ABSTRACT
Seafood is part of a healthful diet, but seafood consumption is not risk-free because of sea contaminations by anthropogenic activities and environmental factors. Their microbiological quality is of major concern to the food processors, consumers and public health authorities hence the need for proper assessment of the microbial quality. This study therefore characterizes pathogenic microorganisms isolated from fresh sea snail and shrimp in Ugbo nla, Igbokoda, Ondo State, Nigeria. Isolation and molecular identification of microorganisms from snail and shrimp using 16sRNA and 18sRNA gene sequence for bacteria and fungi respectively, effects of salt concentration and temperature on isolated microorganisms were carried out using standard microbiological methods. Molecular identification revealed the presence different pathogens namely; Proteus mirabilis LZPM-03, Pantoea agglomerans BJCP2, Pseudomonas aeruginosa R8-589, Klebsiella pneumoniae 2N3, Serratia marcescens, Alcaligenes faecalis ACBC1, Yersinia. pseudotubercules YPIII, Escherichia coli 2A, Lysinibacillus sphaericus C3-41, Listeria monocytogen J1776, Staphylococcus aureus and Proteus mirabilis LCX20, Fusarium proliferatum FproStRINI, Aspergillus flavus NRRL3357, Aspergillus oryzae RIB40 and Aspergillus peniciliodes. Also, 4% salt concentration at 45°C effectively inhibited the pathogens isolated from seafood after 18 h. The presence of pathogenic microorganisms in the sea foods could make them a potential health-risk when consumed without proper cooking. Salting, a traditional technique could be used to eliminate these pathogens before consumption.
Key words: Seafood, molecular identification and salt concentration.
An aquatic ecosystem is an ecosystem in a water body. In aquatic ecosystem, communities of organisms are dependent on each other and on their environment. The two main types of aquatic ecosystems are marine ecosystems and freshwater ecosystems. Freshwater ecosystems cover 0.78% of the Earth's surface, inhabit 0.009% of its total water, and generate nearly 3% of its net primary production (Barange et al., 2010). These ecosystems contain 41% of the world's known fish species. Marine ecosystems cover approximately 71% of the Earth's surface, contain approximately 97% of the planet's water. They generate 32% of the world's net
primary production Food is a substance consumed to provide nutritional support for the body. It is a material consisting protein, carbohydrate, vitamin, fibre, water, and fat used by an organism to provide energy, sustain growth, repair and enhance vital body processes (Food, 2015). Diet is an important factor essential for the wellbeing and good health of individuals. In recent times, emphases have been made on eating well and eating good food due to its economic consequence. Some of these foods are rendered unsuitable for human consumption by pathogenic microorganism. Pathogenic microorganisms are defined as those microorganisms that may cause illness in humans. Some pathogenic microorganisms are transmitted to humans through food (FAO, 2003).
There is an increasing world demand for seafood because of its nutritive content which is beneficial for people of all age group including the young and the old. However, the increasing demands for seafood have led to increase in illness resulting from the consumption of seafood (Borresen, 2008), for instance, disease caused by Vibrio spp., Salmonella spp., Listeria monocytogenes and Escherichia coli have been reported previously (Graves et al., 2010; Food and Agriculture Organisation, 2014). Foodborne pathogens from seafood origin have gained prominence and are a threat to public health. The survival of foodborne pathogens under different environmental conditions demand the development and use of efficient and reliable isolation, detection, differentiation, classification and/or typing techniques for their surveillance (Ajayi and Omoya, 2017). During research and study of diversity, it is important to identify unknown microorganism, the identity of pathogenic bacteria and fungi must be unveiled and known. Primary identification of bacteria in the laboratory is based on phenotypic characteristics, some simple tests such as morphology, growth on various types of culture media and biochemical tests are carried out (Brindha and Ani, 2012). These tests are widely used, are cheaper, detect only viable bacteria, and show isolates that can further be characterized and studied. However, they are slow, laborious, less efficient and less accurate (Adzitey et al., 2011).
Traditional microbiological methods are limited and are not precise enough in the quest for the study of the entire microbial diversity. The use of molecular techniques in the characterization and identification of bacteria and other microorganism has many advantages (Brindha and Ani, 2012). Molecular techniques have also been more widely used in the surveillance and genetic studies of foodborne pathogens to increase knowledge and understanding on primary source of foodborne pathogens and its genetic diversity (Ajayi and Omoya, 2017). Molecular techniques are widely embraced because, they are rapid, less laborious, specific and efficient, and are more sensitive and accurate compared to the conventional method (Borresen, 2008; Magistrado et al., 2001; Keramas et al., 2004).
The high nutritious content of seafood which has been reported has increased the demand and consumption of sea foods (Adegoke et al., 2010). However, some pathogenic microorganisms of public health importance are present in these foods. Thus it is important to provide information on the pathogenic microorganism of public health importance that may cause illness from the consumption of seafood from Igbokoda sea water and provide an effective treatment method using traditional salting method applied at specified temperature and time which have not been previously considered.
Sample location
Igbokoda is the seat of Ilaje Local Government Area in Ondo State, Nigeria. Its coordinates are 6°19’0’’N and 4°49’0’’E in Degrees Minutes Seconds (DMS). The people are of Yoruba ethnic group and recently comprising of the Ikale’s, Apoi, Arogbo Ijaws, Itsekiri, the Aheri and Etikan. Ilaje land is blessed with petroleum, glass sand, salt, Tar sand/Bitumen, quartz and clay. Traditional occupational activities include fishing, canoe making, lumbering, net making, mat making, launch building, farming and trading yielding agricultural products such as Fish, Poultry, piggery, Maize, Palm oil, Vegetables, Timber, Rafia, Poultry, Copra, cocoyam, Bananas and Cassava. The Ilaje land has one of the longest coastal lines in Nigeria and also, the largest deep sea making it suitable for the collection of sea food samples. Samples were collected between August 2016 and October 2016.
Sample collection
Samples were collected at Ugbo-Nla Sea in Igbokoda. Fresh seafood were aseptically picked at random using sterile gloves and placed into sterilized containers, then placed in an iced pack. They were transported to microbiology research laboratory of Federal University of Technology, Akure within 4-5 h of collection for microbiological analyses. A total of ninety seven snail and one hundred and six shrimp samples were collected once in a week at two weeks interval for six weeks from Atlantic line at Ugbo nla, Igbokoda.
Isolation and identification of microorganism from selected sea foods
Sample preparation was made modifying the method described by Eze et al. (2011). The samples were disserted with a sterile knife. The cut samples were crushed into small pieces in a sterile mortar with about 10 ml sterile water. From the crushed sample, 1 ml aliquot volume was measured out and homogenized in a clean, dry sterile beaker containing 9 ml of distilled water giving a 1:10 dilution. 1.0 ml each of dilution 10-5 - 10-10 were pour plated on Nutrient agar, Potato dextrose agar and some selective and differential media (Salmonella Shigella agar, Eosin Methylene Blue agar, MacConkey agar and Manitol Salt agar), the plates were inverted and incubated aerobically at 37°C for 24 h for bacteria and 25°C for fungi, after which the plates were examined for growth. Bacterial isolates were identified with Microbact 24E identification kit following the manufacture standard procedure while fungi were identified using cultural and microscopic characterization as described by Cheesbrough (2006).
Molecular identification of bacteria and fungi isolated from selected sea food
This test was carried out according to the method of Nicole et al. (2004), as follow:
(i) Genomic DNA extraction of bacteria and fungi
A 1.5 ml of broth culture was taken in centrifuge tube. Centrifuged at 10,000 rpm for 2 min and supernatant was discarded. To the pellet 1 ml of distilled water was added and dissolved the pellet completely. Again centrifuged at 10,000 rpm for 2 min, the procedure was repeated for two times. The supernatant was discarded and to the pellet 100 µl of Tris EDTA buffer was added and dissolve the pellet completely in buffer. The supernatant containing the DNA were transferred to another tube and stored at -20°C. The concentration and purity of the extracted DNA was estimated using a Nanodrop spectrophotometer (Model: 752).
(ii) Polymerase chain reaction (PCR) amplification and purification of 16S rRNA and 18S rRNA gene
Protocol for bacteria PCR: The 16S rRNA gene was amplified using primers 5’AGAGTTTGAT CCTGGCTCAG 3’ and 5’GACGGGCRGTGWGTR CA 3’ forward and reverse respectively. PCR mix contained 10X buffer, 100 mM dNTPs, 2.52 M MgCl2, 2UTaqDNA polymerase, 1 μl of each (forward and reverse) primers, 2 μl of genomic DNA and sterilized distilled water to make a final volume of 25 ml. TaqDNA polymerase initiates replication of DNA fragment by using nucleotide bases from dNTPs mixture (A, C, G, T). The PCR reaction included the following steps; initial denaturation of 2 min at 94°C (Pre heating) followed by 25 cycles were run on a thermal cycler, each comprising 1 min at 94°C (denaturation), 1 min at 94°C (annealing) and 1.5min at 94°C (extension), followed by a final extension of 10 min at 94°C for utilization of extra dNTPs in the PCR mixture.
Protocol for fungi PCR: The 18S rRNA gene was amplified using primers 5’-TCCGTAGGTGAACCTGCGG-3’ and 5’-TCCTCCGCTTATTGATATGC-3’ forward and reverse respectively. The PCR Master Mix contained 4 μl of PCR buffer, 2 μl each of universal primers, 5 μl of dNTPs, and 2 μl of Taq DNA polymerase. The 20 μl PCR mixtures were prepared by adding 5 μl of extracted fungal DNA to Master Mix. In the initialization step, the mixtures were heated at 94°C for 5 min. The fungal DNA was then amplified in 30 cycles of 94°C for 30 s for denaturation, 55°C for 45 s for annealing, and 72°C for 1 min for extension; and final elongation at 72°C for 5 min in an automated thermal cycler.
(iii) Agarose gel electrophoresis
Agarose gel electrophoresis protocol for bacteria PCR product: A 0.8 g of agarose was weighted and taken in a 100 ml reagent bottle. To this 100 ml of 1X TBE buffer was added and it was heated in microwave oven till agarose melts. The agarose solution was then poured in a gel-casting unit assembled with appropriate comb and it was allowed to get polymerize. When the agarose polymerized, the comb was removed and the gel was kept in electrophoresis tank consisting of 1X TBE buffer. About 20 µl of isolated bacterial genomic DNA from PCR product was mixed with 20 µl of loading dye (bromophenol blue) and it was loaded in 0.8% agarose gel. The gel was electrophoresed at 100 volts for about 30 min and it was observed on UV transilluminator.
Agarose gel electrophoresis protocol for bacteria PCR product: A 20 μl of each mixed PCR product with loading dye was used to perform electrophoresis in 1.5% (w/v) agarose gel, with 5 μl 1Kb DNA Ladder as a molecular marker in parallel. The gel was electrophoresed at 100 volts for about 30 min and it was observed on UV transilluminator.
(iv) 16S rRNA and 18S rRNA gene sequence, BLASTn and phylogenetic analysis
Identification of strain was done by sequencing the PCR products of 16S rRNA (bacteria) and 18S rRNA (fungi) using sequencer, determined sequences were compared with sequences available in GeneBank, derived sequence aligned by Basic Local Alignment Search Tool (BLAST) algorithm, the highest S-ab value with identified species in the Sequence match search. Using the results received through BLASTn a phylogenetic tree is created using the BLASTn web-page.
Determination of effects of salt concentrations and temperature on microorganisms isolated from selected sea foods
Alkaline peptone water solution was prepared as described by Cheesbrough (2006) and 24 h broth culture of test organism was also prepared, the turbidity was standardized to 0.5 MacFalad turbidity standards.
(i) Salt (NaCl) (AnalaRBDH) was prepared in different concentrations, 1.0 g of salt was completely dissolved in 99.0 ml of sterile distilled water to make concentration of 1% salt solution, 1.0 g of peptone was added and dissolved. The solution was sterilized at 121°C for 15 min. The procedure was repeated till concentration of 10% salt solution was archived. The concentration of salt solution used are; 1, 2, 3, 4, 5, 6, 7, 8, 9 and 10%. Ten milliliter of alkaline peptone solution (1% salt concentration) was dispensed into a sterilized test tube, 100 µl of the standardized test microorganism was dispensed into the same tube and incubated. This was repeated for salt concentration 2, 3, 4, 5, 6, 7, 8, 9 and 10%.
(ii) Temperature: The set up above were incubated at different temperature of 4°C (refrigerated temperature), 25°C (room temperature), 37°C (body temperature), 40oC (slightly elevated temperature) and 45°C (elevated temperature). Bacterial and fungal isolates were incubated at these temperatures for 24 and 72 h respectively. After the period of incubation, the growth turbidity was observed using a spectrophotometer at 640 nm. Inoculated tube with 0.0% salt concentration and tubes with 1 to 10% salt concentration without inoculation were used as control. The temperature and salt concentration at which little or no turbidly was observed are noted.
(iii) Time kill assay: The most effective temperature and salt concentration for each microorganism observed in the previous assay were used, the solution was prepared again, inoculated and incubated at the respective most effective temperature and salt concentration for 24 h. Microbial load were checked using pour plate method (Fawole and Oso, 2004) just after inoculation (0 h), 6, 12, 18 and 24 h after inoculation. For bacterial isolates, 1.0 ml of the sample was incubated at 37°C for 24 h and number of colony was noted while fungal isolates were incubated at 27°C for 72 h, number of fungi spores was also noted. The time at which zero growth was recorded is taken as time kill.
Data analysis
Data was statistically analysed using SPSS version 20, percentage resistance was separated using new Duncan’s Multiple Range test and significant difference was value at Æ¿≤ 0.05, test of significance for resistant, descriptive statistics was used to present the result of salt concentration and incubation time.
Total viable bacterial count of selected sea foods collected from Ugbo nla, Igbokoda
Mean total bacterial viable counts are represented in Figure 1. For sea water, there was no significant difference (p≤0.05) between the total bacterial counts at week 2 (30.33 cfu/100 ml), 3 (34.00 cfu/100 ml), 4 (29.33 cfu/100 ml), 5 (31.33 cfu/100 ml) and 6 (33.00 cfu/100 ml) while the bacterial load at week 1 (36.67 cfu/100 ml) was significantly higher (p≤0.05) than other weeks. In shrimp, there were no significant differences (p≤0.05) between the total bacterial counts in all the weeks. However, the bacteria load of the shrimp showed a range of 2.77 ± 0.23 ×106 cfu/g to 2.10 ± 0.06 × 106 cfu/g. In snail, there were no significant differences (p≤0.05) between the total bacterial counts in all the weeks. However, the bacteria load of the snail showed a range of 4.63 ± 0.88 ×106 cfu/g to 3.97 ± 0.33 × 106 cfu/g. comparatively, total bacteria load observed in snail was higher than shrimp and water.
Total coliform counts of selected sea foods collected from Ugbo nla, Igbokoda
Mean coliform counts of sea samples are illustrated in Figure 2. It was observed in sea water that there were no significant difference (p≤0.05) between the total coliform counts at week 1 (11.00 cfu/100 ml), 2 (12.00 cfu/100 ml), 3 (10.67 cfu/100 ml), 4 (13.00 cfu/100 ml) and 5 (10.00 cfu/100 ml) while the count at week 6 (13.67 cfu/100 ml) is significantly higher (p≤0.05) than other weeks while in shrimp, there was no significant difference (p≤0.05) between the total coliform counts at week 2 (6.33 ×104 cfu/g), 3 (5.00 ×104 cfu/g), 4 (5.00 ×104 cfu/g), 5 (6.00 ×104 cfu/g) and 6 (5.00 ×104 cfu/g), while the coliform count at week 1 (7.67 ×104 cfu/g) is significantly higher (p≤0.05) than other weeks.
Also, it was noted in snail that there was no significant difference (p≤0.05) between the total coliform counts at week 2 (12.00 ×104 cfu/g), 4 (13.33 cfu/g) and 5 (11.33 ×104 cfu/g). Also, the counts at week 3 (15.33 ×104 cfu/g), 4 (13.33 ×104 cfu/g), and 6 (14.67 ×104 cfu/g) showed no significant difference (p≤0.05). The coliform counts at week 1 (16.33 ×104 cfu/g) is significantly higher (p≤0.05) than other weeks. Comparatively, total coliform counts recorded in snail were generally higher than what was obtained in sea water and shrimp.
Total fungal counts of selected sea foods obtained in Ugbo nla, Igbokoda
Mean fungal counts of sea water samples are reveled in Figure 3. The result revealed that in sea water samples, there were no significant difference (p≤0.05) between the total fungal counts at week 1 (0.00 sfu/100 ml), 2 (0.33 sfu/100 ml), 3 (0.00 sfu/100 ml), 4 (0.00 sfu/100 ml), 5 (0.00 sfu/100 ml) and 6 (0.33 sfu/100 ml).
It was observed in shrimp that there was no significant difference (p≤0.05) between the total fungal counts at week 1 to 6. The fungal load of the shrimp showed a range of 3.00 ± 0.58 to 5.00 ± 0.58 × 102 sfu/g. Also, in snail, there was no significant difference (p≤0.05) between the total fungal counts at week 1 to 6. The fungal load of the snail ranged from 2.00 ± 1.00 to 2.67 ± 0.33 ×102 sfu/g. Fungal plate counts observed in shrimp were generally higher than what was recorded in sea water and snail.
Molecular identification of bacteria isolated from selected sea foods
Molecular identification of bacterial isolates from seafood is presented in Table 1. The length of amplified PCR products was 1500 base pair. The sequence obtained was blasted in National Centre for Biotechnology Information (NCBI) database. Based on the 16s rRNA sequences, the following bacterium were confirmed Proteus mirabilis LZPM-03, Klebsiella pneumoniae 2N3, Serratia marcescens, Escherichia coli 2A, Pantoea agglomerans BJCP2, Pseudomonas aeruginosa R8-589, Alcaligenes faecalis ACBC1, Yersinia pseudotubercules YPIII, Listeria monocytogen J1776, Staphylococcus aureus, Lysinibacillus sphaericus C3-41, and Proteus mirabilis LCX20. The results were compared with biochemical identification and it was noted that B. subtilis, P. rettgeri and A. iwoffii were identified as L. sphaericus, P. mirabilis and Alcaligenes faecalis respectively using molecular methods and however, there was no change in the identity of other isolates.
Molecular identification of fungal isolates
In Table 2, result showed the identity of the fungal isolates. Fusarium proliferatum FproStRINI, Aspergillus peniciloides, Aspergillus flavus NRRL3357 and Aspergillus oryzae RIB40 were identified. The molecular results were compared with cultural identification and it was noted that F. culmorum and Aspergillus fumigatus were identified as F. proliferatum FproStRINI and A oryzae RIB40 respectively. However, A. peniciloides and A. flavus remained A. peniciloides and A. flavus NRRL3357 respectively.
Effects of different salt concentration on isolated bacteria at refrigerated temperature
The effects of salt concentration on isolated bacteria were illustrated in Figure 4. The most effective salt concentration that totally inhibited the growth of each bacteria isolated were; P. mirabilis (9%), Klebsiella oxytoca (8%), Enterobacter agglomerans (9%), Pseudomonas aeruginosa (9%), Klebsiella pneumoniae (8%), Serratia marcescens (9%), Acenetobacter iwoffii (8%), Proteus vulgaris (8%), Yesinia psudotubercules (9%), Escherichia coli (10%), Escherichia coli-inactive (9%), Citrobacter freundi (8%), Bacillus subtilis (9%), Bacillus thuringensis (9%), Listeria monocytogen (10%), S. aureus (9%) and Providencia rettgeri (9%).
Effects of different salt concentration on isolated bacteria at room temperature
The effects of salt concentration on isolated bacteria were revealed in Figure 5. The most effective salt concentration that totally inhibited the growth of each bacteria isolated were; P. mirabilis 7% (0.008 nm), K. oxytoca (7%), E. agglomerans (6%), P. aeruginosa (7%), K. pneumoniae (7%), S. marcescens (7%), A. iwoffii (5%), P. vulgaris (6%), Y. psudotubercules (6%), E. coli (7%), E. coli-inactive (7%), C. freundi (5%), B. subtilis (6%), B. thuringensis (7%), L. monocytogen (6%), S. aureus (7%) and P. rettgeri (6%).
Effect of different salt concentration on isolated bacteria at 37°C
The effects of salt concentration on isolated bacteria were shown in Figure 6. The result showed that the most effective salt concentration that totally inhibited the growth of P. mirabilis, K. oxytoca, E. agglomerans, P. aeruginosa, K. pneumoniae, S. marcescens, A. iwoffii, P. vulgaris, Y. psudotubercules, E. coli, E. coli-inactive, C. freundi, B. subtilis, B. thuringensis, L. monocytogen, S. aureus, and P. rettgeri were 8, 6, 6, 6, 7, 7, 6, 7, 7, 7, 6, 6, 6, 6, 7, 7, and 8% salt concentration respectively.
Effect of different salt concentration on isolated bacteria at 40°C
The effect of salt concentration on bacteria isolates were illustrated in Figure 7. The result revealed that most effective salt concentration that totally inhibited the growth of all bacterial isolates was 5% salt concentration.
Effect of different salt concentration on isolated bacteria at 45°C
The effect of salt concentration on bacteria isolates were illustrated in Figure 8. The most effective salt concentration that totally inhibited the growth of bacterial isolates was 4% salt concentration except of B. subtilis and B. thuringensis which were totally inhibited at 5% salt concentration.
Effect of different salt concentration on isolated fungi at refrigerated temperature, room temperature, 37, 40 and 45°C
Figures 9 to 13 revealed the effect of salt concentration on the isolated fungi; Fusarium culmorum, Aspergillus flavus, Aspergillus peniciloides and Aspergillus fumigatus at refrigerated temperature, room temperature, 37, 40 and 45°C respectively. The salt concentrations from 0% to 10% were not effective on all the fugal isolates at different temperature. However, the growth of Fusarium culmorum, Aspergillus flavus, Aspergillus peniciloides and Aspergillus fumigatus were inhibited by 8% salt concentration at 45°C.
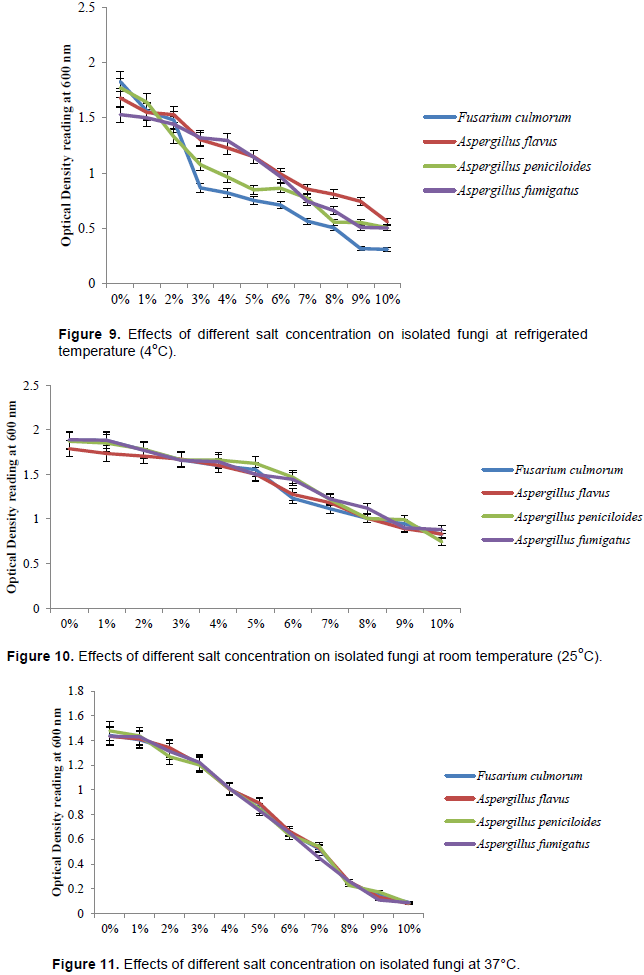
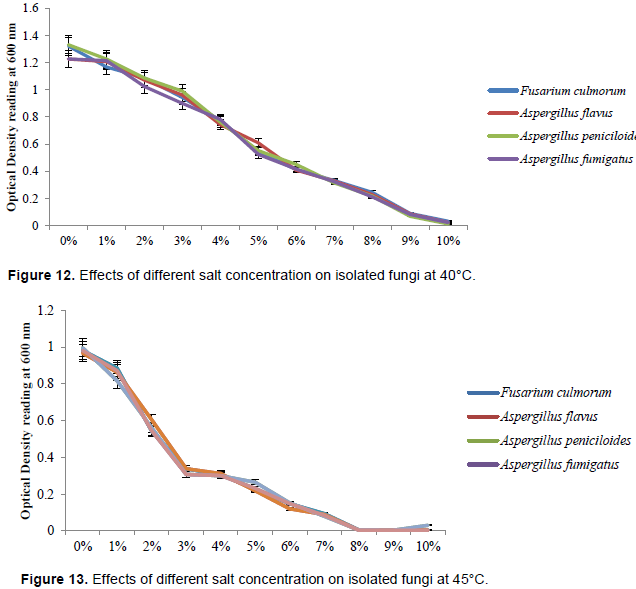
Effects of incubation time on bacterial isolates at 45°C using 4% salt concentration
Figure 14 illustrates the effect of incubation time on the bacterial isolates at 45°C using 4% concentration. The respective bacterial load at 0, 6, 12, 18 and 24 h cfu/ml × 103 of the isolates are Proteus mirabilis (74, 8, 0, 0, 0), Klebsiella oxytoca (82, 7, 0, 0, 0), Enterobacter agglomerans (93, 6, 0, 0, 0), Pseudomonas aeruginosa (77, 4, 0, 0, 0), Klebsiella pneumoniae (76, 6, 0, 0, 0), Serratia marcescens (83, 5, 0, 0, 0), Acenetobacter iwoffii (87, 8, 0, 0, 0), Proteus vulgaris (71, 4, 0, 0, 0), Yesinia psudotubercules (83, 5, 0, 0, 0), E. coli (81, 7, 0, 0, 0), E. coli-inactive (92, 7, 0, 0, 0), Citrobacter freundi (87, 5, 0, 0, 0), Bacillus subtilis (84, 8, 2, 0, 0), B. thuringensis (76, 8, 3, 0, 0), Listeria monocytogen (74, 7, 0, 0, 0),Staphylococcus aureus (82, 6, 0, 0, 0), and Providencia rettgeri (85, 5, 0, 0, 0). There was no growth recorded in 18 and 24 h incubation time in all the bacteria isolate.
Effects of incubation time on fungal isolates at 45°C using 8% salt concentration
The effect of incubation time on fungal isolates at 45°C using 8% salt concentration was revealed in Figure 15. The respective fungal load at 0, 6, 12, 18 and 24 h sfu/ml × 102 of the isolates is Fusarium culmorum (8, 0, 0, 0, 0), Aspergillus flavus (11, 0, 0, 0, 0), Aspergillus peniciloides (13, 0, 0, 0, 0) and A. fumigatus (16, 2, 0, 0, 0). There was no fungal growth at 6 h with the exception of A. fumigatus, 12, 18 and 24 h.
This study revealed that seafood habours bacteria and fungi. There were differences in the microbial load of sea water, shrimp and snail, the total viable bacterial counts was higher in sea water while total coliform counts was higher in snail, also the highest fungal counts was obtained in shrimp samples. High microbial counts observed in this study corroborate the result of Adebayo-Tayo et al. (2011), Dabadé (2015), Edun et al. (2016) and Kaumadi and Sriyanie (2017).
The high microbial load observed in sea water as observed in this study may be due to pollution. Pollution and contamination of sea water has increased in recent years due to improper garbage disposal, discharge of untreated domestic sewage, animal waste, industrial waste into sea water and or pollution that are brought by rain water and river water into the sea water (Kaumadi and Sriyanie, 2017). Snails burrow more into the sand or soil seeking for calcium to get a thicker shell, in the process of burrowing, it diffuse more of fecal contaminant which has sediment in the floor while feeding, this may account for the high coliform count observed in snail in this study (Siliman and Newel, 2003). Snails have been found to live mutually with fungi, the interaction can be traced to the fungal load found in snail. Also, the interaction between intestinal microbiota and snail contribute to it ability to feed and persist in its environment (Van Horn et al., 2012). It will be important to determine what role these microorganisms played in snail immunology.
Observation from this study revealed the fungal load of shrimp exceeded that of snail, this may be due to the feeding habit of shrimp. While feeding, shrimp assimilate fungi as part of its diet (Siliman and Newel, 2003). Due to the size of snail as a filter feeder, it may have filter out some fungi while feeding to a level that will not be detrimental to its wellbeing. The high microbial load observed in shrimp could aslo be that these microorganisms are in mutual relationship with shrimp, further studies will be needed to validate this claim. The term seafood is extended to freshwater organisms consumed by humans, all edible aquatic life may be referred to as seafood, and therefore the significance of polluted rivers cannot be neglected (FAO, 2015). The Niger Delta region which Igbokoda is located has a history of heavy pollution from industrial effluent and other pollutant from human activities. The rivers are sometimes linked to each other (with a preceding runoff into rivers) resulting to exchange of pollutant and microbial communities (Edun et al., 2016). These microbes often find surfaces or organs of aquatic organisms for colonization.
Recreational activities and religious activities along Ugbo nla, Igbokoda coast may be another source of high microbial load. The activities include fun bathing, spiritual bathing with materials such as soaps and throwing of foreign materials into the water body. Environmental factor such as rainfall accompanied with strong wind have also been contributing to increased microbial load in seawater and other water bodies. Kaumadi and Sriyanie (2017) reported that increased rainfall during wet season (sample collection period) which would have increased the surface runoffs, urban storm water overflows and/or re-suspension of sediments might have increased the contaminants in the seawater and subsequently aquatic organisms. Therefore, microbial contamination on environmental surfaces may be transferred to the aquatic organism/ food products directly through surface contact and air movements (Pal et al., 2016). Favourable physical and chemical conditions such as NO3-, S042, pH and temperature occasioned by allochthonus materials from the sampling area of the coast during the season increases the microbial population due to the stimulatory effect of additional carbon and energy sources in the form of effluents and sometimes crude oil (Edun et al., 2016).
Sea and fresh waters are endowed with highly diversified aquatic resources which serve as food for human (Dabadé, 2015). Seafood is a rich source of protein, vitamins and minerals, their flesh pH is around neutral (pH 7). These properties make seafood a suitable living and proliferation place for microorganism (Colakoglu et al., 2006). The result of this work revealed that several microorganism were present in the selected seafood and seawater sample in Ugbo nla, Igbokoda and they are Proteus mirabilis, Klebsiella oxytoca, Enterobacter agglomerans, Pseudomonas aeruginosa, Klebsiella pneumoniae, Serratia marcescens, Acenetobacter iwoffii, Proteus vulgaris, Yersinia pseudotubercules, E. coli, E. coli-inactive, Citrobacter freundi, B. subtilis, Bacillus thuringensis, Listeria monocytogen, Staphylococcus aureus, Providencia rettgeri, Fusaruim culmorum, Aspergillus flavus, Aspergillus peniciloides and Aspergillus fumigatus. These isolated microorganisms were also reported by Colakoglu et al. (2006), Adebayo-Tayo et al. (2011), Balfour et al. (2014), Bassey et al. (2014), Dabadé (2015), Edun et al. (2016) and Kaumadi and Sriyanie (2017).
Seafood can be a source of various food borne diseases, it acts as a vehicle for all important species of foodborne pathogens. They may be contaminated with food borne pathogens which are naturally present in aquatic environments (Dib et al., 2014). These pathogenic organisms have been implicated in outbreaks of food-borne diseases in many parts of the world (Adebayo-Tayo et al., 2011). It has been found that these pathogens has the ability to cause many health problems such as gastrointestinal, respiratory, skin, eye, nasal cavity and ear infections. Edun et al. (2016) reported that the bacteria Salmonella spp has been found to survive and persist in the aquatic environment. It has been detected in seafood harvested in different creeks in Niger Delta and causes new born meningitis and infantile diarrhoea. Adebayo-Tayo et al. (2011) also reported that E. coli isolated from snail was found to be the causative agent of diarrhoea, dysentery, haemolytic uremic syndrome, bladder and kidney infection, septicaemia, pneumonia and meningitis.
The high prevalence of Enterobacteriaceae (Proteus mirabilis, K. oxytoca, Enterobacter agglomerans, Klebsiella pneumoniae, Serratia marcescens, Proteus vulgaris, Yesinia psudotubercules and E. coli) in this study suggest that there is high contamination of faecal effluent through runoff from rivers and other sources such as direct disposal of human, animal and domestic waste. This was in agreement with the report of Dib et al. (2014) which account for high faecal contamination of microbiologically analyzed seafood. The presence and high occurrence of Aspergillus spp reveals possible production of aflatoxins in the samples. There were variations in the rate and occurrence of bacteria and fungi in the samples with seawater sample recording the lowest percentage occurrence while the seafood have higher percentage occurrence of both bacteria and fungi. This might result from the self-cleansing properties of the water and the filtering ability of sea organisms (Edun et al., 2016). The sea organisms are filter feeders, that is, they are capable of accumulating microorganisms in high concentrations. These organisms filter the surrounding water and consequently assimilate all the contaminants present in these environments.
Consumers of these sea foods are therefore exposed to microbial pollutants in the ecosystem. Worldwide, molecular approaches have emerged in environmental microbiology practices, molecular techniques have also been widely used in surveillance, mutation and other genetic studies of foodborne pathogens to increase our understanding into the primary source of foodborne pathogens, source of infection and genetic diversity. Molecular techniques have the advantage that, they are rapid, less laborious, and more sensitive, specific and efficient compared to the conventional method (Magistrado et al. 2001; Keramas et al., 2004). Molecular method also provides insights into etiologies of infectious disease (Woo et al., 2008) and appropriate antibiotic treatment (Harris et al., 2002). PCR revealed that the molecular weight of the genomic DNA of sequenced bacteria is 1500bp. According to the 16S and 18S rRNA respective analyses, bacterial and fungal isolates showed more than 70% similarity in the NCBI GenBank by BLAST. Based on the BLASTn results the isolates confirmed were twelve bacteria and four fungi. The bacteria were Proteus mirabilis LZPM-03, Klebsiella pneumoniae 2N3, Serratia marcescens, Escherichia coli 2A, Pantoea agglomerans BJCP2, Pseudomonas aeruginosa R8-589, Alcaligenes faecalis ACBC1, Yersinia. pseudotubercules YPIII, Listeria monocytogen J1776, Staphylococcus aureus Lysinibacillus sphaericus C3-41, and Proteus mirabilis LCX20 while the fungi are Fusarium proliferatum FproStRINI, Aspergillus peniciliodes, Aspergillus flavus NRRL3357 and Aspergillus oryzae RIB40.
Salting is a traditional technique that has been used regularly for the improvement and storage of fish and seafood (Pal et al., 2016), temperature has strong inhibitory effect on microbial growth rate (Membre and Burlot, 1994). Since technology can be defined as a method and process developed by people to enable them to provide for their daily needs, seafood vendors in Igbokoda usually use salt to prolong the shelf life of seafood due to the epileptic power supply experienced in Igbokoda and total blackout for over a year but there is death of information on appropriate concentration that will be enough for the inhibition of microbial growth. The result from this study showed high concentration of salt (8-10%) is required to inhibit growth of the bacterial isolate at refrigerated temperature (4°C), this was in agreement with the study of Zaika et al. (1989) who reported that low temperatures and high sodium chloride concentrations interacted strongly to decrease growth rates of Shigella flexneri at all pH values studied. However, salt concentration of 6-7% was the most effective concentration against the bacterial isolates at room temperature of 25°C and biological temperature of 37°C. Salt concentration of 5% at 40°C and 4% at 45°C were the most effective concentration and temperature against the bacterial isolates. This implies that the salt concentration required to inhibit bacterial growth reduces with increase in temperature. The most effective salt concentration and temperature in this study was 4% concentration at 45°C, it has the lowest bacterial cell population (optical density). Result of the inhibition of salt on fungal isolates were different, fungi generally have higher salt tolerance and can withstand harsh environmental condition than bacteria (Zajc et al., 2012). Eight percent salt concentration at 45°C was effective in the inhibition of the fungal isolates, higher salt concentrations increase its osmolarity, which is likely to have resulted in hyper osmotic shock to cells causing growth suppression (Abdulkarim et al., 2009). The rate of killing of the most effective concentration and temperature showed that at 12 h, bacterial growth will have been killed completely and by 6 h, fungal growth will have been totally inhibited. To avoid the spreading of these bacterial pathogens and toxin producing fungi from being spread in food chain, sea foods can be preserved by 4% salt at 45°C.
The study revealed that sea foods and seawater from Ugbo nla, Igbokoda water in Niger Delta Area of Ondo state, Nigeria were heavily contaminated with micro-organisms. Although, there are no much surveillance data on foodborne disease of public health importance in Nigeria, especially those that arose from environmental contamination from the source, this study confirmed the suspicion and assertions of some indigenous researchers that laboratory studies will reveal the presence of foodborne pathogens in sea foods. The sea food and seawater (habitat) habours different bacteria and fungi, K. oxytoca and A. flavus were the most prevalent respective bacteria and fungi isolated respectively.
The molecular method of identification was found to be advantageous as bacteria and fungi were identified to strain level. Thus, there is an urgent need to mount an intensive public health education on foodborne illnesses arising from raw seafood consumption in Nigeria. Traditional method of salting to preserve seafood can inhibit microorganisms as revealed in this study; 4% salt concentration at 45°C to inhibited bacteria and 8% salt concentration at 45°C inhibited fungi at 18 and 12 h respectively.
The authors have not declared any conflict of interests.
REFERENCES
Abdulkarim SM, Fatimah AB, Anderson JG (2009). Effect of salt concentrations on the growth of heat-stressed and unstressed Escherichia coli. Journal of Food Agriculture and Environment 7(3-4):51-54.
|
|
Adebayo-Tayo BC, Onilude AA, Etuk FI (2011). Studies on Microbiological, Proximate Mineral and Heavy Metal Composition of Freshwater Snails from Niger Delta Creek in Nigeria. Assumption University Journal of Technology 14(4):290-298.
|
|
|
Adegoke AA, Adebayo-Tayo CB, Inyang UC, Aiyegoro AO, Komolafe OA (2010). Snails as meat source: Epidemiological and nutritional perspectives. Journal of Microbiology and Antimicrobials 2(1):1-5.
|
|
|
Adzitey F, Huda N, Gulam R (2011). Comparison of media for the isolation of Salmonella (XLD and Rambach) and Listeria species (ALOA and Palcam) in naturally contaminated duck samples. International Journal of Food Safety 13:20-25.
|
|
|
Ajayi KO, Omoya FO (2017). Molecular Identification of Selected Multiple Antibiotic Resistance Bacteria Isolated from Poultry Droppings in Akure, Nigeria. Biochemistry and Molecular Biology 2(1):6-11.
Crossref
|
|
|
Balfour ST, Badrie N, Chang YI, Chatergoon L (2014). Microbiological, physical and sensory quality of marine shrimp (Peneaus spp.) sold by vendors in Trinidad, West Indies. International Food Research Journal 21(4):1279-1288.
|
|
|
Barange M, Field J, Harris R, Eileen E, Hofmann E, Perry R, Werner F (2010). Marine Ecosystems and Global Change. Oxford University Press.
Crossref
|
|
|
Bassey SC, Ofem OE, Essien NM, Eteng MU (2014). Comparative Microbial Evaluation of Two Edible Seafood Pomacea palludosa (Apple Snail) and Ergeria radiata (Clam) to ascertain their Consumption Safety. Journal of Nutrition and Food Science 4:328.
|
|
|
Borresen T (2008). In Improving Seafood Products for the Consumer. Woodhead Publishing Series in Food Science, Technology and Nutrition No. 158. Woodhead Publishing Limited.
Crossref
|
|
|
Brindha V, Ani AM (2012). Molecular characterization and identification of unknown bacteria from waste water. Indian Journal of Innovations and Development 1(2):87-91.
|
|
|
Cheesbrough M (2006). District Laboratory Practice in Tropical Countries. 2nd Edition. Cambridge University Press, Cambridge, UK. ISBN-13:9781139449298. 50:165-176.
Crossref
|
|
|
Colakoglu FA, Ozen O, Cakir F (2006). Microbiological Quality of Seafood in the Dardanelles, Turkey. Journal of Biological Science 9(3):425-427.
Crossref
|
|
|
Dabadé SD (2015). Shrimp quality and safety management along the supply chain in Benin. PhD thesis, Wageningen University, Wageningen, pp. 1-158.
|
|
|
Dib AL, Lakhdara N, Rodriguez EE, Kabouia R, Roldán ME, García ME, Hafida KH, Guerraichi L, Bouaziz O (2014). Prevalence of microbial contamination of fresh seafood product sold in Constantine, Algeria. Environmental Skeptics and Critics 3(4):83-87.
|
|
|
Edun OM, Akinrotimi OA, Makinde OO (2016). Seasonal Changes of Microbial Load in Some Sea Foods from Buguma and Ekerekana Creeks, Niger Delta, Nigeria. Peertechz Journal of Environmental Science and Toxicology 1(1):001-007.
Crossref
|
|
|
Eze EI, Echezona BC, Uzodinma EC (2011). Isolation and identification of pathogenic bacteria associated with frozen mackerel fish (Scomber scombrus) in a humid tropical environment. African Journal of Agricultural Research 6(8):1947-1951.
|
|
|
FAO/WHO (2003). Risk Assessment of Campylobacter spp. in broiler chickens and Vibrio spp. in seafood. FAO Food and Nutrition Paper 75:57.
|
|
|
Fawole MO, Oso BA (2004) Characterization of Bacteria: Laboratory Manual of Microbiology. 4th Edition, Spectrum Book Ltd., Ibadan, Nigeria, pp. 24-33.
|
|
|
Food (2015). In Merriam- Webster.com. Retrieved August 16, 2015 from
View
|
|
|
Food and Agriculture Organisation of the United Nations (FAO) (2014). Assessment and Management of Seafood Safety and Quality. In Food and Agriculture Organization of the United Nations, Rome, Italy. FAO Fisheries Technical Paper 574.
|
|
|
Food and Agriculture Organisation of the United Nations (FAO) (2015). Fishery Fact Sheets Collections. ASFIS List of Species for Fishery Statistics Purposes. In Fisheries and Aquaculture Department.
|
|
|
Graves LM, Helsel LO, Steigerwalt AG, Morey RE, Daneshvar MI, Roof SE, Orsi RH, Fortes ED, Milillo SR, den Bakker HC, Wiedmann M, Swaminathan B, Sauders BD (2010). Listeria marthii sp. nov., isolated from the natural environment, Finger Lakes National Forest. International Journal of Systematic and Evolutionary Microbiology 60:1280-1288.
Crossref
|
|
|
Harris KA, Fidler KJ, Hartley JC, Vogt J, Klein NJ, Monsell F and Novelli VM (2002). Unique case of Helicobacter sp. osteomyelitis in an immunocompetent child diagnosed by broad-range 16S PCR. Journal of Clinical Microbiology 40:3100-3103.
Crossref
|
|
|
Kaumadi S, Sriyanie IA (2017). Microbiology of Seawater and Sand in a Selected Bathing Site of Sri Lanka - A Study towards Microbial Quality Assessment. Frontiers in Environmental Microbiology 3(1):9-18.
Crossref
|
|
|
Keramas G, Bang DD, Lund M, Madsen M, Bunkenborg H, Telleman P, Christensen CBV (2004). Use of culture, PCR analysis and DNA microarrays for detection of Campylobacter jejuni and Campylobacter coli from chicken faeces. Journal of Clinical Microbiology 47:3985-3991.
Crossref
|
|
|
Magistrado P, Carcia M, Raymundo A (2001). Isolation and polymerase chain reaction-base detection of Campylobacter jejuni and Campylobacter coli from poultry in Philippines. International Journal of Food Microbiology 70:194-206.
Crossref
|
|
|
Membre JM, Burlot PM (1994). Effects of Temperature, pH, and NaCl on Growth and Pectinolytic Activity of Pseudomonas marginalis. Applied and Environmental Microbiology 60(6):2017-2022.
Crossref
|
|
|
Nicole JM, Zhilong G, Xing-fung LI (2004). Reverse Transcription- multiplex PCR Assay for Simultaneous Detection of E. coli O157:H7, Vibrio cholera O1 and Salmonella typhi. Journal of Clinical Chemistry 50:2037-2044.
Crossref
|
|
|
Pal M, Asnake K, Manyazewal A, Selamawit M, Yashodhara D (2016). Microbial quality of Fish and Fish Products. Beverage and Food World 43(2):46-49.
|
|
|
Silliman BR, Newell SY (2003). Fungal farming in a snail. Proceedings of the National Academy of Sciences 100(26):15643-15648.
Crossref
|
|
|
Van Horn, DJ, Garcia JR, Loker ES, Mitchell KR, Mkoji GM, Adema CM, Takacs-Vesbach CD (2012). Complex intestinal communities in three species of planorbid snails. Journal of Molluscan Studies 78(1):74-80.
Crossref
|
|
|
Woo PCY, Lau SKP, Teng JLL, Tse H, Yuen KY (2008). Then and now: use of 16S rDNA gene sequencing for bacterial identification and discovery of novel bacteria in clinical microbiology laboratories. Clinical Microbiology and Infection 14:908-934.
Crossref
|
|
|
Zaika LL, Engel LS, Kim AH, Palumbo SA (1989). Effect of Sodium Chloride, pH and Temperature on Growth of Shigella flexneri. Journal of Food Protection 52(5):356-359.
Crossref
|
|
|
Zajc J, Zalar P, Plemenitas A, Gunde-cimerman N (2012). The Mycobiota of the salterns. Progress in Molecular and Subcellular Biology 53:133-58.
Crossref
|
|