ABSTRACT
The fungi are living organisms of great importance around agriculture, food industry, and the pharmaceutical industry. The usage of these microorganisms in agriculture is for the biocontrol of diseases in plants of commercial interest. Some yeasts can fight phytopathogens through secondary metabolites produced, inhibiting their development. Some species show positive results in the control of pathologies in different cultures. Yeasts have been used as biocontrol agents, and among them, a Lachancea (Kluyveromyces) thermotolerans, which is present in plants (such as grapes), soil and insects, can be adapted to different environments and, possibly perform biological control, although it is not known its mode of action. This work aimed to analyze and identify secondary metabolites used by the yeast L. thermotolerans CCMA 0763 isolated from commercial grapes in the Agricultural Microbiology Laboratory (Federal University of Lavras-MG/BR), using the analytical method of Ultra High-Efficiency Liquid Chromatography (UHPLC) (HRMS) and Tandem Mass Spectrometry (MS/MS). Four heterocyclic compounds of the Alkaloids class were identified, three (03) natural: 4-Hydroxyquinoline, Xanthine and Calistegine A3, and one (01) synthetic: Clausehainanine C. Therefore, these compounds can be tested against phytopathogenic microorganisms.
Key words: Yeast, biological control, secondary metabolite, ultra high-efficiency liquid chromatography (UHPLC), mass spectrometry.
Non-Saccharomyces yeasts are sources of complex aromatics, glycerol content and responsible in ethanol reduction and polysaccharide concentrations in industrial processes. Its main functionality is associated with the production of alcoholic beverages from fermentation processes (Contreras et al., 2014; Belda et al., 2015, 2017; Domizio et al., 2017). This fungus is mainly in the production of wine, on substrates containing hexoses, playing an important role in the characteristics of the drink not producing spores or allergenic toxins; and are not nutritionally demanding. They present rapid growth, they do not present risks to the consumer and they act as antagonists, either through competition for nutrients, antibiosis or hyperparasitism (Domizio et al., 2017; Mehlomakulu et al., 2014, 2015). However, its use is not limited to wines, beers, breads and cheeses productions, but on other products for example control of phytopathogens. The good performance of yeasts as biocontrol agents is due to the different modes of action of these microorganisms against phytopathogens. Among these, we can highlight the competition with phytopathogens for space and nutrients, mycoparasitism, antibiosis, resistance induction in plants and the production of antimicrobial toxins and alcohol by yeasts. Understanding these biocontrol mechanisms is essential to improve the action of these microorganisms in combating plant diseases (Hatoum et al., 2012; Muccilli and Restuccia, 2015). Biological control is an important management strategy for fungal diseases, with the basic principle of using antagonistic microorganisms to reduce and/or keep the population of a phytopathogen below levels that cause economic loss (Carmona-Hernandez et al., 2019). Organic control using biological agents against mycotoxins is considered a safer option until now, gaining popularity in the food industry (Tsitsigiannis et al., 2012). In fact, the application of yeasts (cells and their volatiles) and yeast derivatives has great potential to minimize the economic losses caused by mycotoxigenic fungi. Several of yeast and bacteria species have been identified as having effective fungi biological control activities at pre- and pos-harvest, where they were tested against toxigenic fungi to inhibit their growth and mycotoxin synthesis (Catara, 2007; Haiissam, 2011). Within the microorganisms tested, the non-saccharomyces yeasts are promising antimicrobial and bio-remediation agents (Ùbeda et al., 2014). However, the antimicrobial properties associated with yeast are linked to the production of killer toxins. Killer toxins have been identified as glycosylated proteins that bind to specific receptors. Their strains were called killer yeast. Killer yeasts secrete lethal toxins to and their strains are insensitive to their own toxins (Alturki et al., 2019; Mannazzu et al., 2019).
In addition, the biological properties associated with this type of microorganism are not limited only to the production of proteins, but in the direct and/or indirect interactions between the different strains through the physical-transformed changes in the environment caused by the reaction of one strain to another. The effect of a specific yeast strain on its environments is unique suited to its metabolites and their respective (Roullier-Gall et al., 2020). Thus, it is evident that non-saccharomyces yeasts have a favorable metabolic engineering for the production of new and well-known compounds with wide biological activity (Kulkarni et al., 2015; Liu et al., 2020). Currently, thousands of secondary metabolites have been identified from various living organisms, mostly plants. However, it is estimated that this number is higher, being, in part, limited to analytical techniques. With the instrumental advance, compounds with a high degree of difficulty in analysis, whether due to their abundance, structural diversity or the complexity of the matrix, have been constantly identified (Zhao et al., 2013). Among the technological tools used to identify these molecules, is Ultra High Efficiency Liquid Chromatography (UHPLC) (HRMS), to analyze the yeast metabolome, for its excellent resolution, mass accuracy, new possibilities for improving analytical methods for matrices complex samples (ingredients, food and biological samples) and a wide range of spectrometric data (Motilva et al., 2013). Thus, the present work aimed to partially characterize the secondary metabolites produced by the yeast Lachancea thermotolerans CCMA 0763, isolated from commercial raisin, using the analytical technique of (UHPLC)(HRMS) and Tandem Mass Spectrometry (MS/MS).
Crude extract obtainment
The yeast L. thermotolerans CCMA 0763 (Sample) belonging to the Agricultural Microbiology Culture Collection at the Federal University of Lavras - Minas Gerais/Brazil was grown in a Petri dish containing Potato-Dextrose-Agar (PDA) (Culture Medium) for 2 days and inoculated in 500 ml Erlenmeyers containing Potato-Dextrose (PD) with pH 6.8 and incubated without shaking for 21 days at 28°C (Quality Control). The mycelium was separated from the fermented broth by filtration in cotton and hydrophobic gauze and the aqueous phase was centrifuged at 2750 rpm for 15 min. The liquid-liquid extraction was performed with Ethyl Acetate in the proportion of 1:3 (Ethyl acetate: fermented broth) in a separating funnel. This step was repeated three times. The solvent was collected and evaporated in a Rotary Evaporator (Tecnal TE-210) at 400°C and the Crude Extract (1.014 g) subjected to Ultra High Efficiency Liquid Chromatography Analysis (UHPLC) (HRMS) and Tandem Mass Spectrometry (MS/MS). The same procedure was used for the Quality Control sample.
Chemical analyses
The solvents used were: Acetonitrile grade UHPLC-MS (JT Baker-Phillipsburg, NJ, USA), Deionized Water from a Millipore Milli-Q water reagent system (Millipore, Bedford, MA, USA) and Analytical grade Formic Acid (85%). The extract of the yeast L. thermotolerans (1.0141 g) was solubilized in methanol and the concentration of the solution corrected to 1.0 mg. mL-1. For the Mass Spectrometry Analysis, a volume of 1.0 ml of Yeast Extract was collected and 10.0 μl of 0.1% Formic Acid (v/v) (Analysis in positive mode) was added and stored in a vial for analysis.
Liquid chromatography
The extracts (Sample and Control) were analyzed by Ultra-High Performance Liquid Chromatography (Shimadzu, Nexera X2, Japan) coupled to a High Resolution Mass Spectrometer (Impact II, Bruker Daltonics Corporation, Germany) equipped with an ionization source by eletrospray. The chromatographic separation was performed with an Acquity UPLC® CSHTM C18 column, particle size of 2.1 μm, with 2.1 × 100 mm (Waters, Ireland) and flow rate of 0.200 ml min-1. The gradient of the solvent mixture A (H2O with 0.1% (v/v) formic acid) and B (acetonitrile with 0.1% (v/v) formic acid) as follows: 5% B 0-1 min, 50% B 1- 5 min, 95% B 5-10 min and maintained at 95% B 10-16 min, 5% B 16-18 min and maintained at 5% B 18-32 min at 40°C.
Mass spectrometry
The ionization source was operated in positive ionization modes and set to 4500V with a potential displacement of the final plate of 500V. The drying gas parameters were adjusted to 8 L min-1 at 180°C and the nebulizer gas pressure was set to 4 bar. The data were collected from m/z 50 to 1300 with an acquisition rate of 5 Hz and the 4 most intense ions were selected for automatic fragmentation (Auto MS/MS).
Data processing and statistical analyses
The software used for data processing for peak detection, multivariate analysis and identification was Data Analysis 4.0 (Bruker, Germany). The specialized databases were: MoNa (http://mona.fiehnlab.ucdavis.edu/), ChemSpider (http://www.chemspider.com/) and Metlin (https: //metlin.scripps. Edu) for the identification of compounds. The search parameters in the online databases were: precursor mass with error ≤ 10 ppm and fragment tolerance ≤ 10 ppm. Principal component analysis (PCA) and projection discrimination in an orthogonal latent structure (OPLC-DA) were generated using the ProfileAnalysis 2.1 software.
Figure 1 represents the diversity of the chemical profiles of L. thermotolerans and Quality Control extracts in the positive mode chromatogram generated from the UHPLC-HRMS. The chromatographic run had an injection time of 28.0 min with 4.0 min for column balance and cleanliness. It was revealed in this first analysis that in the time between 1.0 to 6.0 min, the two chromatograms behave differently in relation to the intensity and areas of the peak. This is also seen in the time between 14.0 and 25.5 min. However, the chromatographic profile is very similar between the Control and the Sample, little difference between them. However, UHPLC-HRMS analyzes provide coverage of the chemical space based on MS and MS/MS data and are capable of presenting the best differentiation between Sample and Control. For this experiment, the molecular masses studied were 50 to 1300 Dalton (Da). This mass distribution of precursors was based on studies in the literature (Maciá-Vicente et al., 2018).
The global chemical space observed in the complete data set comprised eight thousand exclusive MS/MS spectra after the first performed for a sample and control. To minimize and discard potential noise or artifacts present in the UHPLC-HRMS analyzes, three boundary conditions were imposed: first, the removal of the spectra present only in the control or in the whites; second, consider the final spectra that occurred only in the sample; third, consider spectra with acquired MS/MS, an important identification of the compounds in the sample. All spectral data were collected and processed using centralization, isotopic pattern, filtering, retention time and peak recognition methods to generate a datamatrix including sample identity, ion identity and ion abundance. The result of this mathematical deconvolution procedure provided 126 exclusive ions from the sample. The software used for data processing for peak detection, multivariate analysis and identification was DataAnalysis 4.0 (Bruker, Germany) and Software ProfileAnalysis 2.1. For the identification of compounds, specialized Database sites were used, such as: MoNa, ChemSpider and Metlin. Other parameters, such as fragmentation score and bibliographic record on the occurrence of the molecules, were also considered for disambiguation. The main exclusion factors used for identification were: mass error less than or greater than 10 ppm to decrease the number of candidates, fragmentation profile to justify and prove the identification and the probability of ionization by ESI.
This attention with several exclusion factors is associated with the possibility of compounds within a mass range of 0.5 to 5 ppm, which generally have very different numbers of carbon atoms and the biosynthesis of units with a common elementary composition that provides information limited. Thus, mass accuracy and isotopic ratio, are almost orthogonal parameters that can be used to exclude hypothetical elementary compositions, but do not establish a consistent identification (Nielsen et al., 2011). In these cases, MS/MS, or preferably by Sequential Mass Spectrometry (MSn), with subsequent fragmentation patterns is more efficient, assuming that reference standards are available to model the fragmentation of the class of compounds (Sharma et al., 2007). Thus, all precursors were submitted to the parameters presented here to support their identification. Four compounds were identified (Table 1), with their ESI-MS/MS spectra, respectively (Figures 2 to 5).
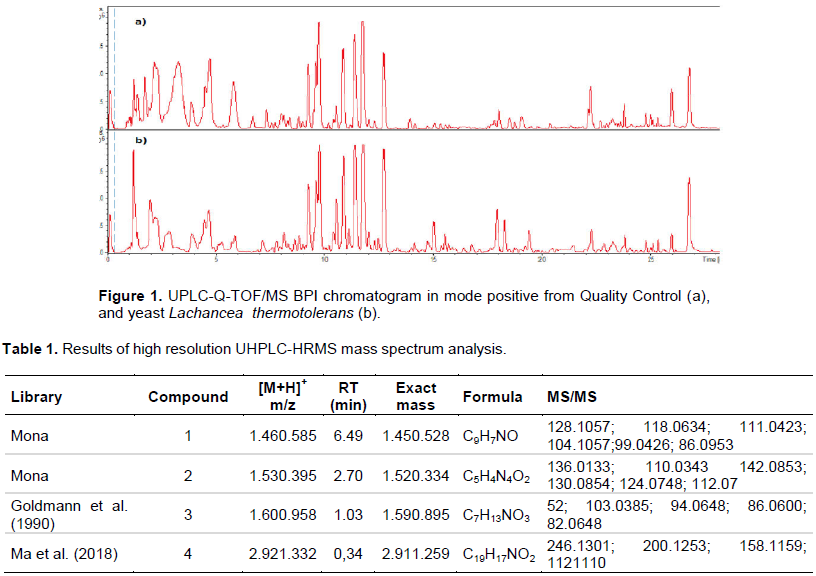
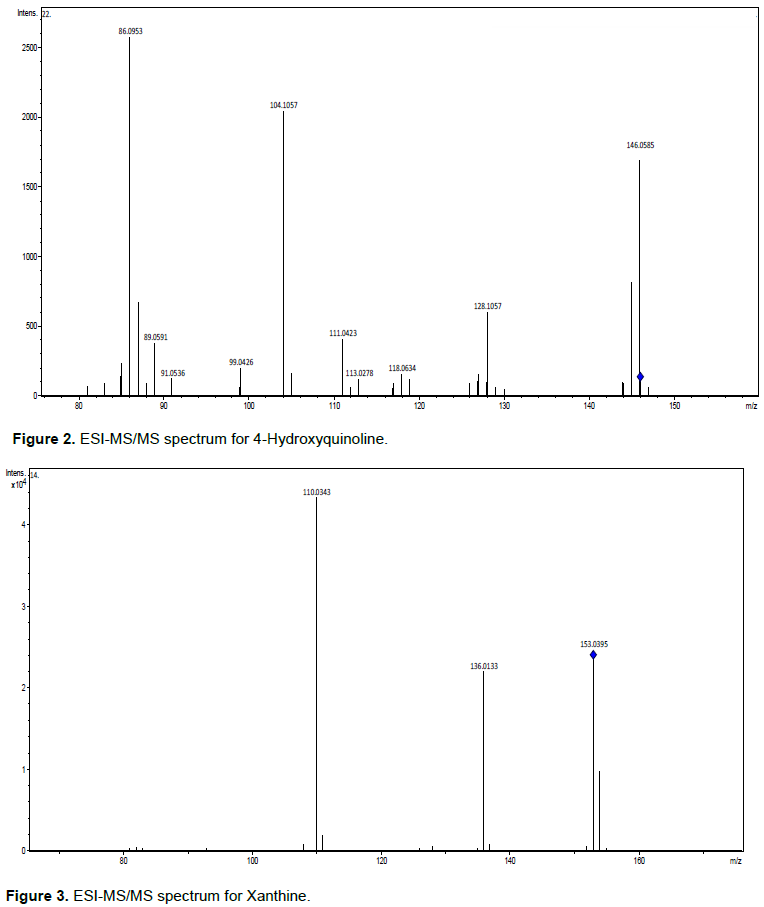
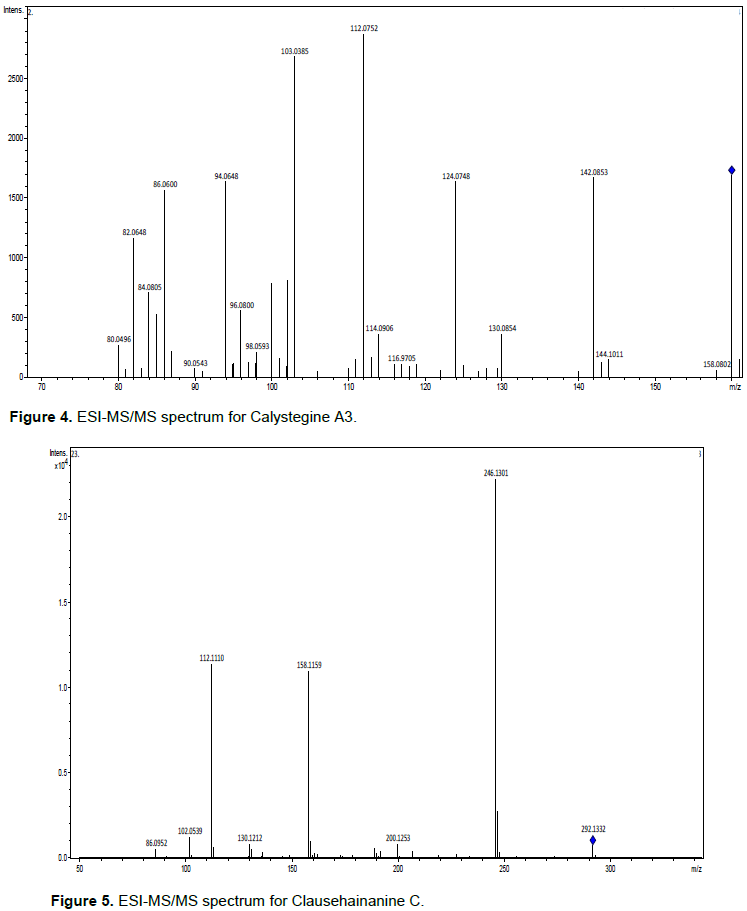
All compounds identified are alkaloids (Figure 6). They have nitrogen atoms and a differentiated fragmentation profile in their chemical skeleton. The compound identified as 4-Hydroxyquinoline belongs to the group of nitrogenous heterocyclic compounds of the quinolone alkaloids class. They play an important role in several areas of knowledge and have a wide range of natural substances. Quinolones are molecules structurally derived from the heterocyclic Quinoline, being distributed in nature as a product of the secondary metabolism of several species of plants and fungi, mainly in species of the family Rutaceae (Suárez et al., 2011). Several derivatives of Quinolone have been isolated from this family with analgesic, amebicidal, antiviral, herbicidal and fungicidal activities (Barrera and Suarez, 2007). The compound 8-Hydroxyquinoline and its derivatives are a subclass of Quinolines with a wide variety of biological activities. They have been used as a fungicide in agriculture and a preservative in the textile, wood and stationery industries (Oliveri and Vecchio, 2016).
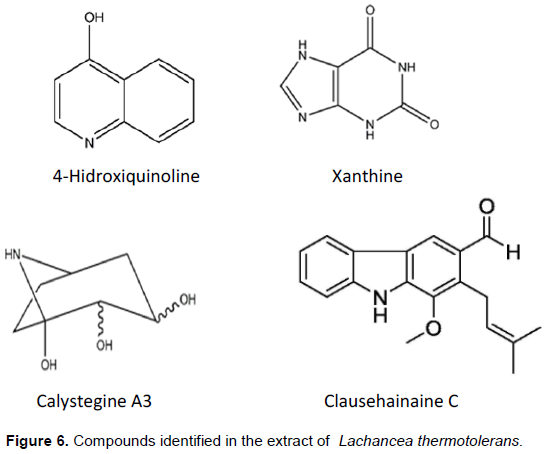
Xanthines are a class of Purine Alkaloids found in many different plants, including yerba mate (1,3- and 3,7- Dimethylxanthine), coffee (1,3,7-Trimethylxanthine) and cocoa (Athayde et al., 2000). Another particularity associated with these compounds is their biological function in conjunction with the enzyme Xanthine A dehydrogenase (XDH), a Hydroxylase containing Molybdenum, being important for regulating the metabolism of Purines and Uric Acid responsible for the regulation of Arabidopsis aging and resistance to stress, peas, corn and grapes (Barabás et al., 2000; Zdunek-Zastocka and Lips, 2003; Brychkova et al., 2008; Werner and Witte, 2011; Shuanghong et al., 2017; Han et al., 2020).
Calistegines are a group of alkaloids (glycoalkaloids) initially discovered in the roots of cultures of Calystegia sepium and Atropa belladonna. The chemical structures contain a Nortropane ring system with 3-, 4-, or 5-Hydroxyl groups (Calistegines A, B or C, respectively), located in various positions with different stereochemistry (Aminocetal functionality), and an Amino bridge group (Asano et al., 2000). These compounds were identified in several genera such as Atropa, Datura, Duboisia, Hyoscyamus and Escopolia belonging to the Solanaceae, Convolvulaceae, Erythroxylaceae and Brassicaceae families (Schimming et al., 2005; Brock et al., 2005, 2006; Torres-Romera et al., 2019). The biological activities associated with polyhydroxylated alkaloids are their levels of toxicity to bacteria, fungi, viruses, insects, animals and humans (Friedman, 2006).
Carbazolic Alkaloids are characterized by a basic aromatic tricyclic skeleton consisting of a central Pyrrole ring fused with two Benzene rings. Carbazol itself was originally isolated from the Anthracene fraction of coal tar (Greger, 2017). They have been found in bacteria, myxomycetes, fungi, sponges, tunicates and in the related plant families Apocynaceae and Loganiaceae. The vast majority of Carbazoles, comprising more than 330 derivatives, have been shown to be derived from 3-Methylcarbazole as a common precursor (Ryvolova et al., 2012). The vast majority of Carbazoles, comprising more than 330 derivatives, have been shown to be derived from 3-Methylcarbazole as a common precursor. This type of Carbazoles was called Fitocarbazoles (Chakraborty and Roy 2003). However, there is no scientific evidence from recent studies related to the application of this compound in the area of agriculture, so it can be the object of research to be investigated.
The crude extract of secondary metabolites from the yeast L. thermotolerans presents eight thousand exclusive MS/MS spectra through the UHPLC-HRMS analysis. These data were included in the mathematical deconvolution procedure, obtaining 126 exclusive ions from the sample. The analytical method of UHPLC-HRMS has been a high performance tool for the identification of compounds and biomolecules of great structural diversity for the analysis of secondary metabolites. Thus, four components of the class of heterocyclic alkaloids were identified, three (03) naturals: 4-Hydroxyquinoline, Xanthina and Calistegina A3; and one (01) synthetic: Clausehainanina C from the crude extract of L. thermotolerans. These compounds present, through the literature: antimicrobial, antiproliferative, inhibitory and regulatory activities, applied in the most diverse areas of knowledge, being able to be tested as biocontrol agents in antagonistic tests of biological tests against phytopathogens that cause fungal and bacterial diseases in agricultural cultures.
This study was partially funded by the Coordination for the Improvement of Higher Education Personnel - Brazil (CAPES) - Financial Code 001.
The authors have not declared any conflict of interests.
REFERENCES
Alturki SN, Al-Saud NS, Alhenjin AM, Hassan SM (2019). Study of Yeast Killer Toxins and Their Interactions. International Journal Advanced of Research 7(5):314-320.
Crossref
|
|
Asano N, Nash RJ, Molyneux J, Fleet GWJ (2000). Sugar-mimic glycosidase inhibitors?: natural occurrence, biological activity and prospects for therapeutic application. Tetrahedron: Asymmetry 11(8):1645-1680.
Crossref
|
|
|
Athayde ML, Coelho GC, Schenkel EP (2000). Caffeine and theobromine in epicuticular wax of Ilex paraguariensis A. St.-Hil," Phytochemistry 55(7):853-857.
Crossref
|
|
|
Barabás NK, Omarov RT, Erdei L, Lips SH (2000). Distribution of the Mo-enzymes aldehyde oxidase, xanthine dehydrogenase and nitrate reductase in maize (Zea mays L.) nodal roots as affected by nitrogen and salinity. Plant Science 155(1):49-58.
Crossref
|
|
|
Barrera CAC, Suarez LEC (2007). Metabolitos secundarios aislados de los géneros Raputia y Esenbeckia (Rutaceae). Scientia et Technica 1(33):337-338.
|
|
|
Belda I, Navascués, E, Marquina D, Santos A, Calderon F, Benito S (2015). Dynamic analysis of physiological properties of Torulaspora delbrueckii in wine fermentations and its incidence on wine quality. Applied Microbiology and Biotechnology 99(4):1911-1922.
Crossref
|
|
|
Belda I, Ruiza J, Beata Beisert B, Navascuésc E, Marquina D, Calderón F, Rauhut D, Benito S, Santos A (2017). Influence of Torulaspora delbrueckii in varietal thiol (3-SH and 4-MSP) release in wine sequential fermentations. International Journal of Food Microbiology 257:183-191.
Crossref
|
|
|
Brock A, Bieri S, Christen P, Dra B (2005). Calystegines in wild and cultivated Erythroxylum species. Phytochemistry 66(11):1231-1240.
Crossref
|
|
|
Brock A, Herzfeld T, Paschke R, Koch M, Dräger B (2006). Brassicaceae contain nortropane alkaloids. Phytochemistry 67(18):2050-2057.
Crossref
|
|
|
Brychkova G, Alikulov Z, Fluhr R, Sagi M (2008). A critical role for ureides in dark and senescence?induced purine remobilization is unmasked in the Atxdh1 Arabidopsis mutant. The Plant Journal 54(3):496-509.
Crossref
|
|
|
Catara V (2007). Pseudomonas corrugata?: plant pathogen and/or biological resource?? Molecular Plant Pathology 8(3):233-244.
Crossref
|
|
|
Carmona-Hernandez S, Reyes-Péres JJ, Chiquito-Contreras RG, Rincon-Enriquez G, Cerdan Cabrera CR, Hernandez-Montiel LG (2019). Biocontrol of Postharvest Fruit Fungal Diseases by Bacterial Antagonists?: A Review. Agronomy 9(3):121.
Crossref
|
|
|
Chakraborty DP, Roy S (2003). Carbazole Alkaloids IV. In: Fortschritte der Chemie organischer Naturstoffe / Progress in the Chemistry of Organic Natural Products. Fortschritte der Chemie organischer Naturstoffe/Progress in the Chemistry of Organic Natural Products, vol 85. Springer, Vienna.
Crossref
|
|
|
Contreras A., Hidalgo C, Henschke PA, Chambers PJ, Curtin C, Varela C (2014). Evaluation of non-Saccharomyces yeasts for the reduction of alcohol content in wine. Applied and Environmental Microbiology 80(5):1670-1678.
Crossref
|
|
|
Domizio P, Liu Y, Bisson LF, Barile D (2017). Cell wall polysaccharides released during the alcoholic fermentation by Schizosaccharomyces pombe and S. japonicus: quantification and characterization. Food Microbiology 61:136-149.
Crossref
|
|
|
Friedman M (2006). Potato glycoalkaloids and metabolites: roles in the plant and in the diet. Journal of Agricultural and Food Chemistry 54(23):8655-8681.
Crossref
|
|
|
Greger H (2017). Phytocarbazoles: alkaloids with great structural diversity and pronounced biological activities. Phytochemistry Reviews 16:1095-1153.
Crossref
|
|
|
Haiissam JM (2011). Pichia anomala in biocontrol for apples: 20 years of fundamental research and practical applications Antonie Van Leeuwenhoek 99(1):93-105.
Crossref
|
|
|
Han R, He X, Pan X, Shi Q, Wu Z (2020). Enhancing xanthine dehydrogenase activity is an effective way to delay leaf senescence and increase rice yield. Rice 13(1):1-14.
Crossref
|
|
|
Hatoum R, Labrie S, Fliss I (2012). Antimicrobial and probiotic properties of yeasts?: from fundamental to novel applications. Frontiers in Microbiology 3:421.
Crossref
|
|
|
Kulkarni P, Loira I, Morata A, Tesfaye W, González MC, Suárez-Lepe JA (2015). Use of Non-Saccharomyces yeast strains coupled with ultrasound treatment as a novel technique to accelerate ageing on lees of red wines and its repercussion in sensorial parameters. Food Science and Technology 64(2):1255-1262.
Crossref
|
|
|
Liu S, Laaksonen O, Marsol-Vall A, Zhu B, Yang B (2020). Comparison of Volatile Composition between Alcoholic Bilberry Beverages Fermented with Non- Saccharomyces Yeasts and Dynamic Changes in Volatile Compounds during Fermentation. Journal of Agricultural and Food Chemistry 68(11):3626-3637.
Crossref
|
|
|
Maciá-Vicente JG, Shi YN, Cheikh-Ali Z, Grün P, Glynou K, Haghi Kia S, Piepenbring M, Helge B, Bode HB (2018). Metabolomics-based chemotaxonomy of root endophytic fungi for natural products discovery. Environmental Microbiology 20(3):1253-1270.
Crossref
|
|
|
Mannazzu I, Domizio P, Carboni G, Zara S, Zara G, Comitini F, Budroni M, Ciani M (2019). Yeast killer toxins: from ecological significance to application. Critical Reviews in Biotechnology 39(5):603-617.
Crossref
|
|
|
Mehlomakulu NN, Setati ME, Divol B (2015). Non-saccharomyces killer toxins possible biocontrol agents against brettanomyces in wine. South African Journal of Enology and Viticulture 36(1):94-104.
Crossref
|
|
|
Mehlomakulu NN, Setati ME, Divol B (2014). Characterization of novel killer toxins secreted by wine-related non-Saccharomyces yeasts and their action on Brettanomyces spp. International Journal of Food Microbiology 188:83-91.
Crossref
|
|
|
Motilva MJ, Serra A, Macià A (2013). Analysis of food polyphenols by ultra high-performance liquid chromatography coupled to mass spectrometry?: An overview. Journal of Chromatography A 1292:66-82.
Crossref
|
|
|
Muccilli S, Restuccia C (2015) Bioprotective Role of Yeasts. Microorganisms 3(4):588-611.
Crossref
|
|
|
Nielsen KF, Ma M, Rank C, Frisvad JC, Larsen TO (2011). Dereplication of microbial natural products by LC-DAD-TOFMS. Journal of Natural Products 74(11):2338-2348.
Crossref
|
|
|
Oliveri V, Vecchio G (2016). 8-Hydroxyquinolines in medicinal chemistry: A structural perspective. Journal of Medicinal Chemistry 120:252-274.
Crossref
|
|
|
Roullier-Gall C, David V, Hemmler D, Schmitt-Kopplin P, Alexandre H (2020). Exploring yeast interactions through metabolic profiling. Scientific Reports 10(1):1-10.
Crossref
|
|
|
Ryvolova M, Adam V, Eckschlager T, Stiborova M, Kizek R (2012). Study of DNA-ellipticine interaction by capillary electrophoresis with laser-induced fluorescence detection. Electrophoresis 33(11):1545-1549.
Crossref
|
|
|
Shuanghong Y, Zhu Bo, Wang F, Han H, Sun M, Zhu H, Peng R, Yao Q (2017). A Vitis vinifera xanthine dehydrogenase gene, VvXDH, enhances salinity tolerance in transgenic Arabidopsis. Plant Biotechnology Reports 11(3):147-160.
Crossref
|
|
|
Schimming T, Jenett-Siems K, Mann P, Tofern-Reblin B, Milson J, Johnson RW, Deroin T, Daniel F, Austin DF, Eich E (2005). Calystegines as chemotaxonomic markers in the Convolvulaceae. Phytochemistry 66(4):469-480.
Crossref
|
|
|
Sharma A, Jansen R, Nimtz M, Johri BN, Wray V (2007). Rhamnolipids from the rhizosphere bacterium Pseudomonas sp. GRP3 that reduces damping-off disease in chilli and tomato nurseries. Journal of Natural Products 70(6):941-947.
Crossref
|
|
|
Suárez LEC, Barrera CAC, Barrera EDC, Moreno JML (2011). Antibacterial activity of terpenes and alkaloids isolated from three Colombian plants. Cuban Magazine of Pharmacy 45(2):275-282.
|
|
|
Torres-Romera A, Arrebola-Liebanas J, Vidal JLM, Frenich AG (2019). Determination of Calystegines in Several Tomato Varieties Based on GC-Q-Orbitrap Analysis and their Classi?cation by ANOVA. Journal of Agricultural and Food Chemistry 67(4):1284-1291.
Crossref
|
|
|
Tsitsigiannis DI, Dimakopoulos M, Antoniou PP, Tjamos EC (2012). Biological control strategies of mycotoxigenic fungi and associated mycotoxins in Mediterranean basin crops. Phytopathologia Mediterranea 51(1):158-174.
|
|
|
Ùbeda JF, Maldonado M, Briones AI, González FJ (2014). Bio-prospecting of Distillery Yeasts as Bio-control and Bio-remediation Agents. Current Microbiology 68(5):594-602.
Crossref
|
|
|
Zhao N, Wang G, Norris A, Chen X, Chen F (2013). Studying Plant Secondary Metabolism in The Age of Genomics. Critical Reviews in Plant Sciences 32(6):369-382.
Crossref
|
|
|
Zdunek-Zastocka E, Lips HS (2003). Is xanthine dehydrogenase involved in response of pea plants (Pisum sativum L .) to salinity or ammonium treatment?? Acta Physiologiae Plantarum 25(4):395-401.
Crossref
|
|
|
Werner AK, Witte CP (2011). The biochemistry of nitrogen mobilization: Purine ring catabolism. Trends in Plant Science 16(7):381-387.
Crossref
|
|