forestry and Fisheries 8(1):1-9.
|
|
|
Witczak A, Tomza- Marciniak A (2010). The health risk assessment of organochlorine pesticides in smoked fish products available in Szczecin, Poland. Journal of Environmental Science and Health, Part B 45:658-665.
Crossref
|
|
|
Witczak A (2012). Influence of Smoking on Indicatory PCB Congeners Residues Levels in Fish Slices. Polish Journal of Food and Nutrition Sciences 62(1):31-39.
Crossref
|
|
|
Witczak A (2009). Effect of Heat Treatment on Organochlorine Pesticides in Selected Fish Species. Polish Journal of Food and Nutrition Sciences 59(3):231-235.
|
|
|
Yi Z, Guo P, Zheng L, Huang X, Bi J (2013). Distribution of HCHs and DDTs in the soil-plant system in tea gardens in Fujian, a major tea-producing province in China. Agriculture, Ecosystem and Environment 171:19-24.
Crossref
|
|