ABSTRACT
In this study, strawberry seedlings were treated with 250 mmol·L-1 NaCl solution; then the O2- production rate, the content of H2O2 and thiobarbituric acid reactive substance (TBARS), electrolyte leakage (EL), the enzyme activity of superoxide dismutase (SOD), peroxidase (POD), and catalase (CAT) in the root tip cells were determined to be used for analysis after salt stress. The results revealed that the O2- production rate, EL, and the content of TBARS showed an increasing trend with the time of salt stress, while the content of H2O2, the enzyme activity of SOD, POD and CAT exhibited a pattern of increasing first and decreasing later under the salt stress. In conclusion, the results demonstrated that the oxidative damage in root tip cells of strawberry was increased with the time of salt stress, and the enhanced antioxidant activity was not enough to eliminate the peroxidation damage which was caused by 250 mmol·L-1 NaCl solution.
Key words: Strawberry root tip, salt stress, the activities of the antioxidant enzymes.
Roots are not only the most important nutrient uptake organs of plants, but also the most sensitive parts of salt stress, and promote the plant to make a further response to stress. Roots response to salt stress mainly includes growth inhibition, osmotic regulation, protects the enzyme system to operate effectively, the metabolism of active oxygen, the changes of membrane structure and function in the root (Bohra and Doerffling, 1993). Salt stress leads to accumulation of a variety of reactive oxygen species (ROS) in plant cells, such as H2O2 and O2-; the excessive ROS is toxic to the plant cells and causes the peroxidation of membrane lipid, which leads to an increase in the level of EL level and the content of TRABS, and degradation of biological molecules such as proteins, DNA molecules and carbohydrates (Rusty and Regina, 2005; KOC, 2015; Yang and Guo, 2018), thus affecting the normal functions of these molecules, which interfere with the normal physiological activities of the cells. Further research indicates that the imbalance of ROS metabolism is the primary reason that salt stress leads to the over-oxidation injury in plant cells and cell death (Katsuhara and Kawasaki, 1996). There are non-enzymatic antioxidant systems, and antioxidant enzyme systems in the plants to eliminate the peroxidation damage caused by salt stress and to maintain the normal growth of plants.
The strawberry (Fragaria × ananassa Duch) is the collective name of Rosaceae family plants, belonging to perennial herbaceous plants, the fruits are red berries, soft and succulent, with unique flavor, so it is popular with people. With facilities in the development of agricultural technology, the cultivation facilities of strawberry have
also been developed rapidly. However, secondary salinization of the soil gradually becomes an important factor limiting the greenhouse cropping system, which affects the improvement of the yield and quality of strawberry. Therefore, the study on the damage to strawberry growth and root system caused by high concentration salt stress becoming an important subject for the future development of strawberry industry. In this work, the strawberry root tip was used to analyze antioxidant activity and peroxidation damage under the salt stress, which provides a theoretical basis for the study of the effects of salt stress on the physiological characteristics of strawberry.
Plant and treatment
Forty-five-days old strawberry (Fragaria × ananassa Duch. cv. Fengxiang) plants were used in this study; the uniform and healthy seedlings were pre-cultured with 1/2 strength Hoagland’s nutrient solution for three weeks until the growth of roots at about 6 cm. Then, the strawberry seedlings were divided into two groups (control and stress treatment) and transferred into Hoagland’s nutrient solution and high-salinity 1/2-strength Hoagland’s nutrient solution (250 mmol·L-1 NaCl), and then the 1 cm roots tip samples were harvested at 0, 12, 24, 48 and 72 h, respectively. Then, the samples were frozen immediately in liquid nitrogen and stored at -80°C for the further experiments. The strawberry seedlings were kept in a growth chamber with a light/dark regime of 16 h/8 h at 24°C, 75% relative humidity, and a light intensity of 800 µmol m-2 s-1 of photosynthetically active radiation. The culture solution was as follows: 6 mmol·L-1 KNO3, 4 mmol·L-1 Ca(NO3)2, 2 mmol·L-1 NH4H2PO4, 1 mmol·L-1 MgSO4, 50 μmol·L-1 KCl, 10 μmol·L-1 H3BO3, 2 μmol·L-1 MnSO4, 2 μmol·L-1 ZnSO4, 0.5 μmol·L-1 CuSO4, 0.065 μmol·L-1 (NH4)6Mo7O24, and 40 μmol·L-1 Fe–EDTA (Hoagland and Arnon, 1950). The nutrient solution was ventilated for 30 min every 2 h by the combined action of the timer (Pinyi AL-06, China) and ventilation pump (SUNSUN ACO, China); the hydroponic system is shown in Supplementary Figure 1 and replaced once a day. The pH of all nutrient solutions was adjusted to 6.5 with 0.1 M KOH. Three biological replicates were used for each sample and 50 seedlings were used for each group.
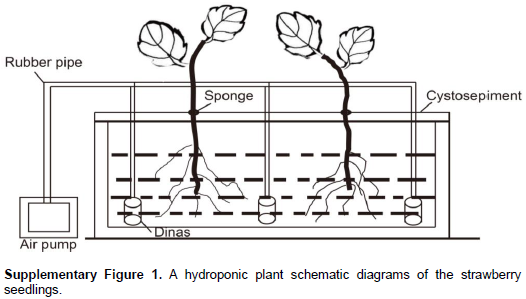
Physiological measurement
The measurement of electrolyte leakage (EL) referred to the method used by Heath and Packer 1968), and the content of thiobarbituric acid reactive substance (TBARS) was measured by adding an equal aliquot of 0.5% TBA in 20% trichloroacetic acid to an aliquot of the incubation mixture containing a few grains of sand used as a boiling aid. The solution was heated 25 min at 95°C, and then centrifuged for 1 min in a clinical centrifuge to clarify the solution. Absorbancy was measured at 532 nm corrected for nonspecific turbidity by subtracting the absorbancy at 600 nm (Fu and Huang, 2001). H2O2 was extracted and measured by Mukherjee and Choudhuri (1983), and brief method is as follows: 0.5 mL potassium phosphate buffer with pH=7.8 was added to the test tube containing the sample, and then 0.1 mL with 10 mmol/L hydroxylamine hydrochloride were added to the experimental sample. After 20 min of water bath (25°C), 1 mL with 58 mmol/L of sulfonamide and 1 mL with 7 mmol/L of alpha-naphthalamine were added to the experimental sample. After 20 min of water bath (25°C), the light absorption value of the sample at 530 nm was measured. O2- production rate were measured by Elstner and Heupel (1976), and the brief method is as follows: isolation was made from 5 g of tissue in ice cold acetone. By addition of 5% (w/v) titanyl sulphate and conc. NH4OH solution, the peroxide-titanium complex was precipitated. This was dissolved in 15 ml of 2 M H2SO2, marking the final volume 20 ml with cold water. The absorbance was read at 415 nm against a water blank. The H2O2 content was calculated from a standard curve prepared in a similar way. Activities of catalase (CAT), peroxidase (POD) and superoxide dismutase (SOD) referred to the method used by Chen and Cheng (2003)and Giannopolitis and Ries (1977), respectively. For CAT, the decomposition of H2O2 was measured by the decline in absorbance at 240 nm for 1 min. The 3 ml reaction mixture contained 0.1 ml enzyme extract, 15 mM H2O2 and 50 mM phosphate buffer (pH 7.0), which initiated the reaction. For POD, the oxidation of guaiacol was measured by the increase in absorbance at 470 nm for 1 min. The reaction mixture contained 0.1 ml enzyme extract, 50 μl of 20 mM guaiacol and 2.8 ml of 10 mM phosphate buffer (pH 7.0). The reaction was started with 20 μl of 40 mM H2O2. For SOD, a 3 ml reaction mixture contained 20 μl of enzyme extract, 1.3 μM riboflavin, 63 μM NBT, 13 mM methionine, 0.1 mM EDTA, and 50 mM phosphate buffer (pH 7.8). The test tubes containing the mixture were placed under light at 78 μmol photons s-1 m-2 for 10 min and absorbance at 560 nm was recorded. Each treatment was repeated three times.
Statistical analysis
Three replicates (three plants per replicate) were used for all experimental treatment. Statistical analyses of the data were performed using the SPSS statistical package and the differences were statistically compared by employing the Duncan test with a significance level of p < 0.05.
To evaluate the degree of oxidation of strawberry root tip cells, the O2- production rate and the content of H2O2 were measured. As shown in Figure 1A, the production rate of O2- did not change notably in the control, however, the production rate of O2- went up steadily until reaching the peak value at 72 h in the strawberry root tip cells under the salt stress treatment, nearly 2.1 times higher than the control. There was no significant change in the content of H2O2 in the root tip of the control, while the content of H2O2 rose steadily at the root tip of strawberry until reaching the peak at 36 h under salt stress, which was 2.4-fold increase relative to the control, and the content of H2O2 decreased afterwards, but was still significantly higher than the control (Figure 1B).
TBARS was one of the main products of membrane lipid peroxidation, which shows the degree of oxidative damage in plant cells. As shown in Figure 2A, the content of TBARS had been higher than the control after 12 h salt stress treatment in the root tip cells of strawberry, and then continued to rise until reaching the maximum value at 72 h, almost 3 times as much as the control. In this period, there were few changes in the control (Figure 2A).
In addition, there was the same trend of the level of EL and the content of TBARS in both control and treatments, and the level of the EL reached the peak value at 72 h in the root tip cells of strawberry under salt stress, which was 3.5-fold increase relative to the control (Figure 2B). These results indicated that salt stress caused injury to the membrane in the root tip cells of strawberry, which increased the permeability of plasma-membrane leading to increase in the TBARS content and the EL.
Three significant antioxidant enzymes (SOD, POD and CAT) were important factors for scavenging ROS, which proved to be the most effective in maintaining the balance of ROS in plant cells. In the present study, the activities of the three antioxidant enzymes were measured under normal condition and salt stress treatment with the extension of processing time. As can be seen in Figure 3A, the activities of SOD in the root tip cells of strawberry had no significant changes under normal condition, while the activities of SOD rise during early salt stress treatment, but tended to decline after 24 h and became significantly higher than the control at 72 h. The activities of POD and SOD in the root tip cells of strawberry showed similar tendency, except the activities of POD in control were higher than in the case of salt stress treatment at 72 h (Figure 3B). Besides that, the variation patterns of CAT enzyme activities were similar to that of POD enzyme activities (Figure 3C).
Salt stress causes a variety of injuries to plant cells, among which ion toxic, osmotic stress and the accumulation of ROS are the particular problems (Mittler, 2002). ROS is the secondary metabolite in plant photosynthesis and respiration, which primarily is generated in some oxidation of some mitochondrial enzymes, chloroplasts and peroxisome. Under normal growth conditions, the generation and depletion of ROS are kept in a state of dynamic balance, nevertheless, the state of balance is easy to destroy to generate excessive ROS in plant cells in the abiotic stress conditions which leads to oxidative stress occurrence and cell damage (Faghih et al., 2017). Under salt stress, the fixed rate of CO2 is limited, leading to increase in the suppression of electron flow in the photosynthetic chains, thus excessive superoxide anion, other free radicals and active oxygen such as H2O2 and O2- are generated (Gill and Tuteja, 2010). In this research, we found that the O2- production rate and the content of H2O2 in the root tip cells of strawberry increased significantly as stress time increased, which is supported by earlier studies, such as soybean, wheat and chickpea. Under the condition of oxidative stress, ROS are so extremely active that lead to membrane lipid peroxidation. Fortunately, the existence of antioxidant enzymes such as POD, SOD and CAT in the plant cells can reduce the damage by the peroxidation. However, the present study shows that though the activities of antioxidant enzyme are elevated, but there are still high EL level and TBARS content in the root tip cells of strawberry under salt stress treatment, which are similar to the experimental results of Christou et al. (2014), showing that the antioxidant system does not provide enough protection to prevent the root tip cells of strawberry from the oxidative damage, and the results of this study are similar to the results reported by Rasool et al. (2013). In addition, although the activity of antioxidant enzymes increased in the early stage of salt stress, with the extension of stress time, the ability of antioxidant system to scavenge reactive species is gradually reduced, which further aggravates lipid peroxidation reaction in the root tip cells of strawberry, which are supported by Christou et al. (2014) and Gill and Tuteja (2010).
The authors have not declared any conflict of interests.
This work was supported by China Spark Program (2015GA690287), Policy Guidance Technology (industry-university-institute cooperation)-prospective joint research project in Jiangsu Province (BY2016061-26), the Postgraduate Practical Innovation Project in Jiangsu Province (SJCX17-07 01), and the Jiangsu Provincial Natural Science Foundation (BK20170463).
REFERENCES
Bohra JS, Doerffling K (1993). Potassium nutrition of rice (Oryza sativa L.) varieties under NaCl salinity. Plant and Soil 152(2):299-303.
Crossref
|
|
Chen LS, Cheng L (2003). Both xanthophyll cycleâ€dependent thermal dissipation and the antioxidant system are upâ€regulated in grape (Vitis labrusca L. cv. Concord) leaves in response to N limitation. Journal of Experimental Botany 54(390):2165-2175.
Crossref
|
|
|
Christou A, Manganaris GA, Fotopoulos V (2014). Systemic mitigation of salt stress by hydrogen peroxide and sodium nitroprusside in strawberry plants via transcriptional regulation of enzymatic and non-enzymatic antioxidants. Environmental and Experimental Botany 107(22):46-54.
Crossref
|
|
|
Elstner EF, Heupel A (1976). Inhibition of nitrite formation from hydroxylammoniumchloride: A simple assay for superoxide dismutase. Analytical Biochemistry 70(2):616-620.
Crossref
|
|
|
Faghih S, Ghobadi C, Zarei A (2017). Response of strawberry plant cv. 'Camarosa' to salicylic acid and methyl jasmonate application under salt stress condition. Journal of Plant Growth Regulation 36(3):1-9.
Crossref
|
|
|
Fu J, Huang B (2001). Involvement of antioxidants and lipid peroxidation in the adaptation of two cool-season grasses to localized drought stress. Environmental and Experimental Botany 45(2):105-114.
Crossref
|
|
|
Giannopolitis CN, Ries SK (1977). Superoxide dismutases: I. Occurrence in higher plants. Plant physiology 59(2):309-314
Crossref
|
|
|
Gill SS, Tuteja N (2010). Reactive oxygen species and antioxidant machinery in abiotic stress tolerance in crop plants. Plant Physiology and Biochemistry 48(12):909-930.
Crossref
|
|
|
Heath RL, Packer L (1968). Photoperoxidation in isolated chloroplasts: I. Kinetics and stoichiometry of fatty acid peroxidation. Archives of Biochemistry and Biophysics 125(1):189-198.
Crossref
|
|
|
Hoagland DR, Arnon DI (1950). The water-culture method for growing plants without soil. Circular California Agricultural Experiment Station 347:3-32.
|
|
|
Katsuhara M, Kawasaki T (1996). Salt stress induced nuclear and DNA degradation in meristematic cells of barley roots. Plant and Cell Physiology 37(2):169-173.
Crossref
|
|
|
KOC A (2015). Effect of plant growth-promoting bacteria and arbuscular mycorrhizal fungi on lipid peroxidation and total phenolics of strawberry (Fragaria × ananassa 'San Andreas') under salt stress. Turkish Journal of Agriculture and Forestry 39(6):992-998.
Crossref
|
|
|
Mittler R (2002). Oxidative stress, antioxidants and stress tolerance. Trends in Plant Science 7(9):405-410.
Crossref
|
|
|
Mukherjee SP, Choudhuri MA (1983). Implications of water stress-induced changes in the levels of endogenous ascorbic acid and hydrogen peroxide in Vigna seedlings. Physiologia plantarum 58(2):166-170.
Crossref
|
|
|
Rasool S, Ahmad A, Siddiqi TO, Ahmad P (2013). Changes in growth, lipid peroxidation and some key antioxidant enzymes in chickpea genotypes under salt stress. Acta Physiologiae Plantarum 35(4):1029-1050.
Crossref
|
|
|
Rusty R, Regina R (2005). Balancing the generation and elimination of reactive oxygen species. Proceedings of the National Academy of Sciences of the United States of America 102(9):3175-3176.
Crossref
|
|
|
Yang Y, Guo Y (2018). Elucidating the molecular mechanisms mediating plant salt-stress responses. New Phytologist 217(2):523-539.
Crossref
|
|