ABSTRACT
The sheath rot rice disease is known to be associated to Pseudomonas fuscovaginae and a recent study on the symptomatic of sheath rot revealed that this bacterial pathogen is abundant in highland rice ecology cultivation in Burundi. From asymptomatic samples of sheath rice, a collection of bacterial isolates and culturable microbiome have been carried out. A comparison between the culturable microbiome and total microbiome was made. Phenotypic assays in vitro on the bacterial isolates were performed after identification at the genus level of each bacterial isolate by sequencing of 16S RNA gene. A bacterial isolate belonging to Alcaligenes genus has antibacterial activity against the rice sheath rot pathogen P. fuscovaginae in vitro conditions.
Key words: Pseudomonas fuscovaginae, sheath rot, microbiome, Alcaligenes species.
Each part of the plant (e.g. root, stem, leaf, and fruit) is associated with a microbial community that altogether form the plant microbiome. Depending on the plant part, the microbial community can vary considerably consisting of only a few species or being very diverse. Microbiome studies have an important role to bring insight into the composition of these communities that form the plant microbiome. The rhizospheric microbiome is the community of microbes closely associated or attached to the roots whereas the endosphere microbiome is the microbes that live inside plants in intercellular spaces mostly originating from the rhizosphere (Edwards et al., 2015). The phyllosphere/epiphytic microbiome is located on the surface aerial parts and lastly, the seed microbiome is the vertically transferred microbes (Gopal and Gupta, 2016).
The phyllosphere part of plants represents the largest environmental surface habitat area of microbes on earth (Lindow and Brandl, 2003; Vorholt, 2012; Peñuelas and Terradas, 2014), and much of that surface area is due to the agriculture of crops (Foley et al., 2011). Phyllosphere microorganisms or phyllosphere microbiome can be beneficial to plants by (i) increasing stress tolerance (Lindow and Leveau, 2002; Redman et al., 2002; Hamilton et al., 2012), (ii) promoting plant growth, (iii) having a role in reproduction (Doty et al., 2009; Taghavi et al., 2009; Canto and Herrera, 2012), (iv) protecting plants against aerial danger like foliar pathogens (Lee et al., 2014), and (v) can be involved in the control of flowering phenology (Wagner et al., 2014). Importantly, these microorganisms also play important roles in earth’s biogeochemical cycles by moderating methanol emissions from plants (Galbally and Kirstine, 2002; Barud et al., 2016) and contributing to global nitrogen fixation (Fürnkranz et al., 2008). Despite this importance, knowledge of phyllosphere microbiomes remains relatively modest, especially for crops (Weyens et al., 2009; Vorholt, 2012; Hacquard and Schadt, 2015). To leverage, both above and below ground, plant microbiomes to support productivity and resilience of crops to environmental stresses in crops both above and below ground (Lebeis, 2014; Vandenkoornhuyse et al., 2015; Hassani et al., 2018), it is important to advance the knowledge on phyllosphere microbiome diversity and dynamics. The major roles of phyllosphere microbiome in healthy plants have been recently reviewed (Stone et al., 2018). Cultivation-independent studies have revealed that few bacterial phyla predominate in the phyllosphere of different plants and that plant factors are involved in shaping these phyllosphere communities; this is the result of specific adaptations and multipartite relationships among community members and with the host plant as reviewed by Vorholt (2012).
The rice plant (Oryza sativa), like other plants, has a microbial community showing differences according to the plant compartment (rhizospheric: root and phyllosphere: stem, leaves, sheath that protect the panicles). In the last decade, several studies reported an emerging rice disease that affects the phyllosphere part of the sheath tissue; the disease is called rice sheath rot. This disease has been mainly associated to Pseudomonas fuscovaginae which is a rice seedborne pathogen.
Microbiome and pathobiome studies on rice sheath rot have revealed that P. fuscovaginae is much more abundantly present in symptomatic rice plant samples with respect to asymptomatic samples (Musonerimana et al., 2020). Asymptomatic rice samples of the same rice variety in the same area/fields may possess a phyllosphere microbiome that promotes plant health and helps the plant fight sheath rot pathogen invasion. It was therefore of interest to perform an analysis of the culturable microbiome, create a bacterial culture collection isolates and characterize bacterial isolates from healthy/asymptomatic sheath rice plant samples.
Rice samples information
The asymptomatic samples of rice used to perform the analysis of the culturable microbiome and for the isolation of bacteria in order to create a culturable collection of possible P. fuscovaginae antagonists were collected in two different rice growing seasons; the wet season (2017) and the dry season (2018) in Burundi. The collected plant sheath samples were not surface sterilized. 1 g of each sample was then macerated in the presence of PBS and was stored in 18% glycerol at -80°C in final volume of 1.5 ml. Before plating, 1 ml of macerated and stored at -80°C of samples were recovered and then thawed. They were transferred in PBS with final volume of 10 ml and necessary diluted at 10 times. In total, 10 asymptomatic samples were used: 6 samples from wet season and 4 from dry season. All samples were from the highland location where the pathogen P. fuscovaginae was reported to be predominant in symptomatic samples (Musonerimana et al., 2020).
Bacterial strains isolation
The asymptomatic samples collected and used to perform a collection of bacterial isolates were rice sheath. They were used to prepare undiluted and diluted solutions. The undiluted and the 10-2 diluted solution were plated on TSA (Tryptic Soy Bean: 6 g + 16 g of Agar in 1 L of sterile water) and incubated at 28°C for 2 to 3 days. The bacteria grown from the undiluted solution plates were collected en masse for the genomic DNA extraction for 16S rRNA gene amplicon community sequencing and contributed to the culturable microbiome whereas the total microbiome was from total microbial DNA isolation from the sheath rice material. Single colonies from the 10-2 diluted solution plates were purified by selecting those different in shape, colour and margins appearing on inoculated plates and isolates were stored at -80°C in 20% sterile glycerol.
Bacterial strains identification
The bacterial isolates were prepared for molecular identification. For this the amplification of the 16S rRNA gene was performed by using Fd1 5’ AGAGTTTGATCCTGGCTCAG 3’ and Rp2 5’ ACGGCTACCTTGTTACGACTT 3’ primers set (Weisburg et al., 1991). Colony PCR was performed after boiling (10’ at 95°C) a colony suspension in 50 µL of sterile H2O. PCR amplification was performed using GoTaq® G2 Enzyme (Promega) according to the supplier’s instructions and 5 µL of template in a final volume of 50 μL was used for the PCR reaction. Reactions were performed in a T100™ Thermal Cycler (Biorad Laboratories Inc., Hercules, CA, USA) with the following thermal protocol: DNA denaturation for 5 min at 95°C, amplification (30 cycles) at 95°C for 30 s, 54°C for 30 s, and 72°C for 1 min 30 s, extension 7 min at 72°C. Agarose gel electrophoresis was run for the PCR products and DNA from agarose was purified by using EuroGold gel extraction kit (Euroclone SpA, Italy) according to the instructions of the manufacturer. The purified PCR DNA products (16S rRNA gene) were then sequenced with the 907F AAACTCAAAGGAATTGACG universal primer by Eurofins Genomics (Germany). Identification of the bacterial isolates at the genus level was obtained by BLAST analysis at NCBI (http://www.ncbi.nlm.nih.gov).
In vitro phenotypic assays
The bacterial isolates were tested for several in vitro phenotypes presumptive test. Assays for antibacterial activity against the rice bacterial plant pathogen P. fuscovaginae were performed by well diffusion, the culture and supernatant of each bacterial isolates were put in the middle of the TSA medium mixed with the pathogen at 0.1%; isolates were checked for lipolytic activity by streaking the bacterial isolates on 6 times diluted TSA medium amended with 1% tributyrin (Smeltzer et al., 1992); the proteolytic activity was tested by streaking the bacterial isolates on 6 times diluted TSA medium amended with 2% of powder milk (Huber et al., 2001); the exopolysaccharides (EPS) production was estimated by streaking the bacterial isolates on Yeast Extract Mannitol medium (Zlosnik et al., 2008); the indole acetic acid (IAA) production was tested by streaking the bacterial isolates on nitrocellulose membranes placed on TSA medium plates containing 5 mM tryptophan, incubating them for 24 h at 28°C and then removing the nitrocellulose membranes from TSA to place them onto a saturated Whatman paper that was previously treated with the Salkowski reagent (Bric et al., 1991); the IAA production resulted in the formation of a red/purple halo around the streak line growth of the bacterial isolates. Acyl homoserine lactones (AHLs) production was analysed by T-streak technique (Steindler and Venturi, 2007)using the biosensor Chromobacterium violaceum CV026 after incubation for 1 to 2 days. Motility was checked on M8 medium plates with 0.3% (swimming) or 0.5% (swarming) agar (Kohler et al., 2000).
Culturable microbiome and total microbiome analysis
Bacterial genomic DNA extraction
Bacterial genomic DNA extraction was performed from the culturable bacteria isolated from rice plant samples. The undiluted suspensions from 10 asymptomatic rice plant samples were plated on TSA medium and incubated at 28°C for 3 days. The bacteria grown were collected in 2 ml of PBS and used for genomic DNA extraction according to the Bacterial Genomic DNA extraction Kit instructions (QIAGEN, Hilden, D). The genomic DNAs extracted from 10 samples, 6 from the wet season and 4 from the dry season, were used to perform the 16S rRNA gene amplicon library as described subsequently. The same samples were used for performing the total microbiome as described by Musonerimana et al. (2020).
16S rRNA gene amplicon library preparation
The 16S rRNA gene amplicon library was performed by using the following primers: 16S Illumina library FW 5’TCGTCGGCAGCGTCAGATGTGTATAAGAGACAGCCTACGGGNGGCWGCAG and 16S Illumina library RW 5’GTCTCGTGGGCTCGGAGATGTGTATAAGAGACAGGACTACHVGGGTATCTAAATCC. A mix of 2.5 μl (5 ng/μl) microbial DNA, 5 μL (1 μM) of each primers and 12.5 μl of KAPA HiFi HotStart ReadyMix in final volume of 25 μl was used for the first PCR to amplify the V3 and V4 regions of 16S rRNA gene by following this program: initial denaturation of 95°C for 3 min followed by 25 cycles of 95°C for 30 s, 55°C for 30 s and 72°C for 30 s; and a final 5 min of extension at 72°C and hold at 4°C.
The PCR products were cleaned as described by Illumina protocol using AMPure XP beads, and a second PCR for adding the Illumina index was set. A mix of 5 μl (PCR products), 5 μl of each Nextera XT Index Primer (N7xx and S5xx), 25 μl of 2xKAPA HiFi HotStart ReadyMix and 10 μl PCR Grade water in final volume of 50 μl and the following program was used for the second PCR, initial denaturation of 95°C for 3 min, followed by 8 cycles of 95°C for 30 s, 55°C for 30 s, 72°C for 30 s; and a final 5 min of extension at 72°C and hold at 4°C. The second cleaning was done as recommended in the protocol always using AMPure XP beads.
The second cleaning amplification products of 16S rRNA gene amplicon libraries were quantified using the Qubit Kit (Invitrogen) and the quality (integrity and presence of a unique band) was confirmed by Bioanalyzer equipment (Agilent Inc., Santa Clara, CA, USA). After quantification and normalization, all PCR products were diluted to 4 nM and aliquots of 5 μl of diluted DNA from each library were pooled together and sent to sequence by Illumina Miseq sequencing platform.
Sequence data processing
FASTQ files were analysed using DADA2 v1.4.0 (Callahan et al., 2016)adapting the methods from the DADA2 Pipeline Tutorial (1.4). R version 3.5.2 was used for all analyses. Briefly, prior to analysis in DADA2, samples were demultiplexed using the QIIME 1.9.1 split_libraries_fastq.py script. The demultiplexed files were then used as the input for DADA2. Cutadapt 1.15 was used for adapter removal and quality filtering. Later quality profiles of the reads were analysed using the DADA2 function; plot Quality Profile, to determine positions at which read quality greatly decreases. Reads were then filtered and trimmed at the identified positions (truncLen=190) using the filterAndTrim function with standard parameters (maxN=0, truncQ=2, and maxEE=2). Dereplication was performed combining all identical sequencing reads into “unique sequences” with a corresponding “abundance” equal to the number of reads of that unique sequence. DADA2’s error model automatically filters out singletons, removing them before the subsequent sample inference step. Sample inference was performed using the inferred error model and chimeric sequences were removed using the removeBimeraDenovo function. The Greengenes (GG) database (McDonald et al., 2012), giving a final OTU table, was used to assign bacterial taxonomy using the assignTaxonomy function with a 97% sequence similarity.
Statistical analysis
The sequence table counts and rarefaction curves were determined on sequence count files generated by the analysis pipeline. The OTU table was rarefied according to the sample with the lowest number of reads, using the Rarefy function of the GUnifrac library. The resulting OTUs were clustered at the genus taxonomic level obtaining a final number of bacterial taxa for the two samplings. Statistical analyses were performed using the vegan package version 2.5-4 (Oksanen et al., 2019)and phyloseq package (McMurdie and Holmes, 2013)in R version 3.5.2 (Team, 2014). Relative abundances of OTUs between samples were calculated.
Culturable phyllosphere microbiome
It was of interest to compare the total microbiome of asymptomatic samples from highland during the two rice growing seasons (wet season: 2017 and dry season: 2018) the culturable microbiome detected under laboratory conditions performed on the same samples. In the samples collected in the wet season of 2017, 151 taxa were detected in the total microbiome from total microbial DNA isolation and 108 were detected in the culturable microbiome. In the samples collected during the dry season of 2018, 105 taxa were detected in the total microbiome and 88 taxa were detected in the culturable microbiome. Among the 151 different taxa inferred in the total microbiome from the wet season (see above), 29% of these were found to be culturable under the conditions tested here. Similarly, among the 108 taxa identified in the total microbiome from dry season, 31% of these were found to be culturable under laboratory conditions. The number of shared and unique taxa between total and culturable microbiome is shown in the Venn diagram (Figure 1a).
The number of different taxa observed in each sample and the comparison of the alpha diversity between total and culturable microbiome is as shown in Figure 1b. The difference in the mean value of different taxa observed between the total microbiome and the culturable microbiome is not significant for the asymptomatic samples from the wet season (2017) whereas is significant for the asymptomatic samples from the dry season (2018).
During the wet and dry seasons the most frequent/abundant genera among the asymptomatic samples from the highland were Herbaspirillum, Curtobacterium, Enterococcus, Methylobacterium, Rothia, Chryseobacterium, Pantoea, Streptococcus, Neisseria, Microbacterium and Sphingomonas (Table 1).
Bacterial strains isolation and identification from asymptomatic samples
It was also of interest to purify and isolate the bacteria present in asymptomatic samples since some of these could be involved in pathogen control; in total, 150 pure bacterial colonies were purified and isolated. The 16S rRNA gene amplification and sequencing enabled the classification of 58 bacterial isolates at the genus level. The 58 bacterial isolates belonged to 21 genera; among them 16 genera were also identified in the total and/or culturable microbiome study whereas surprisingly 5 were not. This result was most likely due to their very low amounts in the samples processed for culturable microbiome study. Among the 58 isolates collected, Microbacterium, Bacillus, Sphingomonas and Methylobacterium were the most predominant (Figure 2 and Table 1).
Most of the bacterial isolates belonged to Microbacterium, Sphingomonas, Methylobacterium and Bacillus genera.
In vitro phenotypes characterization of the bacterial isolates
To obtain more information on the ability of these bacterial isolates to exert a beneficial direct or indirect effect on the plant, several plant growth-promoting (PGP) activities and phenotypes were tested. The 58 bacterial isolates were tested for the following activities and phenotypes: proteolytic activity, exopolysaccharides production (EPS), antibacterial activity against P. fuscovaginae, IAA production, swarming and swimming motility. In summary, 24/58 bacterial isolates displayed proteolytic activity whereas no bacterial isolates displayed lipolytic activity. In addition, 17/58 bacterial isolates displayed EPS production, 7/58 displayed swimming motility, 19/58 displayed IAA production activity, 4/58 bacterial showed swarming motility and only 1/58 isolate had anti-P. fuscovaginae activity (Figure 3 and Table 2) which was observed on naked eyes. This latter bacterial isolate belongs to the Alcaligenes genus; interestingly this activity is not due to a protein since it was resistant to the strong protease pronase hence it is most likely a compound without amino acids and peptide bonds.
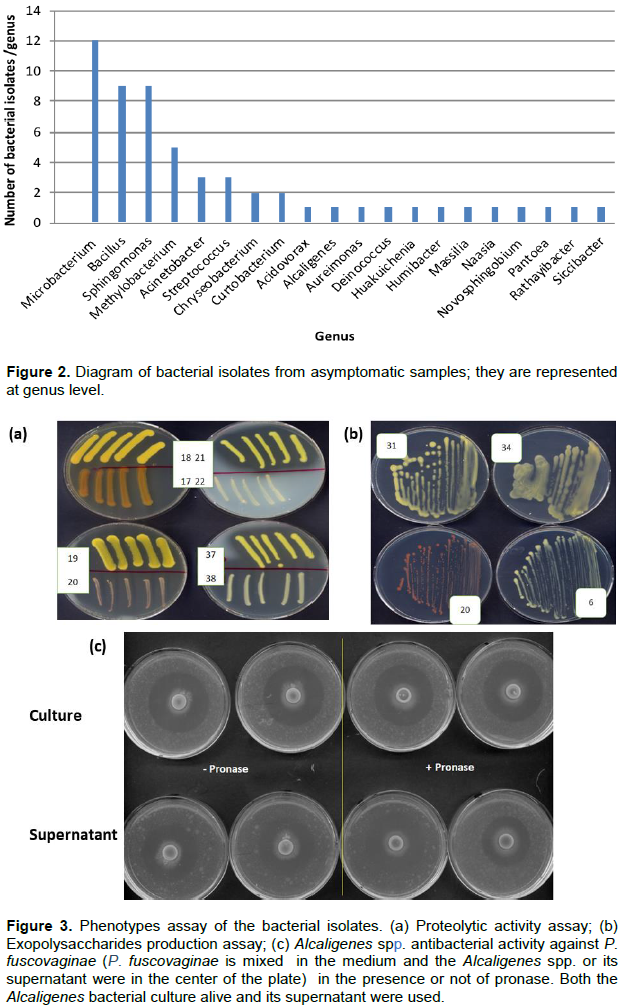
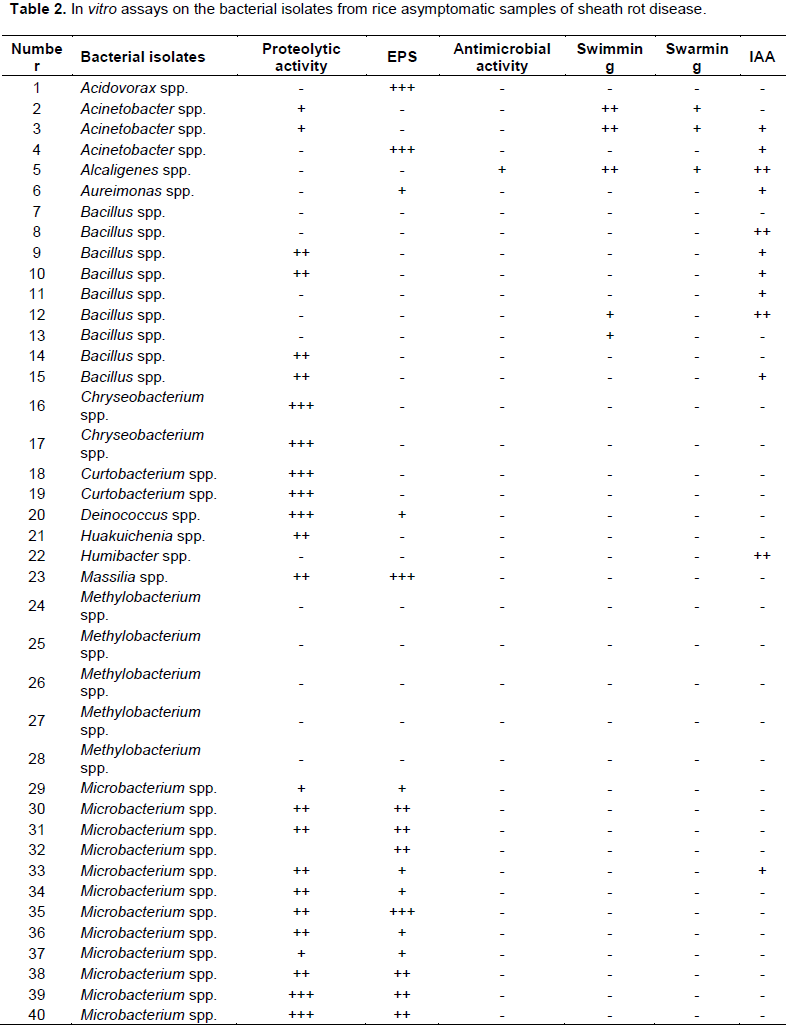
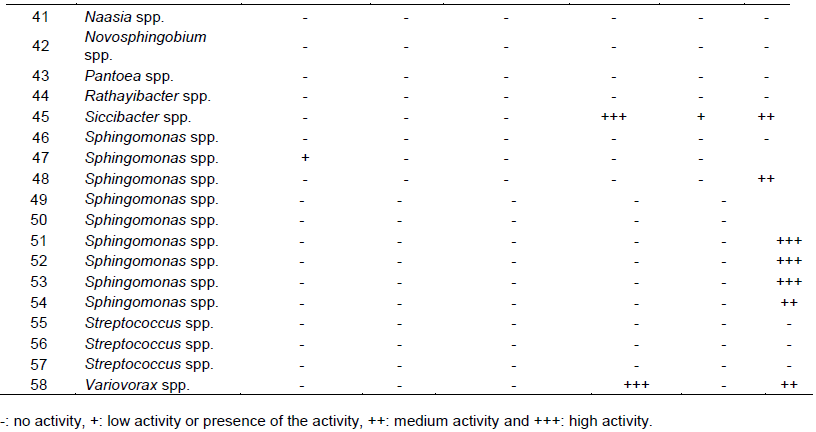
This study aimed to perform an analysis of the culturable microbiome, create a bacterial culture collection isolate and characterize bacterial isolates from healthy/asymptomatic sheath rice plant samples. These asymptomatic samples were collected from rice fields in Burundi, where the rice sheath rot disease is a serious issue. The total microbiome was performed on the same asymptomatic samples as presented (Musonerimana et al., 2020)and these had a high number of genera; 151 in 2017 and 105 in 2018. In comparison the culturable microbiome presented in this work revealed 108 genera in 2017 and 88 in 2018.
The comparison, between the total microbiome and culturable microbiome displayed some differences. Some genera were present in the total microbiome and not present in the culturable: most probably some genera in the total microbiome are unculturable or could not grow under the growth conditions used here or since the plant material was frozen, could not survive freezing. Surprisingly, some isolated bacteria of the culturable microbiome belong to genera that were not detected in total microbiome analysis: most likely the growth conditions used are optimal for them and besides these genera are present in very low abundance thus the total microbiome analysis did not detect them. Many of the genera in the culturable microbiome were mostly previously reported as being part of the rice phyllosphere microbiome like Methylobacterium, Sphingomonas and Microbacterium (Bertani et al., 2016). These bacteria that colonize the phyllosphere can adapt to a nutrient limiting environment and survive under high UV radiation (Stone et al., 2018). These members of the phyllosphere microbiome could be involved in providing the plant resistance to different stress conditions. Interestingly, the bacterial collection reported here possessed a few genera like Alcaligenes, Massilia, Rhayibacter that have not been reported previously to be associated with the phyllosphere of the rice plant. A possible reason is that the rice sheath samples used in this work were not surface sterilized meaning that bacterial isolates could contain endophytic and epiphytic bacteria. Many isolates belong to the genus Microbacterium, Sphingomonas, Bacillus and Methylobacterium; this could have been caused by enrichment due to the isolation conditions, especially the culture medium (TSA) and the temperature of growth.
The in vitro assays performed on the 58 bacterial isolates showed a diversity of phenotypes; 24 isolates had a proteolytic activity which is an important property involved in the virulence of plant pathogens (Figaj et al., 2019)as well as in the biological control of plant disease (Mota et al., 2017). 17 isolates were able to produce EPS, these molecules are known to be produced also by some plant pathogens like Pseudomonas and the EPS produced by Pseudomonas syringae is involved in biofilm formation, virulence and epiphytic fitness (Yu et al., 1999; Laue et al., 2006). It is possible that bacterial EPS provides some protection to the plant, both from desiccation and from UV damage. Biofilms in the phyllosphere may provide resistance to desiccation unlike those found in water; for example, Pseudomonas putida biofilms grown in air retained their morphology better after drying than biofilms were grown in liquid medium (Auerbach et al., 2000). Pseudomonas species are often dominant constituents of the phyllosphere suggesting that naturally occurring biofilms may limit the loss of water and exposure to UV radiation. Plants are exposed to high levels of UV radiation and can suffer developmental and genetic damage (Jansen et al., 1998). Pigmented bacteria are more UV resistant, and the phyllosphere microbiome as a whole becomes more UV tolerant towards the end of the growing season (Jacobs and Sundin, 2001). Phyllosphere microorganisms may provide some UV protection to the plant through pigmented compounds; interestingly several isolates (many Microbacterium species) produced EPS that were yellow pigmented. It is also known that EPS production is involved in the endophytic colonization of Gluconacetobacter diazotrophicus since EPS mutants were defective in the colonization of the rice root endosphere (Meneses et al., 2011).
IAA was produced mostly by isolates that belonged to Bacillus and Sphingomonas. The plant hormone (IAA) from phyllosphere microorganisms influences plant growth and the evidence suggests that phyllosphere microorganisms producing it could be involved in increasing plant productivity (Glick, 1995; Romero et al., 2016)and also be involved in the activity of stomata (Tanaka et al., 2006). Swimming and swarming movement was detected on a few isolates; these phenotypes can have an important role in the motility for the acquisition of nutrients.
The antimicrobial activity assay against P. fuscovaginae showed that only one isolate belonging to the Alcaligenes genus displayed a positive test in vitro. Alcaligenes species strains exist in soil, water, and environment, as well as in association with humans. The bacteria of this genus are usually non-pathogenic but occasionally can cause opportunistic human infections. Bacterial species belonging to the genus Alcaligenes have also demonstrated versatile pollutant bioremediation capability, including phenols (Rehfuss and Urban, 2005; Kumar et al., 2013), phenanthrene (Singleton et al., 2009)as well as having algicidal activity (Sun et al., 2015). The in vitro antimicrobial assay performed here excludes the isolates that attenuate/block P. fuscovaginae pathogenesis/ invasion via other mechanisms like competition for nutrients or quorum quenching. It is therefore likely other bacteria that live in the phyllosphere are involved in promoting plant health by keeping away pathogens like P. fuscovaginae.
The authors have not declared any conflict of interests.
This study was supported by an ICGEB Arturo Falaschi fellowship. The authors thank ICGEB for financial support and IRRI-Burundi for laboratory financial support and providing the material samples used.
REFERENCES
Auerbach ID, Sorensen C, Hansma HG, Holden PA (2000). Physical morphology and surface properties of unsaturated Pseudomonas putida biofilms. Journal of Bacteriology 182(13):3809-3815.
|
|
Barud HS, Gutierrez J, Lustri WR, Peres MFS, Ribeiro SJL, Saska S, Tercjak A (2016). Bacterial Cellulose. In: Biomaterials from Nature for Advanced Devices and Therapies First Edition, Chap. 21.
|
|
|
Bertani I, Abbruscato P, Piffanelli P, Subramoni S, Venturi V (2016). Rice bacterial endophytes: Isolation of a collection, identification of beneficial strains and microbiome analysis. Environmental Microbiolology Reports 8(3):388-398.
|
|
|
Bric JM, Bostock RM, Silverstone SE (1991). Rapid in situ assay for indoleacetic acid production by bacteria immobilized on a nitrocellulose membrane. Applied Environmental Microbiology 57(2):535-538.
Crossref
|
|
|
Callahan BJ, McMurdie PJ, Rosen MJ, Han AW, Johnson AJA, Holmes SP (2016). DADA2: High-resolution sample inference from Illumina amplicon data. Nature Methods 13(7):581-583.
|
|
|
Canto A, Herrera CM (2012). Micro-organisms behind the pollination scenes: Microbial imprint on floral nectar sugar variation in a tropical plant community. Annals of Botany 110(6):1173-1183.
Crossref
|
|
|
Doty SL, Oakley B, Xin G, Kang JW, Singleton G, Khan Z, Vajzovic A, Staley JT (2009). Diazotrophic endophytes of native black cottonwood and willow. Symbiosis 47(1):23-33.
Crossref
|
|
|
Edwards J, Johnson C, Santos-Medellín C, Lurie E, Podishetty NK, Bhatnagar S, Eisen JA, Sundaresan V, Jeffery LD (2015). Structure, variation, and assembly of the root-associated microbiomes of rice. Procedings of National Acadademy of Sciences of USA 112(8):911-920.
Crossref
|
|
|
Figaj D, Ambroziak P, Przepiora T, Skorko-Glonek J (2019). The role of proteases in the virulence of plant pathogenic bacteria. International Journal of Molecular Sciences 20(3):672.
Crossref
|
|
|
Foley JA, Ramankutty N, Brauman KA, Cassidy ES, Gerber JS, Johnston M, Mueller ND, O'Connell C, Ray DK, West PC, Balzer C, Bennett EM, Carpenter SR, Hill J, Monfreda C, Polasky S, Rockström J, Sheehan J, Siebert S, Tilman D, Zaks DPM (2011). Solutions for a cultivated planet. Nature 478(7369):337-342.
Crossref
|
|
|
Fürnkranz M, Wanek W, Richter A, Abell G, Rasche F, Sessitsch A (2008). Nitrogen fixation by phyllosphere bacteria associated with higher plants and their colonizing epiphytes of a tropical lowland rainforest of Costa Rica. The ISME Journal 2(5):561-570.
Crossref
|
|
|
Galbally IE, Kirstine W (2002). The production of methanol by flowering plants and the global cycle of methanol. Journal of Atmospheric Chemistry 43(3):195-229.
Crossref
|
|
|
Glick BR (1995). The enhancement of plant growth by free-living bacteria. Canadian Journal of Microbiology 41(2).
Crossref
|
|
|
Gopal M, Gupta A (2016). Microbiome selection could spur next-generation plant breeding strategies. Frontiers in Microbiology 7(e1002352):1971.
Crossref
|
|
|
Hacquard S, Schadt CW (2015). Towards a holistic understanding of the beneficial interactions across the Populus microbiome. New Phytologist 205(4):1424-1430.
Crossref
|
|
|
Hamilton CE, Gundel PE, Helander M, Saikkonen K (2012). Endophytic mediation of reactive oxygen species and antioxidant activity in plants: A review. Fungal Diversity 54:1-10.
Crossref
|
|
|
Hassani MA, Durán P, Hacquard S (2018). Microbial interactions within the plant holobiont. Microbiome 6:58.
Crossref
|
|
|
Huber B, Riedel K, Hentzer M, Heydorn A, Gotschlich A, Givskov M, Molin S, Eberl L (2001). The cep quorum-sensing system of Burkholderia cepacia H111 controls biofilm formation and swarming motility. Microbiology 147(9):2517-2528.
Crossref
|
|
|
Jacobs JL, Sundin GW (2001). Effect of Solar UV-B Radiation on a Phyllosphere Bacterial Community. Applied and Environmental Microbiology 67(12):5488-5496.
Crossref
|
|
|
Jansen MAK, Gaba V, Greenberg BM (1998). Higher plants and UV-B radiation: Balancing damage, repair and acclimation. Trends in Plant Science 3(4):131-135.
|
|
|
Kohler T, Curty LK, Barja F, Van Delden C, Pechere JC (2000). Swarming of Pseudomonas aeruginosa is dependent on cell-to-cell signaling and requires flagella and pili. Journal of Bacteriology 182(21):5990-5996.
|
|
|
Kumar A, Bhunia B, Dasgupta D, Mandal T, Dey A, Datta S, Bhattacharya P (2013). Optimization of culture condition for growth and phenol degradation by Alcaligenes faecalis JF339228 using Taguchi Methodology. Desalination and Water Treatment 51(16-18):3153-3163.
|
|
|
Laue H, Schenk A, Li H, Lambertsen L, Neu TR, Molin S, Ullrich MS (2006). Contribution of alginate and levan production to biofilm formation by Pseudomonas syringae. Microbiology 152(10):2909-2918.
|
|
|
Lebeis SL (2014). The potential for give and take in plant-microbiome relationships. Frontiers in Plant Science 5(287):287.
Crossref
|
|
|
Lee DW, Hong JS, Kim SH, Kim JW, Kim BS (2014). First Report of Pseudomonas lurida Causing Bacterial Leaf Spot on Miscanthus sinensis. Journal of Phytopathology 162(3):195-200.
|
|
|
Lindow SE, Brandl MT (2003). MINIREVIEW Microbiology of the Phyllosphere. Applied and Environmental Microbiology 69(4):1875-83.
Crossref
|
|
|
Lindow SE, Leveau JHJ (2002). Phyllosphere microbiology. Current Opinion in Biotechnology 13(3):238-243.
|
|
|
McDonald D, Price MN, Goodrich J, Nawrocki EP, Desantis TZ, Probst A, Andersen GL, Knight R, Hugenholtz P (2012). An improved Greengenes taxonomy with explicit ranks for ecological and evolutionary analyses of bacteria and archaea. The ISME Journal 6(3):610-618.
|
|
|
McMurdie PJ, Holmes S (2013). Phyloseq: An R Package for Reproducible Interactive Analysis and Graphics of Microbiome Census Data. PLoS One 8(4):e61217.
|
|
|
Meneses CHSG, Rouws LFM, Simões-Araújo JL, Vidal MS, Baldani JI (2011). Exopolysaccharide production is required for biofilm formation and plant colonization by the nitrogen-fixing endophyte Gluconacetobacter diazotrophicus. Molecular Plant-Microbe Interactions 24(12):1448-1458.
|
|
|
Mota MS, Gomes CB, Souza Júnior IT, Moura AB (2017). Bacterial selection for biological control of plant disease: criterion determination and validation. Brazilian Journal of Microbiology 48(1):62-70.
|
|
|
Musonerimana S, Bez C, Licastro D, Habarugira G, Bigirimana J, Venturi V (2020). Pathobiomes Revealed that Pseudomonas fuscovaginae and Sarocladium oryzae Are Independently Associated with Rice Sheath Rot. Microbial Ecology 80(5):627-642.
|
|
|
Oksanen J, Blanchet FG, Friendly M, Kindt R, Legendre P, Mcglinn D, Minchin PR, O'hara RB, Simpson GL, Solymos P, Henry M, Stevens H, Szoecs E, Maintainer HW (2019). vegan: Community Ecology Package. R package version 2.5-5. Community Ecology Package.
|
|
|
Peñuelas J, Terradas J (2014). The foliar microbiome. Trends in Plant Science 19(5):278-280.
Crossref
|
|
|
Redman RS, Sheehan KB, Stout RG, Rodriguez RJ, Henson JM (2002). Thermotolerance generated by plant/fungal symbiosis. Science 298(5598):1581.
|
|
|
Rehfuss M, Urban J (2005). Alcaligenes faecalis subsp. phenolicus subsp. nov. a phenol-degrading, denitrifying bacterium isolated from a graywater bioprocessor. Systematic and Applied Microbiology 28(5):421-429.
|
|
|
Romero FM, Marina M, Pieckenstain FL (2016). Novel components of leaf bacterial communities of field-grown tomato plants and their potential for plant growth promotion and biocontrol of tomato diseases. Research in Microbiology 167(3):222-233.
|
|
|
Singleton DR, Ramirez LG, Aitken MD (2009). Characterization of a polycyclic aromatic hydrocarbon degradation gene cluster in a phenanthrene-degrading Acidovorax strain. Applied and Environmental Microbiology 75(9):2613-2620.
|
|
|
Smeltzer MS, Hart ME, Iandolo JJ (1992). Quantitative spectrophotometric assay for staphylococcal lipase. Appl Environ Microbiol. Applied and Environmental Microbiology 58(9):2815-2819.
|
|
|
Steindler L, Venturi V (2007). Detection of quorum-sensing N-acyl homoserine lactone signal molecules by bacterial biosensors. FEMS Microbiology Letters 266(1):1-9.
|
|
|
Stone BWG, Weingarten EA, Jackson CR (2018). The Role of the Phyllosphere Microbiome in Plant Health and Function. In: Annual Plant Reviews online pp. 533-556.
|
|
|
Sun P, Lin H, Wang G, Zhang X, Zhang Q, Zhao Y (2015). Wheat bran enhances the cytotoxicity of immobilized Alcaligenes aquatilis F8 against Microcystis aeruginosa. PLoS ONE 10(8):e0136429.
|
|
|
Taghavi S, Garafola C, Monchy S, Newman L, Hoffman A, Weyens N, Barac T, Vangronsveld J, Van Der Lelie DD (2009). Genome survey and characterization of endophytic bacteria exhibiting a beneficial effect on growth and development of poplar trees. Applied and Environmental Microbiology 75(3):748-757.
|
|
|
Tanaka Y, Sano T, Tamaoki M, Nakajima N, Kondo N, Hasezawa S (2006). Cytokinin and auxin inhibit abscisic acid-induced stomatal closure by enhancing ethylene production in Arabidopsis. Journal of Experimental Botany 57(10):2259-2266.
|
|
|
Team RC (2014). R A Lang Environ Stat Comput R Found Stat Comput Vienna, Austria URL
View
|
|
|
Vandenkoornhuyse P, Quaiser A, Duhamel M, Le Van A, Dufresne A (2015). The importance of the microbiome of the plant holobiont. New Phytologist 206(4):1196-1206.
Crossref
|
|
|
Vorholt JA (2012). Microbial life in the phyllosphere. Nature Reviews Microbiology 10(12):828-840.
Crossref
|
|
|
Wagner MR, Lundberg DS, Coleman-Derr D, Tringe SG, Dangl JL, Mitchell-Olds T (2014). Natural soil microbes alter flowering phenology and the intensity of selection on flowering time in a wild Arabidopsis relative. Ecology Letters 17(6):717-726.
|
|
|
Weisburg WG, Barns SM, Pelletier DA, Lane DJ (1991). 16S ribosomal DNA amplification for phylogenetic study. Journal of Bacteriology 173(2):697-703.
|
|
|
Weyens N, van der Lelie D, Taghavi S, Newman L, Vangronsveld J (2009). Exploiting plant-microbe partnerships to improve biomass production and remediation. Trends in Biotechnology 27(10):591-598.
|
|
|
Yu J, Penaloza-Vázquez A, Chakrabarty AM, Bender CL (1999). Involvement of the exopolysaccharide alginate in the virulence and epiphytic fitness of Pseudomonas syringae pv. syringae. Molecular Microbiology 33(4):712-720.
|
|
|
Zlosnik JEA, Hird TJ, Fraenkel MC, Moreira LM, Henry DA, Speert DP (2008). Differential mucoid exopolysaccharide production by members of the Burkholderia cepacia complex. Journal of Clinical Microbiology 46(4):1470-1473.
|
|